J. Cent. South Univ. Technol. (2009) 16: 0091-0095
DOI: 10.1007/s11771-009-0015-5
Preparation and electrochemical characterization of activated carbons by chemical-physical activation
ZHANG Zhi-an(张治安), CUI Mu(崔 沐), LAI Yan-qing(赖延清),LI Jie(李 劼), LIU Ye-xiang(刘业翔)
(School of Metallurgical Science and Engineering, Central South University, Changsha 410083, China)
Abstract: A process was proposed based on the combination of chemical and physical activation for the production of activated carbons used as the electrode material for electric double layer capacitor (EDLC). By material characterization and electrochemical methods, the influences of the activitation process on the specific surface area, pore structure and electrochemical properties of the activated carbons were investigated. The results show that specific surface area, the mesopore volume, and the specific capacitance increase with the increase of the mass ratio of KOH to char (m(KOH)/m(char)) and the activation time, respectively. When m(KOH)/m(char) is 4.0, the specific surface area and the mesopore volume reach the maximum values, i.e. 1 960 m2/g and 0.308 4 cm3/g, and the specific capacitance is 120.7 F/g synchronously. Compared with the chemical activation, the activated carbons prepared by chemical-physical activation show a larger mesopore volume, a higher ratio of mesopore and a larger specific capacitance.
Key words: activated carbon; electric double layer capacitor; chemical-physical activation; pore structure
1 Introduction
More attention has been paid to the research on the electric double layer capacitor (EDLC) for high-power devices in energy-storage systems in recent years. EDLCs are now utilized in many fields, such as space industry, national defense, war industry, electrical vehicle, wireless communication, and consume electronics [1-5]. EDLCs store energy by the quick formation of a double layer at the interface between a high surface area electrode and an electrolyte solution. On the basis of the mechanism for double-layer formation, the larger the specific surface area of the electrode is, the more the energy can be stored. For this reason, activated carbons (ACs) with high surface area are widely used as EDLC electrode materials. However, their essential microporous and disordered pore structures hinder the performance because the narrow pores are not easily accessible to electrolytes and the motion of ions in such small pores is very slow. These drawbacks have important implications for the practical use of EDLCs electrodes since the charge storage is greatly limited and high rate capability cannot be achieved [6-7]. Therefore, a lot of researches were focused on the development of mesopores of ACs in order to optimize their capacitive performances.
The physical properties of ACs have been found to strongly depend on the activation process and the nature of raw materials. In general, the activation for the preparation of ACs is generally classified as physical and chemical methods. Physical activation (by steam or CO2) was found to significantly develop pores in a wide range diameters. Chemical activation (by KOH, NaOH or H3PO4), on the other hand, is used to form micropores. Thus, a combination of chemical and physical activation method is a potential technique in preparing ACs for EDLCs.
The result in Ref.[8] show that the activated carbon prepared by chemical activation methods using pitch as raw carbon source has a high specific surface with a low mesopore ratio. However, the activated carbon prepared by physical activation shows a high mesopore ratio, but a low specific surface area [9-11]. So combining the two processes helps to improve the pore structure and the specific surface area of ACs [12-14].
The aim of this work is to prepare activated carbons from pitch through the combination of KOH activation and CO2 gasification. The physical properties such as the BET surface area, pore size distribution, total pore volume, micropore volume, mesopore ratio were studied systematically. In addition, their electrochemical properties were evaluated in the nonaqueous electrolyte.
2 Experimental
2.1 Preparation of activated carbons
Coal tar-pitch was placed in a nickel crucible, with a heating rate of 3 ℃/min from room temperature to 500 ℃ and holding 5 h at this temperature, in a flow of argon of 60 mL/min. Under such as oxygen-deficient condition, the easily emitted compound with a small molecule was removed and the mesophase pitches were obtained. This is the carbonization process.
After the carbonization step, the mesophase pitches were crushed, and sieved to a uniform size ranging from 100 to 200 μm. These powders were then mixed with KOH in a stainless steel beaker, with m(KOH)/m(char) of 0.5, 1.0, 2.0, 3.0 and 4.0, respectively. The mixtures were heated at a rate of 5 ℃/min to 800 ℃, and kept at this temperature for 2 h. In the meantime, Ar gas was flowed into the oven at a rate of 60 mL/min. Then argon gas was shut off and CO2 gas was immediately introduced into the oven at a rate of 60 mL/min. The products were cooled to room temperature, poured to a beaker containing HCl, and then washed with deionized water until pH of the washing solution reaches 6-7. After rinsing process, the activated carbons were dried in an oven at 110 ℃ for 10 h. The samples were classified according to m(KOH)/m(char) and the time duration of CO2 gasification, such as AC3020 (30: m(KOH)/m(char) of 3.0; 20: the CO2 gasification time of 2 h).
The BET surface area and pore structure of various activated carbons were measured by the N2 adsorption isotherms at 77 K with a sorptiometer (Autosorb-6). The mesopores and micropores distribution were determined by the BJH and HK methods, respectively.
2.2 Electrochemical measurement
Activated carbon powders were well mixed with 10% (mass fraction) of carbon black as conductor, and 10% (mass fraction) of PVDF as a binder, which were dissolved in N-methyl-2-pyrrolidone (NMP) to obtain a slurry. This slurry was laminated on an aluminum foil, and dried in a vacuum oven at 100 ℃. The roller was then used to roll the electrode film until its thickness was less than 0.19 mm. The capacitor cell was constructed using a couple of electrodes face to face, with a separator inserted between these electrodes. 1 mol/L Et4NBF4 in propylene carbonate (PC) solution was used as the electrolyte. The specific capacitance was calculated from the corresponding voltage variation of the discharge.
3 Results and discussion
3.1 Influence of m(KOH)/m(char) on physical and electrochemical properties of activated carbons
In order to gain an understanding on the influences of activation methods on the structure of activated carbons, the pore structures of various carbons were determined. Fig.1 shows the typical N2 adsorption/ desorption isotherms for activated carbons, prepared at different m(KOH)/m(char) values. Samples AC0520 and AC1020, the m(KOH)/m(char) values of which are not more than 1, have a very little adsorbed volume, and the adsorbed volume of N2 is increased with the increase of the relative pressure, indicating highly mesoporous materials with a low specific surface area. With increasing m(KOH)/m(char) from 2 to 4, the N2 adsorption for samples AC2020, AC3020 and AC4020, is larger than that of the former samples at low, middle, or high relative pressures, and a plateau appears with increasing relative pressures. Therefore, the curves are shaped as a combination of TypeⅠand Type Ⅱ. This suggests that activated carbons prepared at m(KOH)/ m(char) from 2 to 4 have well-developed microporosity as well as mesoporosity.
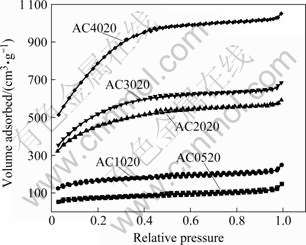
Fig.1 N2 adsorption/desorption isotherms of activated carbons prepared in m(KOH)/m(char) range from 0.5 to 4.0
According to the definition from IUPAC, pores are classified into three groups based on the pore diameter: micropores (<2 nm), mesopores (2-50 nm), and macropores (>50 nm) [15]. The pore size distribution of all activated carbons examined in Fig.1 is shown in Fig.2. The pores distributed within these activated carbons are mainly composed of two groups: micropores and mesopores with the pore diameter in the range of 2-4 nm. In addition, the amount of micropores is gradually increased and the mesopores are steadily developed with increasing m(KOH)/m(char).
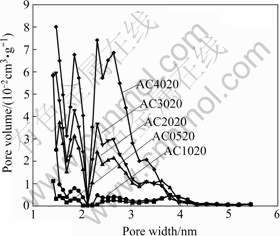
Fig.2 Pore size distributions of activated carbons prepared in m(KOH)/m(char) range from 0.5 to 4.0
The pore properties of the activated carbons prepared at different m(KOH)/m(char) values are listed in Table 1. Increasing m(KOH)/m(char) leads to an increase in the BET surface area, the total pore volume, and the mesopore volume. When m(KOH)/m(char) is 4, the BET surface area reaches 2 960 m2/g; the total pore volume is increased to 1.624 0 cm3/g; and the mesopore volume achieves 0.308 4 cm3/g. The results indicate the continuous development of pores in various diameters. This is quite agreement with Fig.1.
Table 1 Physical properties of activated carbons prepared at different m(KOH)/m(char) values
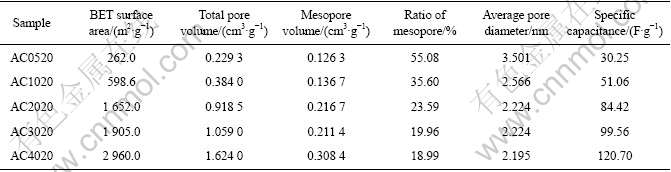
The variation of the ratio of mesopore and average pore diameter with m(KOH)/m(char) is interesting. With increasing m(KOH)/m(char), the ratio of mesopore and the average pore diameter of ACs decrease. This implies that a large proportion of micropore is formed within activated carbons prepared at high m(KOH)/m(char). Moreover, the complete coverage of K2CO3, which is produced in the reaction of KOH with pitch, prevents micropores from being burnt by the attack of CO2 [16]. So the increase of specific surface results from the development of micropores.
It is also found from Table 1 that the specific capacitance is increased from 30.25 to 120.70 F/g, with increasing m(KOH)/m(char) from 0.5 to 4.0. This result may be due to the development of high specific surface and mesopore volume of activated carbons prepared at high m(KOH)/m(char).
3.2 Influence of activation time on physical and electrochemical properties of activated carbons
Fig.3 shows the typical N2 adsorption/desorption isoterms for all activated carbons prepared at different CO2 gasification times (tG). The adsorbed volume of N2 increases with increasing tG. The slope of the corresponding isotherms also increases with increasing tG. These results indicate that the size distribution of pores becomes wider. Moreover, the adsorbed volume also increases with prolonging the activation time.
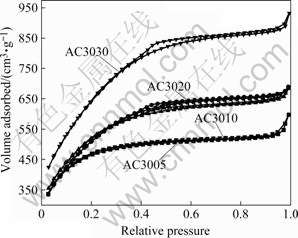
Fig.3 Adsorption/desorption isotherms of N2 at 77 K on activated carbons prepared at different activation time
The pore size distribution of activated carbons prepared for different activation times is shown in Fig.4. The mesopore volume is gradually increased with prolonging the activation time. Moreover, the size distribution of pores is gradually widened. These results suggest that the development of micropores and an increase of average diameter of pores may occur simultaneously.
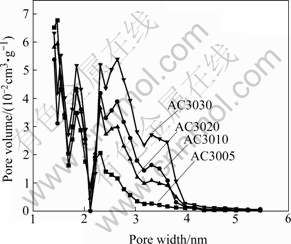
Fig.4 Pore size distribution of activated carbons prepared for different activation time
The pore properties, such as the BET surface area, the total pore volume, the mesopore volume, and the average pore diameter are shown in Table 2. Note that in Table 2 the BET surface area and the mesopore volume increase with prolonging activation time, indicating the gradual transformation of micropores to mesopores. Meanwhile, prolonging activation time renders gradual increase of average pore diameter. In addition, the specific capacitance is found to increase from 77.43 to 115.36 F/g with the increase of the CO2 gasification time. This is attributed to the increase of the BET surface area and ratio of mesopore.
Table 2 Pore properties of activated carbons prepared for different activation time
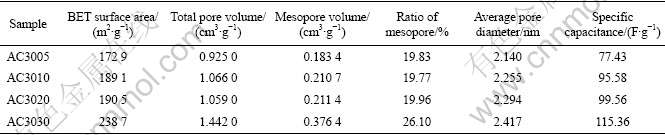
3.3 Comparison of structure and electrochemical properties of activated carbons prepared by different activation method
The pore structure of activated carbons prepared by chemical activation and chemical-physical activation is compared to gain an understanding on the influences of chemical-physical activation methods on the pore structure of activated carbons. Coal tar-pitch and KOH were mixed in nickel crucible, and the range of m(KOH)/ m(char) is from 1 to 4. Every sample was heated at 800 ℃ for 2 h in argon atmosphere. The heating rate is 5 ℃/min. Then the activated carbon was washed and dried as the chemical-physical activation. The activated carbons by chemical activation were obtained, which were denoted as AC1-800, AC2-800, AC3-800, AC4-800 (4: m(KOH)/m(char) of 4.0; 800: heating temperature of 800 ℃), respectively.
The physical properties of activated carbons prepared by chemical and chemical-physical activation are shown in Table 3. Compared with those obtained by chemical activation, the activated carbons prepared by chemical-physical activation have no obvious differences in the specific surface area, but the mesopore volume and ratio of mesopore increase a lot. Note that for AC4-800 and AC4020, the BET surface areas are 2 984 and 2 960 m2/g; the total pore volumes are 1.581 and 1.624 cm3/g; the mesopore volumes are 0.249 3 and 0.308 4 cm3/g; the ratio of mesopore is 15.77% and 18.99%, respectively. These results are probably because of the more powerful etching rate of chemical-physical activation, and the transformation from micropores to mesopores, due to the presence of CO2.
Table 3 Physical properties of activated carbons prepared by chemical and chemical-physical activation
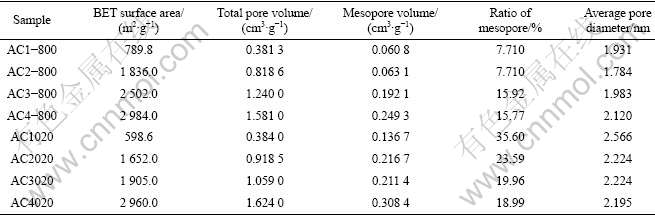
Figs.5(a) and (b) show the dependence of mass specific capacitance and area specific capacitance on m(KOH)/m(char), respectively. The mass specific capacitance of chemical-physical activated carbons is rapidly increased, while a slow rate in developing specific capacitance is found for chemical activated carbons, when m(KOH)/m(char) is gradually increased. On the other hand, the area specific capacitance of chemical-physical activated carbons is larger than that of chemical activated carbons, when m(KOH)/m(char) values for these carbons are the same. Based on the results and discussion of Fig.5, a combination of KOH activation and CO2 gasification is a powerful method in preparation of activated carbon used in EDLC.
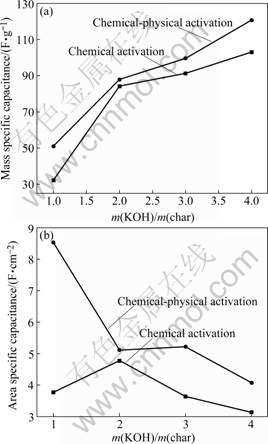
Fig.5 Dependence of mass specific capacitance (a) and area specific capacitance (b) on m(KOH)/m(char)
4 Conclusions
(1) Using KOH and CO2 as agents, the activated carbons are obtained by chemical-physical activation method. The activated carbon material has a high surface area and a high mesopore volume.
(2) With the increase of m(KOH)/m(char), the specific surface area, the total pore volume, and the mesopore volume increase. At m(KOH)/m(char) of 4, the BET surface area reaches 2 960 m2/g and the mesopore volume achieves 0.308 4 cm3/g. The specific capacitance increases with the increase of BET surface area and the mesopore volume. The maximum specific capacitance is 120.7 F/g when m(KOH)/m(char) is 4.
(3) With increasing CO2 gasfication time, the specific surface area, the total pore volume and the mesopore volume increase. AC3030 shows the largest BET surface area of 2 387 m2/g, the largest mesopore volume of 0.376 4 cm3/g, and the maximum specific capacitance of 115.36 F/g.
(4) Compared with the chemical activation method, the chemical-physical activation is a powerful method to prepare the activated carbons with a larger mesopore volume and a high mesopore ratio, and a larger specific capacitance.
References
[1] GAMBY J, TABERNA P L, SIMON P, FAUVARQUE J F. CHESNEAU M. Studies and characterizations of various activated carbons used for carbon/carbon supercapacitors [J]. Journal of Power Sources, 2001, 101(1): 109-116.
[2] RUIZ V, BLANCO C, GRANDA M, SANTAMARIA R. Enhanced life-cycle supercapacitors by thermal treatment of mesophase-derived activated carbons [J]. Electrochimica Acta, 2008, 54(2): 305-310.
[3] ZHAO Jia-chang, DAI Yang, XU Jing-li, CHEN Si-hao, XIE Jing-ying. Synthesis and electrochemical characterizaiton of mesoporous carbons prepared by chemical activation [J]. Journal of the Electrochemical Society, 2008, 155(7): A475-A480.
[4] THOMAS E R, DEMISA H J, ZHU Z H, LU G Q. Mesoporous carbon electrode from waste coffee beans for high performance supercapacitors [J]. Electrochemistry Communications, 2008, 10(10): 1594-1597.
[5] TIAN Yong-ming, SONG Yan, TANG Zhi-hong, GUO Quan-gui, LIU Lang. Influence of high temperature treatment of porous carbon on the electrochemical performance in supercapacitor [J]. Journal of Power Sources, 2008, 184(2): 675-681.
[6] XIA Kai-sheng, GAO Qiu-ming, JIANG Jin-hua, HU Juan. Hierarchical porous carbons with controlled micropores and mesopores for supercapacitor electrode materials [J]. Carbon, 2008, 46(13): 1718-1726.
[7] BARBIERI O, HAHN M, KOTZ R. Capacitance limits of high surface area activated carbons for double layer capacitors [J]. Carbon, 2005, 43(6): 1303-1310.
[8] LAI Yan-qing, LI Jing, SONG Hai-shen, ZHANG Zhi-an, LIU Ye-xiang. Preparation of activated carbons from mesophase pitch and their electrochemical properties [J]. Journal of Central South University of Technology, 2007, 14(5): 601-606.
[9] YANG Jie, SHEN Zeng-min, HAO Zi-biao,. Preparation of highly microporous and mesoporous carbon from the mesophase pitch and its carbon foams with KOH [J]. Carbon, 2004, 42(2): 1872-1875.
[10] KIERZEK K, FRACKOWIAK E, LOTA G, GRYGLEWICZ G, MACHNIKOWSKI J. Electrochemical capacitors based on highly porous carbons prepared by KOH activation [J]. Electrochimica Acta, 2004, 49(4): 515-523.
[11] PRABAHARAN S R S, VIMALA R, ZAINAL Z. Nanostructure mesoporous carbon as electrodes for supercapacitors [J]. Journal of Power Sources, 2006, 161(1): 730-736.
[12] KIM Y J, HORIE Y, MATSUZAWA Y, OZAKI S, ENDO M, DRESSELHAUS S M. Structure features necessary to obtain a high specific capacitance in electric double layer capacitor [J]. Carbon, 2004, 42(12/13): 2423-2432.
[13] HUSSEIN M Z, TARMIZI R S H, ZAINAL Z, IBRAHIM R. Preparation and characterization of active carbons from oil palm shells [J]. Carbon, 1996, 34(11): 1447-1454.
[14] LU W, CHUNG D D L. Mesoporous activated carbon filaments [J]. Carbon, 1997, 35(3): 427-437.
[15] CARVALHO A P, CARDOSO B, PIRES J, CARBALHO M. Preparation of activated carbons from cork waste by chemical activation with KOH [J]. Carbon, 2003, 41(14): 2873-2884.
[16] WU F C, TSENG R L, HU C C, WANG C C. The capacitive characteristics of activated carbons—Comparisons of the activation methods on the pore structure and effects of the pore structure and electrolyte on the capacitive performance [J]. Journal of Power Sources, 2006, 159(2): 1532-1542.
Foundation item: Project(2007BAE12B01) supported by the National Key Technology Research and Development Program of China
Received date: 2008-08-20; Accepted date: 2008-10-08
Corresponding author: ZHANG Zhi-an, PhD; Tel: +86-731-8876454; E-mail: zhianzhang@sina.com
(Edited by CHEN Wei-ping)