
Effects of ultrasonic treatment on microstructure and mechanical properties of Mg-5Zn-2Er alloy
WANG Zhao-hui, WANG Xu-dong, WANG Qing-feng, DU Wen-bo
School of Materials Science and Engineering, Beijing University of Technology, Beijing 100124, China
Received 25 September 2010; accepted 20 December 2010
Abstract: The effects of ultrasonic treatment on the microstructure and mechanical properties of Mg-5Zn-2Er alloy at room temperature (RT) and high temperature (HT) were investigated. The microstructure and mechanical properties of the samples were studied by OM, SEM and MTS material tester. The results show that the microstructure and mechanical properties are improved after the ultrasonic vibration. The best effects of ultrasonic vibration on microstructure and mechanical properties were obtained with the ultrasonic vibration power of 600 W and time of 100 s. The cavitation and acoustic streaming caused by ultrasonic treatment play a major role in refining the microstructure and increasing mechanical properties of the alloy.
Key words: Mg-Zn-Er alloy; ultrasonic treatment; microstructure; mechanical properties; mechanism
1 Introduction
Magnesium alloys are the lightest structural metallic materials. In recent years, they have been widely used for prime consumer electronics and automotive parts because of their low density, high specific strength and stiffness, attractive mechanical properties, good electromagnetic shielding, good thermal conductivity, excellent machine ability and availability[1-4]. Recently, Mg-Zn-Re (Re=Y, Gd, Er) alloys have attracted much attention because of their good mechanical properties at room temperature (RT) and high temperature (HT)[5-8]. But these alloys are still limited because their mechanical properties are relatively low compared with aluminium alloys.
Ultrasonic treatment is a convenient, clean and effective method for degassing and refining the microstructure of the low-melting metals alloys during the solidification course. The research and industrial application on ultrasonic degassing of light alloys, such as aluminium alloys, were initialed in 1960s[9-11].
Ultrasonic treatment in the molten magnesium alloy would refine the grains, increase homogeneity, reduce segregation and degas in the alloy. The effects of ultrasonic treatment on microstructure and mechanical properties of AZ91 alloy were investigated widely [12-15]. But few works have been done on the Mg-Zn-Re alloys. As mentioned above, the Mg-Zn-Re alloys have great application potential, it is necessary to investigate the effects of ultrasonic treatment on microstructure and mechanical properties of these alloys.
In this work, the ultrasonic vibration was introduced into the molten Mg-Zn-Er alloy; the effects of ultrasound vibration on microstructure and mechanical properties at RT and HT of the alloy were investigated.
2 Experimental
Mg-5Zn-2Er magnesium alloy (5.0% Zn, 2. 0% Er, rest Mg, mass fraction) was used as the experimental materials. The apparatus for the experiment consists of an ultrasonic generator, a resistance furnace and a protection gas system. The ultrasound generated by this ultrasonic generator is 20.0 kHz with the maximum output of 2.0 kW. In this work, the ultrasound was introduced from the top of the magnesium melt with a cylindrical probe made of the tool steel. The temperature of the molten alloy was controlled by the PID temperature controller with an accuracy of ±5 °C.
The clean and preheated pure Mg, pure Zn and Mg-10Er master alloy were used to prepare the Mg-Zn-Er alloy. The Mg-Zn-Er alloy of about 1 kg was melted and hold at 740 °C in the resistance furnace. After the molten alloy was cooled down to 690 °C, the ultrasonic probe was dipped into the melt for about 15 mm with the prescribed output power for a certain time. Because the liquidus of Mg-5Zn-2Er alloy was about 630 °C, the superheat of the melt was about 50 K during the ultrasonic vibration. After the ultrasonic vibration, the alloy was cast into a permanent mold preheated to 200 °C. The whole experiments were carried out with the protection gas of 0.5% SF6 and 99.5% N2 (volume fraction). The contrastive samples without ultrasonic treatment were also prepared with the same procedures. The experiments were composed of two phases. Firstly, the ultrasonic vibration time was fixed at 100 s, the ultrasonic vibration powers were set as 300, 600 and 900 W, respectively. Then the ultrasonic vibration power was set as one fixed value, the ultrasonic vibration time was set as 50, 100 and 150 s, respectively.
Specimens for micrograph observation were etched in acetic picral after being polished. The optical microscopy and SEM were carried out on a Zeiss Axio Imager A2m and a HITACH S3400N, respectively. The average grain size was measured according to the linear intercept method. The tensile specimens with a gage diameter of 5 mm and a gage length of 25 mm were tested on a MTS 810 testing machine with the cross head speed of 1 mm/min at RT and HT (175?C).
3 Results and discussion
3.1 Microstructure
Figure 1 shows the microstructures of the Mg-5Zn-2Er alloy without and with ultrasound vibration powers when the ultrasonic vibration time is 100 s. The microstructure of Mg-5Zn-2Er is composed of α-Mg, I-phase and W-phase[5]. As shown in Fig.1(a), the α-Mg phase is coarse and the average grain size is about 110 μm for the alloy without the ultrasonic vibration. When the ultrasonic vibration time is 100 s, the grains are refined obviously with the ultrasonic vibration power increasing to 600 W. And the grain size of the alloy is about 60 μm when the ultrasonic vibration power is 600 W. However, the microstructure of the alloy becomes coarse when the ultrasonic vibration power increases to 900 W.
Figure 2 shows the microstructures of the alloy with different ultrasonic vibration time when the ultrasonic vibration power is 600 W. When the ultrasonic vibration power is 600 W, the grains of the alloy are refined remarkably as the ultrasonic vibration time increases to 100 s. However, the grains become coarse and the average grain size increases to 80 μm when the ultrasonic treatment time increases to 150 s. Therefore, the best refined microstructure can be obtained with the ultrasonic vibration power of 600 W and ultrasonic vibration time of 100 s, as shown in Fig.2(b).
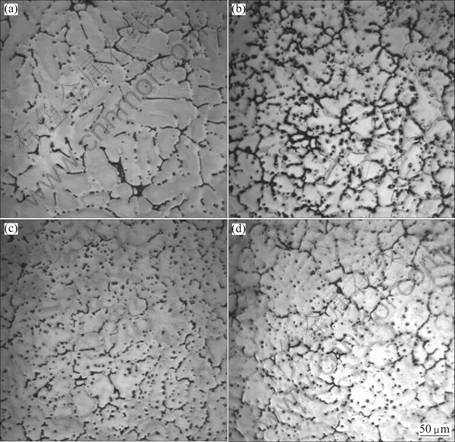
Fig.1 Microstructures of Mg-5Zn-2Er alloy without (a) and with ultrasonic vibration power of 300 W (b), 600 W (c) and 900 W (d)
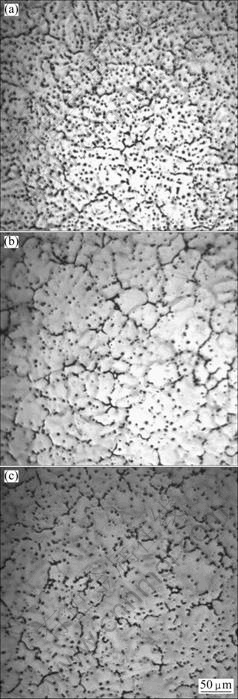
Fig.2 Microstructures of Mg-5Zn-2Er alloys with different ultrasonic vibration time: (a) 50 s; (b) 100 s; (c) 150 s
Figure 3 shows that the SEM micrographs of the Mg-5Zn-2Er alloy without and with ultrasonic vibration. As shown in Fig.3(a), without ultrasonic vibration, most of the intermetallic phases of the alloy locate at the grain boundaries, and these intermetallics are coarse and continuous. After the ultrasonic vibration, the morphologies of these intermetallic phases become discontinuous and fine in comparison with those in the contrastive alloy. Therefore, the mechanical properties of the alloy with ultrasonic vibration could be improved obviously by the combination of the refined α-Mg phase and the intermetallics.
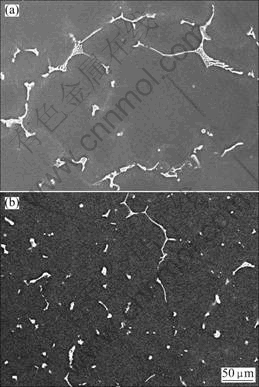
Fig.3 SEM micrographs of Mg-5Zn-2Er alloy: (a) Without ultrasonic vibration; (b) With ultrasonic vibration
The grain refinement of the alloy is due to the heterogeneous nucleation and undercooling induced by the ultrasonic vibration. It was reported that the ultrasonic cavitation in the molten melt could produce transient micro spots at temperature of about 5 000 °C, pressure of above 100 MPa and heating and cooling rates of about 107 K/s[10]. Because of the huge pressure caused by the ultrasonic cavitation, the undercooling and nucleation will occur in the field of ultrasonic cavitation even the superheat of the alloy is 50 K. And because of the high pressure pulses caused by the ultrasonic cavitation, the wettablity of large number of impurity particles, such as Mn or Fe based intermetallic particles, will be improved by eliminating the gas absorbed on the particles and fill the melt in the micro cracks of the particles. Therefore, some of the intermetallic particles become the nuclei during the solidification of the magnesium alloy. And the grain nuclei generated by the two methods will be distributed to the molten alloy by the acoustic streaming induced by the ultrasonic vibration. So the grains of alloy will be refined, as shown in Fig.1 and Fig.2. While the ultrasonic vibration power increases too high, the more generated heat leads to increase the melt temperature in the field of ultrasonic vibration so that some nuclei remelt. Then the microstructure becomes coarser than that of the alloy with lower ultrasound power, as shown in Fig.1.
3.2 Mechanical properties
Figure 4 shows the mechanical properties of the Mg-5Zn-2Er alloy at RT and HT (175 °C) without and with different ultrasound vibration powers when the ultrasonic vibration time is 100 s. Without the ultrasonic vibration, the ultimate tensile strength (UTS) and elongation of the alloy at RT are 151 MPa and 7%, respectively. When the ultrasonic vibration power increases to 600 W, the UTS and elongation are 210 MPa and 11%, respectively. Compared with the untreated alloy, the UTS and elongation are improved by 39% and 57%, respectively. But the UTS and elongation of the alloy decrease slightly while the ultrasonic vibration power increases to 900 W. And the mechanical properties of the alloy at HT are also according to the similar rules.
Figure 5 shows that the mechanical properties at RT and HT of the alloy with different ultrasonic vibration time when the ultrasonic vibration power is 600 W. The UTS and elongation of the alloy at RT and HT increase rapidly when the ultrasonic vibration time increases to 100 s. But the UTS and elongation of the alloy decrease when the ultrasonic vibration time reaches 150 s.
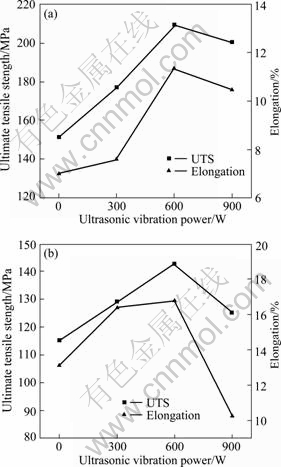
Fig.4 Mechanical properties of Mg-5Zn-2Er alloy with ultrasonic vibration at different temperatures: (a) RT; (b) 175 °C
The fractures of the alloys without and with ultrasonic treatment at RT are shown in Fig.6. As shown in Fig.6(a), the sample without ultrasonic vibration exhibits the brittle batten feature. Comparatively, the fracture of sample with ultrasonic vibration displays vast serried dimples, as shown in Fig.6 (b).
So the optimum ultrasonic vibration process of the Mg-Zn-Er alloy is ultrasonic vibration power of 600 W and ultrasonic vibration time of 100 s in this work. The changes of mechanical properties of the alloy accord with the evolution of microstructure of the alloy with different ultrasonic vibration processes. This indicates that the improvement of mechanical properties of the alloy are mainly caused by the refinement of the grains and intermetallics, which are induced by the cavitation and acoustic flow streaming caused by ultrasonic vibration.
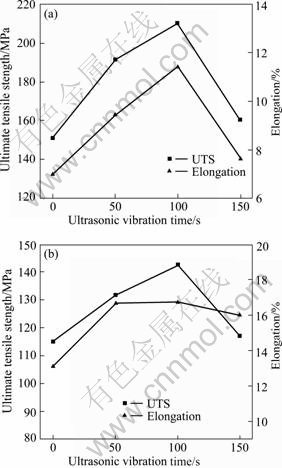
Fig.5 Mechanical properties of Mg-5Zn-2Er alloy with ultrasonic vibration at different temperatures: (a) RT; (b) 175 °C
4 Conclusions
1) The ultrasonic treatment has good effects on the microstructure and mechanical properties of Mg-5Zn-2Er alloy at RT and HT.
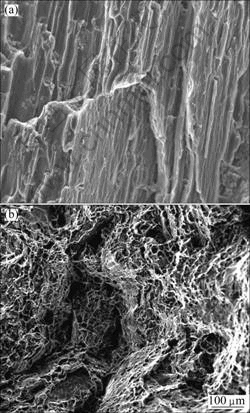
Fig.6 Fracture morphologies of Mg-5Zn-2Er alloy: (a) Without ultrasonic vibration; (b) With ultrasonic vibration
2) The optimum ultrasonic vibration process conditions are ultrasonic vibration power of 600 W and ultrasonic vibration time of 100 s.
3) The grain refinement of the alloy is mainly attributed to the acoustic cavitation and acoustic streaming induced by the ultrasonic vibration.
References
[1] MORDIKE B L, EBERT T. Magnesium: Properties-applications-potential [J]. Materials Science and Engineering A, 2001, 302: 37-45.
[2] FRIENDRICH H, SCHUMANN S. Research for a “new age of magnesium” in the automotive industry [J]. Journal of Materials Processing Technology, 2001, 117: 276-281.
[3] SCHUMANN S. The paths and strategies for increased magnesium applications in vehicles [J]. Materials Science Forum, 2005, 488-489: 1-8.
[4] SHI C X, KE J, KAHN R W. Materials science and technology [M]. Beijing: Science Press, 1999. (in Chinese)
[5] LI J H, DU W B, LI S B, WANG Z H. Tensile and creep behavior of Mg-5Zn-2.5Er alloy improved by icosahedral quasicrystals [J]. Materials Science and Engineering A, 2009, 527: 1255-1259.
[6] WANG J G, HSIUNG L M, NIEH T G, MABUCHI M. Creep of a heat treated Mg-4Y-3RE alloy [J]. Materials Science and Engineering A, 2001, 315 (1-2): 81-88.
[7] ROKHLIN L L, DOBATKINA T V, TARYTINA I E, TIMOFEEV V N, BALAKHCHI E E. Peculiarities of the phase relations in Mg-rich alloys of the Mg-Nd-Y system [J]. Journal of Alloys and Compounds, 2004, 367(1-2): 17-19.
[8] GAO Y, WANG Q D, GU J H, ZHAO Y, TONG Y. Effects of heat treatments on microstructure and mechanical properties of Mg-15Gd-5Y-0.5Zr alloy [J]. Journal of Rare Earths, 2008, 26(2): 298-302.
[9] ESKIN G I. Ultrasonic treatment of light alloy melts [M]. Amsterdam: Gordon & Breach, 1998.
[10] ESKIN G I. Cavitation mechanism of ultrasonic melt degassing [J]. Ultrasonics Sonochemistry, 1995, 2(2): s137-s141.
[11] ESKIN G I. Broad prospects for commercial application of the ultrasonic (cavitation) melt treatment of light alloys [J]. Ultrasonics Sonochemistry, 2001, 8(3): 319-325.
[12] LIU X B, OSAWA Y, TAKAMORI S, MUKAI T. Microstructure and mechanical properties of AZ91 alloy produced with ultrasonic vibration [J]. Materials Science and Engineering A, 2008, 487(1-2): 120-123.
[13] ZHANG Z Q, LE Q C, CUI J Z. Ultrasonic treatment of magnesium alloy melts and its effects on solidification microstructures [J]. Materials Science Forum, 2007, 546-549: 129-132.
[14] XU H B, JIAN X G, MEEK T T, HAN Q Y. Degassing of molten aluminum A356 alloy using ultrasonic vibration [J]. Materials Letters, 2004, 58 (29): 3669-3673.
[15] LI J W, MOMONO T, TAYU Y, FU Y. Application of ultrasonic treating to degassing of metal ingots [J]. Materials Letters, 2008, 62(25): 4152-4154.
超声处理对Mg-5Zn-2Er合金组织及力学性能的影响
王朝辉, 王旭东, 王庆峰, 杜文博
北京工业大学 材料科学与工程学院,北京 100124
摘 要:研究超声处理对Mg-5Zn-2Er镁合金显微组织及室温、高温力学性能的影响规律。利用光学显微镜、扫描电镜和MTS材料试验机等研究不同样品的显微组织及其室温、高温力学性能。结果表明:超声处理后镁合金的组织和力学性能均得到了改善;获得最优镁合金材料组织和性能时超声处理的工艺为:超声处理功率600 W,超声处理时间100 s。超声处理在熔体中引起的空化和声流效应对细化镁合金的组织并提高其力学性能起到了主要作用。
关键词:Mg-Zn-Er合金;超声处理;显微组织;力学性能;机理
(Edited by LI Xiang-qun)
Foundation item: Projects (PHR200906101, 00900054R7001, JC009011201301) supported by Beijing Municipal Education Commission, China; Project (X1009011201002) supported by Beijing University of Technology Science Foundation for Youths, China
Corresponding author: WANG Zhao-hui; Tel: +86-10-67392423; E-mail: wangzhaohui@bjut.edu.cn
DOI: 10.1016/S1003-6326(11)60779-6