J. Cent. South Univ. Technol. (2010) 17: 239-243
DOI: 10.1007/s11771-010-0037-z 
Coexistence of antiferromagnetic and ferromagnetic in Mn-doped anatase TiO2 nanowires
LI Hong-mei(李红梅)1, 2, LIU Min(刘敏)1, 2, ZENG Yang-su(曾阳素)1, 2, HUANG Tong-cheng(黄同成)1, 2
1. Department of Information Engineering, Shaoyang University, Shaoyang 422000, China;
2. Institute of Laser and Information Technology, Shaoyang University, Shaoyang 422000, China
? Central South University Press and Springer-Verlag Berlin Heidelberg 2010
Abstract: A series of Mn-doped TiO2 nanowires (NWs) were prepared by hydrothermal method at the mole fraction of Mn changing from 0 to 12.0%. X-ray powder diffraction (XRD) analysis shows that all the samples have pure anatase structure. SEM and TEM studies show that the diameter and the length of the Mn-doped TiO2 NWs are larger than those of the undoped TiO2 NWs. Energy dispersive X-ray spectroscopy (EDX) reveals that the samples are composed of Ti, Mn and O. According to magnetization measurements, all samples show ferromagnetic behavior, but only the undoped TiO2 NWs are completely ferromagnetic with a saturated magnetization about 1.0 mA?m2/kg. Mn-doped TiO2 samples exhibit antiferromagnetic and ferromagnetic (AF-FM) behaviors simultaneously. Photoluminescence (PL) spectra demonstrate the existence of MnO2 sublattice. These observations indicate that an AF-WF crossover is induced by the coexistence of TiO2 sublattice and MnO2 sublattice.
Key words: TiO2; nanowires; Mn; antiferromagnetism; ferromagnetism
1 Introduction
A variety of doped-semiconducting materials possess two properties: semiconductor nature and ferromagnetic behavior, which are called diluted magnetic semiconductors (DMS). DMS materials have attracted much attention in recent years for their potential spintronics applications. Since Co-doped anatase TiO2 thin film with room temperature (RT) ferromagnetism (FM) was found by MATSUMOTO et al [1], TiO2-based DMS materials have been studied vigorously over recent years. However, the origin of magnetic moment, the coupling mechanism, and the precise state of the impurities remain unknown.
The fully stoichiometrical TiO2 is a non-magnetic semiconductor, with a band gap of about 3.2 eV spanned by O 2p and Ti 3d states (for anatase structure) [2]. Theoretically, transition metal doped TiO2 can be transformed into DMS. Many research groups have focused their work on doping TiO2 with Co, Cu, and Fe [3-5]. However, there are a few reports on Mn-doped TiO2. Great difference also exists in the results. Theoretical calculation revealed that Ti1-xMnxO2 was antiferro-magnetic (AF) [6], while RT FM was found in Mn-doped TiO2 thin films by WANG et al [7]. They thought that Mn strengthened RT FM of TiO2. In contrast, HONG et al [8] revealed that Mn did not play any key role in inducing the FM of TiO2 and Mn, even decreased the FM of TiO2 when its content reached some values.
In this work, the structure and the magnetic properties of Mn-doped TiO2 NWs prepared by a simple and low temperature hydrothermal method were investigated, and a special phenomenon of AF-WF coexistence in Mn-doped TiO2 NWs was analyzed.
2 Experimental
TiO2 NWs were synthesized by a typical hydrothermal method. All chemicals were used as received without further purification. Commercial TiO2 powders (mixed with anatase structure and rutile structure), sodium hydroxide (NaOH), ethanol (C2H5OH) and potassium permanganate (KMnO4) were all analytical reagents (AR). First, 10 mol/L NaOH solution was attained by solving NaOH in the mixed solution of deionized water and ethanol; the volume ratio of deionized water to ethanol was 1:1. 30 mL of this solution was mixed with 1 g TiO2 powder and ultrasonic- vibrated for 5 min in order to make the solution uniform. Then, different contents of KMnO4 were mixed with the solvent. The mole fraction of Mn was changed from 0 to 12%. The mixed solution was ultrasonic-vibrated for another 5 min and transferred into a teflon-lined stainless steel autoclave with 50 mL capacity. The autoclave was maintained at 150 ℃ under autogenous pressure for 15 h, and then cooled to room temperature.
The products were collected by centrifugation and thoroughly washed with 0.1 mol/L HNO3 aqueous solution, and then washed with deionized water until the pH value of the washing solution was about 7.0, finally, dried at 60 ℃ for several hours in air.
The products were characterized by XRD using a Philips X’Pert MRD X-ray diffractometer with Cu Kα radiation (λ=0.154 056 nm). The images of SEM were recorded on an FEI QUANTA 200F analyzer. The images of TEM were recorded on a Hitachi H-9000 NAR microscope. Photoluminescence (PL) spectra were obtained using a Hitachi F-4500 fluorophotometer with the excited wavelength of 325 nm at room temperature. Magnetic properties were measured using an alternative gradient magnetometer.
3 Results and discussion
Fig.1 shows XRD patterns of the raw TiO2 powders and the TiO2 NWs. It can be seen that anatase and rutile phases coexist in the raw TiO2 powders. However, the obtained TiO2 NWs show typical anatase structure (JCPDS No. 21-1272), after hydrothermal treatment. No metallic Mn precipitation was detected in XRD. As we know, KMnO4 is a strong oxidizer, which can react with ethanol or water to form Mn4+ ions. The ionic radius and coordination number of Mn4+ are close to those of Ti4+. Therefore, the lattice of TiO2 did not change significantly. This is consistent with the previous published data for Mn-doped TiO2 thin films [8].
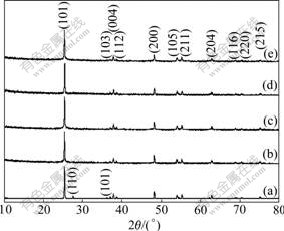
Fig.1 XRD patterns of raw TiO2 powders (a), and 0% (b), 2.4% (c), 7.2% (d) and 12.0% (e) Mn-doped TiO2 NWs
Fig.2 shows the SEM images of TiO2 NWs. It can be seen that the undoped TiO2 NWs are straight and uniform with smooth surface (Fig.2(a)). The diameter of the NWs is about 50 nm and the length of NWs is less than 1 μm. A few nanoparticles do not change to NWs because a low temperature, 150 ℃, is used. However, Mn-doped TiO2 NWs exhibit different results. Figs.2(b)- (d) reveal that Mn-doped TiO2 NWs are no longer straight and have rough surfaces. The curvature and the roughness of Mn-doped samples increase with the increase of the mole fraction of Mn. Both their diameter and length are larger than those of the undoped TiO2 NWs, reaching several hundred nanometers and several micrometers or several tens micrometers, respectively. These indicate that Mn4+ can assist the growth of TiO2 NWs. The possible speculation is that Mn4+ can help TiO2 NWs to release the surface tension and reduce the free energy. These are beneficial to the growth of TiO2 NWs. On the other hand, Mn-doped TiO2 NWs cannot keep straight any more and have some rough materials on the surface. So, with the increase of KMnO4 content, corresponding to the increase of Mn ions doped into TiO2 NWs, the surfaces of TiO2 NWs become rougher.
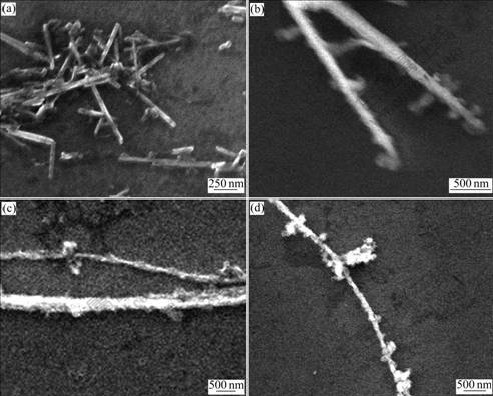
Fig.2 SEM images of TiO2 NWs doped with different mole fractions of Mn: (a) 0%; (b) 2.4%; (c) 7.2%; (d) 12.0%
Fig.3 shows the EDX spectrum of 2.4% Mn-doped TiO2 NWs. It reveals that the sample is composed of Mn, Ti and O, which demonstrates that Mn is doped in TiO2 NWs. Fig.4 shows the TEM images of Mn-doped TiO2 NWs, which present rough surfaces more clearly and coincide with the SEM images.
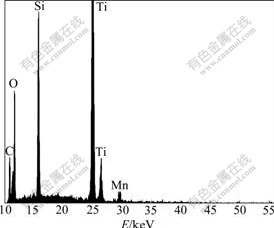
Fig.3 EDX result of 2.4% Mn-doped TiO2 NWs
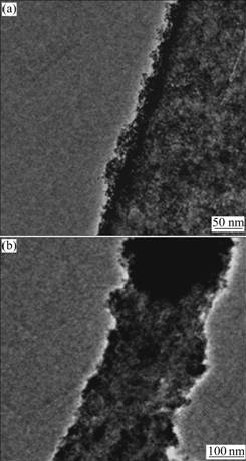
Fig.4 TEM images of 2.4% (a) and 12.0% (b) Mn-doped TiO2 NWs
Fig.5 presents the magnetic hysteresis (M-H) curves of the samples measured at RT. As shown in Fig.5, all the samples have clear magnetic hysteresis loops. However, only undoped TiO2 sample shows clear RT FM. And its saturated magnetization is about 1.0 mA?m2/kg. Mn-doped samples exhibit AF-FM behaviors simultaneously. Their magnetizations are unsaturated even in 1.6 MA/m field (not shown). Similar behavior was observed in Cu-doped TiO2 and Fe-doped TiO2 [4-5], but never observed in Mn-doped TiO2 samples so far. Noncollinear (canted) spin arrangements in two sublattices were used to understand this phenomenon [9]. In canted spin systems, the AF order is along one axis and the FM order is along another axis. It is proved that MnO2 has AF [10]. Furthermore, KAMINSKI and DAS- SARMA [11] found that TiO2 with oxygen defects (Vo) has RT FM. In our Mn-doped TiO2 samples, the possible speculation is that FM arises from TiO2 lattice with Vo and AF arises from MnO2 lattice. Fig.6 shows that with the increase of mole fraction of Mn, the coercivities (Hc) of samples decrease. This indicates that FM decreases with the increase of mole fraction of Mn. As initial mole fraction of Mn increases from zero, remnant magnetization (RM) of the system gradually rises to a maximum at around 7% Mn, then decreases rapidly, and finally, this AF-WF coexistence phenomenon almost disappears at RM around at 12% Mn (Fig.7).
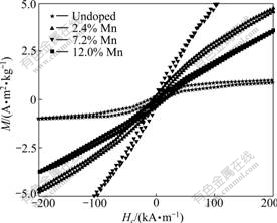
Fig.5 M-H curves of Mn-doped TiO2 NWs at 300 K
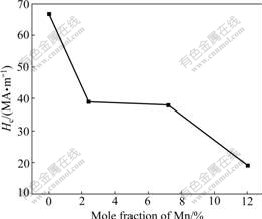
Fig.6 Coercivities of samples at RT vs mole fraction of Mn
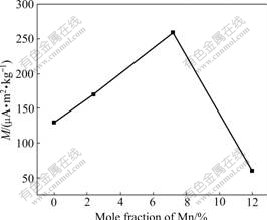
Fig.7 RM of samples vs mole fraction of Mn at RT
In order to investigate the defects in the samples, PL measurements were employed. As shown in Fig.8, the maximum peaks at 3.39, 3.38 and 3.36 eV samples with Mn mole fractions of 2.4%, 7.2% and 12.0%, respectively. These peaks can be attributed to band-to- band recombination whose values should be equal to the values of Eg [12]. However, the values of these peaks are larger than 3.2 eV, the regular Eg of anatase TiO2. This may be related to band gap broaden caused by quantum confinement effect when the diameter is reduced to nano-scale. However, band gap broaden is not just caused by quantum confinement effect. Someone thought that lattice distortions are also contributed to the change of band gap. WUNDERLICH et al [13] believed that distortion of the lattice made Eg higher, and with the increase of distortion, Eg decreases again. In our samples, a possible explanation of the higher Eg can be given if we consider a distortion of TiO2 lattice by doping Mn. Because of doping Mn, many MnO2 lattices are formed, which bring distortions to TiO2 lattice. At the beginning, these distortions lead to an increase of Eg. With the increase of mole fraction of Mn, the degree of disorder in TiO2 lattices increases and Eg decreases finally. The peaks at 3.13, 2.74 and 2.65 eV appear in all Mn-doped TiO2 NWs. The emission peak at 3.13 eV results from electronic transitions between MnO2 and TiO2 nanointerfaces. This is similar to the PL spectra observed by PALOMINO-MERINO et al [14]. It can be seen that the intensity ratio of 3.13 eV to the maximum peak increases with the increase of the mole fraction of Mn. So we can infer that there are more electrons transferring to MnO2 with the increase of mole fraction of Mn, which indicates that the number of MnO2 lattice increases with the increase of mole fraction of Mn. Two peaks at 2.74 and 2.65 eV existing in all samples are attributed to oxygen defects of TiO2 [15]. The interval of the two peaks is 0.09 eV. So, in this case, we attribute 2.74 eV to Ti4+ adjacent to oxygen vacancies (Vos) and 2.65 eV to Vos [14].
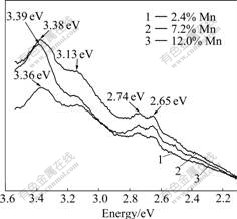
Fig.8 PL spectra of 2.4%, 7.2%, 12.0% Mn-doped TiO2 NWs
Theoretical calculation reveals that Ti1-xMnxO2 is AF [6]. However, FM was found in Mn-doped TiO2 thin films [7-8]. Different from thin films usually prepared at high temperature or with a high temperature post treatment, in this work, TiO2 NWs were prepared by a low temperature hydrothermal method. So many defects exist in TiO2 NWs, such as undercoordinated surface Ti sites and Vos. Vos are considered to be the origin of RT FM of TiO2. The PL peaks at 2.74 and 2.65 eV confirm the existence of Vos. The PL peak at 3.13 eV, which results from electronic transitions between intraband shallow levels at the nanointerfaces between MnO2 and TiO2, proves the existence of MnO2 sublattices [14]. As demonstrated before, TiO2 with Vos has FM and MnO2 has AF [10-11]. So, the reason for AF-FM in Mn-doped TiO2 NWs is the coexistence of TiO2 lattice and MnO2 lattice. With the increase of mole fraction of Mn, Eg of Mn-doped TiO2 NWs increases firstly and decreases finally, and the intensity ratio of 3.13 eV to the maximum peak increases. These indicate that the number of MnO2 lattice increases with increasing mole fraction of Mn. Furthermore, Hc and RM of the Mn-doped samples decrease with the increase of mole fraction of Mn and completely show AF at around 12% Mn. The possible reason is that Vos of TiO2 lattice decrease because MnO2 lattice increases or more Mn substitutes Ti in TiO2 with the increase of mole fraction of Mn. So, with the increase of mole fraction of Mn, both Hc and RM decrease and show AF at RT finally. It is also found that Mn does not have any contribution to FM of TiO2 NWs. In contrast, Mn ions have destructive effect on FM of TiO2.
4 Conclusions
(1) Mn-doped TiO2 nanorods are prepared by a simple and low temperature hydrothermal method. All of the samples are of anatase structure. The diameter and the length of the Mn-doped NWs are larger than those of the undoped TiO2 NWs.
(2) The magnetic property of Mn-doped TiO2 NWs is different from that of undoped TiO2 NWs with FM and has AF-WF at RT.
(3) Further studies show that, FM arises from TiO2 lattices with Vos and AF arises from MnO2 lattices. Due to canted spin arrangements in these two sublattices, AF and FM coexist in Mn-doped TiO2 NWs simultaneously. FM at RT decreases with the increase of mole fraction of Mn, and Mn-doped samples show complete AF finally.
(4) Mn ions do not contribute to the FM of TiO2, instead, they have destructive effect on FM of TiO2.
References
[1] MATSUMOTO Y, MURAKAMI M, SHONO T, HASEGAWA T, FUKUMURA T. Room-temperature ferromagnetism in transparent transition metal-doped titanium dioxide [J]. Science, 2001, 291(5505): 854-856.
[2] ASAHI R, TAGA Y, MANNSTADT W, FREEMAN A J. Electronic and optical properties of anatase TiO2 [J]. Physical Review B, 2000, 61(11): 7459-7465.
[3] WANG Z J, TANG J K, CHEN Y X, LEONARD S, ZHOU W L, TUNG L D. Room-temperature ferromagnetism in manganese doped reduced rutile titanium dioxide thin films [J]. Journal of Applied Physics, 2004, 95(11): 7384-7386.
[4] TORRES C E R, GOLMAR F, CABRERA A F, ERRICO L, NAVARRO M, RENTERIA A M M, SANCHEZ F H, DUHALDE S. Magnetic and structural study of Cu-doped TiO2 thin films [J]. Applied Surface Science, 2007, 254(1): 365-367.
[5] YAMAURA K, WANG X H, LI J G, ISHIGAKI T, TAKAYAMA-MUROMACHI E. Magnetic properties of the highly iron-doped rutile TiO2 nano crystals [J]. Materials Research Bulletin, 2006, 41(11): 2080-2087.
[6] ERRICO L A, WEISSMANN M, RENTERIA M. Ab initio study of the magnetic ordering in the semiconductors MnxTi1-xO2, CoxTi1-xO2 and FexTi1-xO2 [J]. Physica B: Condensed Matter, 2004, 354(1/4): 338-340.
[7] WANG Z J, WANG W D, TANG J K, LUAG L D, LEONARD S, ZHOU W L. Extraordinary Hall effect and ferromagnetism in Fe-doped reduced rutile [J]. Applied Physics Letters, 2003, 83(3): 518-520.
[8] HONG N H, SAKAI J, RUYTER A, BRIZE V. Does Mn doping play any key role in tailoring the ferromagnetic ordering of TiO2 thin films? [J]. Applied Physics Letters, 2006, 89(25): 252504.
[9] KUNDYS B, MAIGNAN A, MARTIN C, NGUYEN N, SIMON C. Magnetic field induced ferroelectric loop in Bi0.75Sr0.25FeO3-δ [J]. Applied Physics Letters, 2008, 92(11): 112905.
[10] DORMANN J L, NOGUES M. Magnetic structures in substituted ferrites [J]. Journal of Physics: Condensed Matter, 1990, 2(5): 1223-1237.
[11] KAMINSKI A, DAS-SARMA S. Polaron percolation in diluted magnetic semiconductors [J]. Physics Review Letters, 2002, 88(24): 247202.
[12] LIU Bao-shun, WEN Li-ping, ZHAO Xiu-jian. The photoluminescence spectroscopic study of anatase TiO2 prepared by magnetron sputtering [J]. Materials Chemistry and Physics, 2007, 106(2/3): 350-353.
[13] WUNDERLICH W, MIAO L, TANEMURA M, JIN P, KANEKO K, TERAI A, NABATOVA-CABIN N, BELKADA R. Ab-initio calculations of the optical band gap of TiO2 thin films [J]. International Journal of Nanoscience, 2004, 3(4/5): 439-445.
[14] PALOMINO M R, TORRES K J, LOZADA M R, PORTILLO M O, GARCIA R M, ZELAYA A O. Photoluminescence of Rhodamine 6G-doped amorphous TiO2 thin films grown by sol-gel [J]. Vacuum, 2007, 81(11/12): 1480-1483.
[15] LEI Y, ZHANG L D, MENG G W, LI G H, ZHANG X Y, LIANG C H, CHEN W, WANG S X. Preparation and photoluminescence of highly ordered TiO2 nanowire arrays [J]. Applied Physics Letters, 2001, 78(8): 1125-1127.
Foundation item: Project(06JJ2031) supported by Natrual Science Foundation of Hunan Province, China; Project(06A065) supported by Education Department of Hunan Province, China
Received date: 2009-03-10; Accepted date: 2009-06-02
Corresponding author: ZENG Yang-su, PhD, Professor; Tel: +86-739-5305947; E-mail: zengyangsu@126.com
(Edited by CHEN Wei-ping)