J. Cent. South Univ. (2016) 23: 1326-1331
DOI: 10.1007/s11771-016-3183-0

Reduction smelting on bismuth oxide residue in FeO-SiO 2-CaO ternary slag system
ZHANG Du-chao(张杜超), ZHANG Xin-wang(张新望), YANG Tian-zu(杨天足), WEN Jian-feng(文剑锋),
LIU Wei-feng(刘伟锋), CHEN Lin(陈霖), RAO Shuai(饶帅), XIAO Qing-kai(肖庆凯), HAO Zhan-dong(郝占东)
School of Metallurgy and Environment, Central South University, Changsha 410083, China
Central South University Press and Springer-Verlag Berlin Heidelberg 2016
Abstract: Reduction smelting of the bismuth oxide residue from pressure leaching of bismuth sulfide was investigated in the FeO-SiO 2-CaO ternary slag system. The results show that all the recovery ratios of Bi, Ag, Cu and Pb increase with the increase of reductive coal proportion, reaction temperature and time, while too much reductive coal would help Fe enter metal phase; CaO/SiO2 and FeO/SiO2 of the chosen slag system should be 0.5-0.75 and 1.25-1.75, respectively, for the reason that the slag system has the optimum mobility and is beneficial for the recovery of metals. The corresponding optimum conditions are determined as follows: the added coal proportion is 7% of the leaching residue, CaO/SiO2 mass ratio in the chosen slag system is 0.5 and FeO/SiO2 is 1.5, the reaction temperature is 1300 °C and the reaction time is 40 min. Under the above conditions, the recovery ratios of Bi, Ag, Cu and Pb are 99.6%, 99.8%, 97.0% and 97.3%, respectively.
Key words: bismuth oxide residue; reduction smelting; FeO-SiO2-CaO ternary slag system; recovery ratio
1 Introduction
Bismuth is a kind of green metals whose reserves in China are 75% of the world total reserves [1]. The bismuth-bearing materials can be processed with hydrometallurgical or pyrometallurgical method. In the traditional hydrometallurgical methods, the bismuth sulfide concentrate is leached in the hydrochloric system with oxide agent, while the bismuth oxide-containing materials are leached by directly hydrochloric acid [2-3] , and then Bi in the leaching solution is replaced with iron powder or recovered by electro-winning in diaphragm electrolytic bath to produce crude bismuth [4]. Besides, bismuth can be separated by hydrolysis precipitation from the leaching solution in the form of bismuth oxychloride [5] and then reduced to produce crude bismuth. Crude bismuth can be transformed to pure bismuth by pyro-refining or electro-refining. Although the hydrometallurgical process has the advantages of high leaching ratio, low reagent costs and simple operation, the hydrochloric acid system causes serious corrosion and a large number of metal impurities transfer into the leaching solution resulting in difficult subsequent separation, poor metal recovery and serious environmental pollution [6]. In addition, leaching bismuth oxide with fluosilicic acid as the leaching agent has been investigated and the results show that the presence of Fe and Pb in leaching solution is harmful to recover Bi [7-9].
Now, pyrometallurgical smelting is the mainstream technology in bismuth production industry. For bismuth sulfide concentrate, SWINBOURNE has researched the thermodynamic model of bismuth smelting with the coal/iron reduction method [10] and the technology has been used to produce crude bismuth by Hunan Shizhuyuan Nonferrous Metals Co., Ltd. Another traditional method of producing bismuth is reverberatory or blast furnace reduction smelting after oxidizing the bismuth sulfide concentrate with sintering method [11]. The above processes have short flowsheet and high metal recovery, but they have such shortcomings as high costs and serious environmental pollution [12-13]. In recent years, YANG et al [14] have studied bismuth separation from bismuth glance concentrate through low- temperature molten salt smelting. Although the method has high metal recovery, the soda would cause strong corrosion to the refractory. CHEN et al [15] have studied bath smelting of bismuth sulfide concentrate in oxygen side-blown furnace. According to this technology, concentrates are smelted in oxidation furnace, and then bismuth liquid molten slag flows into reduction furnace for reduction smelting. The method can get higher metal recovery, but the effects of technological conditions during smelting on the behavior of bismuth have not been researched in detail.
In this work, the leaching residue is used as the raw material from pressure leaching of bismuth sulfide concentrates to separate tungsten and molybdenum. The leaching residue contains not only such valuable metals as Bi, Ag, Cu and Pb but also some impurity elements of Fe, Si and Ca [16-17]. This work aims to value metal extraction from the leaching residue in the ternary slag system of FeO-SiO2-CaO [18-19], which has been thoroughly studied and used widely in copper and lead pyro-metallurgical process. The behavior of the valuable metals is investigated with various operating parameters, such as coal powder dosage, slag type, reaction temperature and time, so that the optimum reduction smelting conditions can be determined.
2 Experimental
2.1 Materials
The raw material used in this work is the leaching residue containing Bi [20], and its chemical composition is listed in Table 1. The fluxes including calcium oxide and silica are analytical reagents. The reductive coal powder has the chemical composition listed in Table 2.
Table 1 Chemical composition of bismuth oxide residue
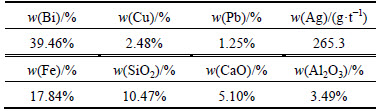
Table 2 Analysis result of reductive coal used in smelting experiment
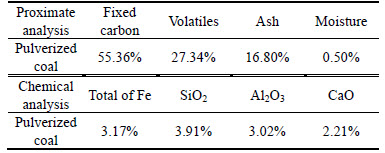
2.2 Experiment procedure
The amount of silica and calcium was calculated according to the FeO-SiO2-CaO ternary slag system. All additives containing silica, calcium oxide, reductive coal were mixed with the leaching residue containing Bi in a mortar and pestle. The mixture was put into a clay crucible and then smelted in a pit furnace with a certain heating system. When smelting was finished, the clay crucible was removed, cooled to room temperature and then carefully broken to obtain two parts of smelting products. The upper part is the smelting slag and the lower part is crude bismuth alloy. Both the two products were weighed. Furthermore, the smelting slag was crushed, mixed and sampled to analyze its metal content and calculate metal recovery ratio.
2.3 Analysis
The main elements in the reactions were bismuth, silver, lead, copper and iron. Gravimetric method, titration method and ICP-AES method were used to quantitatively analyze the proportion of bismuth, lead and copper in the smelting slag. And silver content was determined with fire-assaying method.
The phase composition of the leaching residue and the smelting slag was obtained by X-ray diffraction (XRD) using a Rigaku TTTR-III equipment with Cu Kα radiation (40 kV and 30 mA) and the scanning rate of 10 (°)/min.
3 Results and discussion
3.1 Effect of coal powder amount on reduction smelting
The effects of reductive coal powder amount on metal recovery ratio were investigated by reduction smelting at 1200 °C for 40 min with CaO/SiO2 mass ratio of 0.75 and FeO/SiO2 mass ratio of 1.25. The results in Fig. 1 show that all the metal recovery ratio increases significantly with the increase of the mass ratio of coal powder to residue. The recovery ratio of bismuth, silver, copper and lead increases approximately from 89.6%, 96.9%, 71.6% and 53.8% to 97.8%, 100%, 84.7% and 88.8% when coal powder amount increases from 4% to 7%. Under the above conditions, the silver content in smelting slag is very low because of easy enrichment of silver in the metal alloy.
Although the recovery ratio of most valuable metals has a positive correlation with coal powder amount, such unwanted metals as iron were still reduced into crudebismuth alloy. The iron content in crude bismuth alloy increases from 0.2% to 10% with coal powder amount increasing from 7% to 10%. In order to avoid unwanted metals in crude bismuth alloy, the coal powder amount should be controlled.
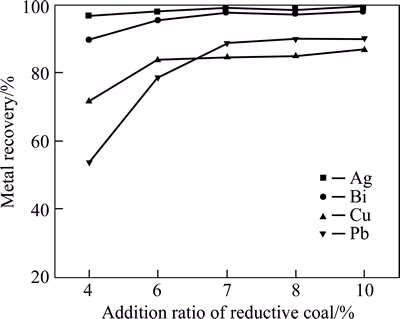
Fig. 1 Effect of adding ratio of reductive coal on metal recovery (Conditions: m(FeO)/m(SiO2)=1.25, m(CaO)/m(SiO2)=0.75,smelting time 40 min, 1200 °C)
3.2 Effect of ternary slag type on reduction smelting
The effects of CaO/SiO2 mass ratio and FeO/SiO2 mass ratio on metal recovery ratio were investigated by reduction smelting at 1200 °C [21] for 40 min with a coal addition of 6%. The results in Fig. 2 show that FeO-to-SiO2 mass ratio has an obvious influence on metal recovery. The maximum bismuth recovery ratio is 95.2% with FeO-to-SiO2 mass ratio of 1.25. The bismuth content in the smelting slag decreases approximately from 5.7% to 3% with FeO-to-SiO2 mass ratio increasing from 1.0% to 1.25%. The further increase of FeO-to- SiO2 mass ratio has no obvious influence on bismuth recovery. The recovery ratios of lead and copper are only 43.6% and 75.1%, respectively, with FeO-to-SiO2 mass ratio of 1.0. When FeO-to-SiO2 mass ratio ranges from 1.25 to 1.75, the recovery ratios of both lead and copper increase a little and then almost keep constant.
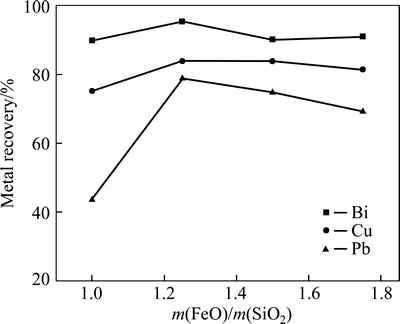
Fig. 2 Effect of change of m(FeO)/m(SiO2) on metal recovery (Conditions: m(CaO)/m(SiO2)=0.75, smelting time 40 min, 1200 °C, coal additional proportion 6%)
The effect of FeO-to-SiO2 mass ratio on metal recovery ratio is shown in Fig. 3 with CaO-to-SiO2 mass ratio of 0.5. From Fig. 3, the maximum bismuth recovery ratio is 97.2% with the lowest bismuth content of 2.18% in the smelting slag. The increase of FeO-to-SiO2 mass ratio improves the bismuth recovery, which can be explained that the increase of FeO-to-SiO2 mass ratio can significantly lower the melting point and viscosity of the smelting slag resulting in easier separation of Bi from the slag. For the ternary system of FeO-SiO2-CaO, when FeO-to-SiO2 mass ratio ranges from 1.5 to 1.75, the melting temperature of the smelting slag is about 1100-1150 °C. Therefore, the experimental temperature 1200 °C is beneficial for increasing slag fluidity. It canbe concluded that the reduction smelting process would be improved by decreasing the melting temperature and viscosity of smelting slag.
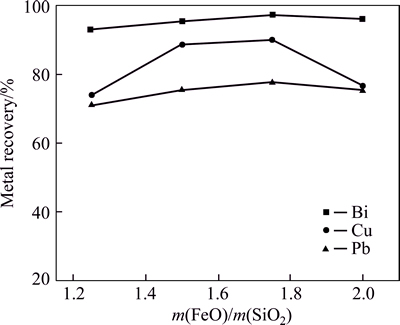
Fig. 3 Effect of change of m(FeO)/m(SiO2) on metal recovery (Conditions: m(CaO)/m(SiO2)=0.5, smelting time 40 min, fining time 20 min, 1200 °C, coal additional proportion 6%)
The effect of CaO-to-SiO2 mass ratio on metal recovery is shown in Fig. 4 with FeO-to-SiO2 mass ratio of 1.5. The bismuth and lead recovery ratios are only 90.5% and 49.7%, respectively, with CaO-to-SiO2 ratio of 1.0. For the ternary system of FeO-SiO2-CaO, the melting temperature of the smelting slag is about 1250 °C when FeO-to-SiO2 and CaO-to-SiO2 mass ratios are 1.5 or 1.0. However, too high melting temperature of the smelting slag is harmful for the smelting process resulting in direct bismuth recovery ratio decreased. When CaO-to-SiO2 mass ratio is 0.35, higher bismuth recovery ratio (96.3%) is obtained. Therefore, CaO-to-SiO2 ratio should be controlled at a lower level during smelting.
Phase compositions of the typical reduction smelting slag were analyzed by XRD. Figure 5 shows XRD pattern of the slag with FeO/SiO2 mass ratio of 1.5and CaO-to-SiO2 mass ratio of 0.35, where the main phases are Fe2O3, CaO·Al2O3·2SiO2 and 2CaO·Al2O3·SiO2. The formation of CaO·Al2O3·2SiO2 and 2CaO·Al2O3·SiO2 is attributed to the slagging reaction between Al2O3 in leaching residue and the additives of CaO and SiO2. Although the melting temperature of CaO·Al2O3·2SiO2 and 2CaO·Al2O3·SiO2 is relatively high, CaO·Al2O3·2SiO2 and 2CaO·Al2O3·SiO2 could still form due to their relatively low content in the slag.
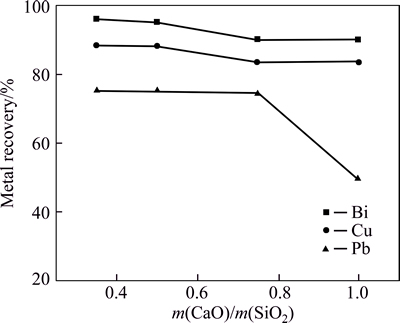
Fig. 4 Effect of change of CaO/SiO2 on metal recovery (Conditions: m(FeO)/m(SiO2)=1.5, smelting time 40 min, fining time 20 min, 1200 °C, coal additional proportion 6%)
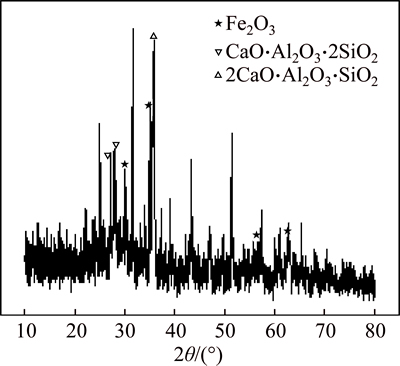
Fig. 5 XRD pattern at m(FeO)/m(SiO2)=1.5 and m(CaO)/ m(SiO2)=0.35
The XRD pattern of the reduction smelting slag with FeO/SiO2 mass ratio of 1.0 and CaO-to-SiO2 mass ratio of 0.75 is shown in Fig. 6, where the main phases are FeO·Fe2O3, CaO·FeO·2SiO2 and SiO2. The high CaO content can promote the transformation of the complex anion (SixOy)z- into smaller anions. So, the characteristic peaks of SiO2 can be observed in Fig. 6. The existence of CaO·FeO·2SiO2 is consistent with SiO2-CaO-FeO phase diagram. The characteristic peaks of Fe2O3 can be observed from Fig. 5 and Fig. 6, showing that reductive coal addition is inadequate to reduce Fe2O3 into FeO. Therefore, a low amount of reductive coal is not conducive to smelting.
A series of optimal experiments were conducted with various mass ratio of coal powder to residue. The experimental conditions and results are given in Table 3. From Table 3, the bismuth and lead recovery ratios are above 97% and 88%, respectively, with FeO/SiO2 mass ratio of 1.25-1.75 and CaO/SiO2 mass ratio of 0.5-0.75. The copper recovery ratio is 91.99% with FeO-to-SiO2 mass ratio of 1.5 and CaO-to-SiO2 mass ratio of 0.5. Therefore, the optimal slag type is of FeO-to-SiO2 mass ratio at 1.5 and CaO-to-SiO2 mass ratio at 0.5.
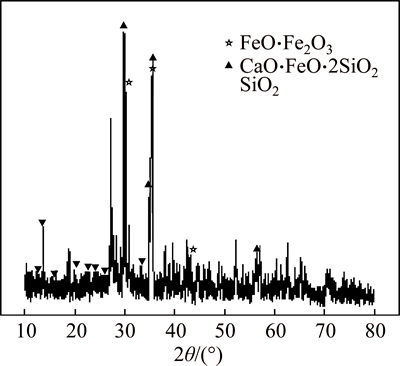
Fig. 6 XRD pattern at m(FeO)/m(SiO2)=1.0 and m(CaO)/ m(SiO2)=0.75
The phase composition of smelting slag in the optimal slag system is determined by XRD, and its XRD pattern is shown in Fig. 7. The characteristic peaks of CaO·Al2O3·2SiO2 and 2FeO·SiO2 appear in Fig. 7. The optimal slag type is around the lowest melting point area in FeO-SiO2-CaO ternary phase diagram, and the melting point of fayalite is relatively low, so it can be concluded that the main phase in smelting slag is 2FeO·SiO2.
Table 3 Further selection and optimization of slag constitution
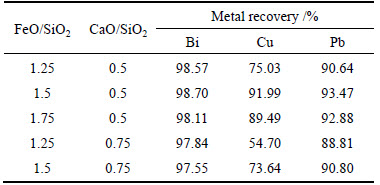
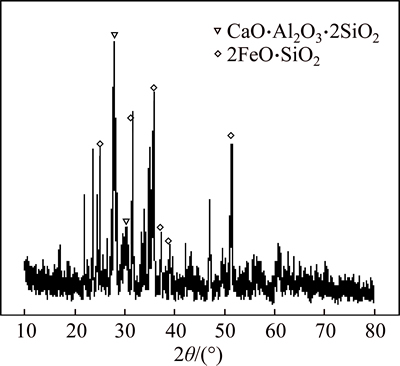
Fig. 7 XRD pattern at m(FeO)/m(SiO2)=1.5, m(CaO)/m(SiO2)= 0. 5 and 1200 °C
3.3 Effect of temperature on reduction smelting
The effect of smelting temperature on metal recovery is shown in Fig. 8, where the higher the temperature, the higher the bismuth recovery ratio.
The recovery ratios of bismuth, silver, copper and lead at 1100 °C are 97.1%, 98.1%, 91.4% and 91.3%, respectively. The recovery ratio of bismuth reaches 99% and its content in smelting slag is less than 0.17% at the temperature over 1300 °C. Increasing temperature notonly accelerates reduction reaction but also decreases the viscosity of smelting slag to promote the settling separation of metal phase from slag phase. The phase composition of smelting slag at 1300 °C and 1400 °C is analyzed by XRD, having the results in Fig. 9 and Fig. 10. The characteristic peaks of silica appear, but the characteristic peaks of other products are not observed as expected in Fig. 9 and Fig. 10. The results demonstratethat the composition of the smelting slag is very complex and similar to amorphous structure.
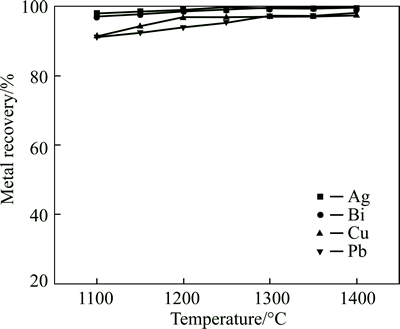
Fig. 8 Effect of temperature on metal recovery (Conditions: m(FeO)/m(SiO2)=1.5, m(CaO)/m(SiO2)=0.5, smelting time 40 min, fining time 20 min, coal additional proportion 7%)
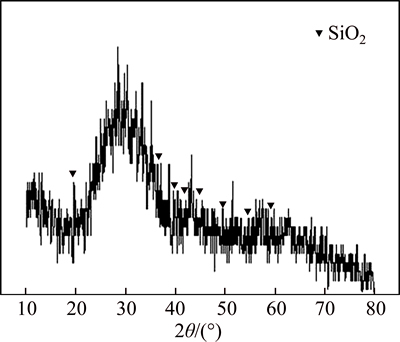
Fig. 9 XRD patterns at m(FeO)/m(SiO2)=1.5, m(CaO)/ m(SiO2)=0. 5 and 1300 °C
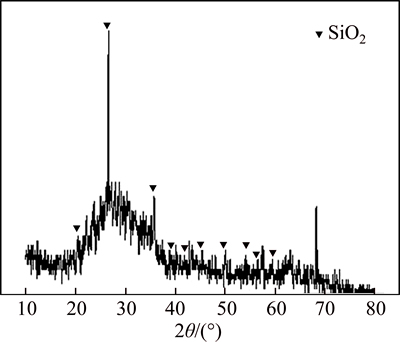
Fig. 10 XRD patterns at m(FeO)/m(SiO2)=1.5, m(CaO)/ m(SiO2)=0. 5 and 1400 °C
3.4 Effect of reaction time on reduction smelting
The effect of smelting time on metal recovery is shown in Fig. 11, where it is can be seen that prolonging reaction time slightly improves metal recovery ratio. The bismuth recovery ratio increases from 96.7% to 99.0% with reaction time increasing from 20 min to 80 min, showing that increasing reaction time is helpful for more complete reduction and settling separation. However, prolonging reaction time decreases production efficiency and increases energy consumption. Therefore, the optimal smelting time is selected at 40 min. The recovery ratios of bismuth, silver, copper and lead are 99.6%, 99.8%, 97.0% and 97.3%, respectively, realizing efficient recovery of bismuth, silver, copper and lead.
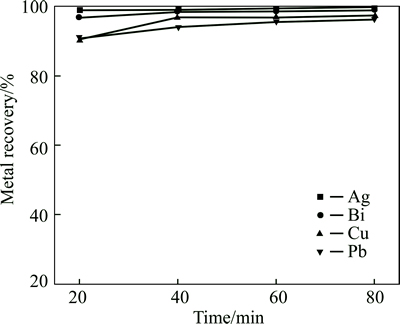
Fig. 11 Effect of smelting time on metal recovery (Conditions: m(FeO)/m(SiO2)=1.5, m(CaO)/m(SiO2)=0.5, fining time 20 min, coal additional proportion 7% and 1200 °C)
4 Conclusions
1) Reduction smelting of bismuth oxide residue from pressure leaching of bismuth sulfide was investigated in the FeO-SiO 2-CaO ternary slag system. The results show that all the recovery ratios of Bi, Ag, Cu and Pb increase with the increased reductive coal proportion, reaction temperature and time. But too much reductive coal would help Fe enter metal phase.
2) The chosen slag system range of m(CaO)/m(SiO2) and m(FeO)/m(SiO2) would be 0.5-0.75 and 1.25-1.75, respectively, for the reason that the slag system in the above range has the optimum mobility and is beneficial for metal recovery. Fayalite phase would appear in smelting slag system according to XRD analysis.
3) The corresponding optimum conditions are determined as follows: smelting temperature of 1300 °C, smelting time of 40 min, coal addition of 7%, CaO/SiO2 mass ratio of 0.5 and FeO-to-SiO2 mass ratio of 1.5. Under the above conditions, the recovery ratios of bismuth, silver, copper and lead are 99.6%, 99.8%, 97.0% and 97.3%, respectively, realizing efficient recovery of bismuth, silver, copper and lead.
References
[1] WANG Li-guo. Bismuth metallurgical [M]. Beijing: Metallurgical Industry Press, 1989: 3-6, 18-46, 72-98. (in Chinese)
[2] KIM D, WANG Shi-jie. Bismuth recovery from hydrochloric acid solution [J]. Canadian Metallurgical Quarterly, 2008, 47(3): 317-326.
[3] ZHENG Guo-qu, TANG Mo-tang. Physico-chemistry in distillation process of BiCl3-HCl-H2O system [J]. Transactions of Nonferrous Metals Society of China, 2002, 12(5): 987-991.
[4] WANG Ji-feng, LI Jing, YANG Jian-guang. Recovery of bismuth from a bismuthinite concentrate by membrane-electrowinning [J]. Hydrometallurgy of China, 2012(1): 45-48.
[5] WANG Yun-yan, PENG Wen-jie, CHAI Li-yuan. Thermodynamic equilibrium of bismuth hydrometallurgy in chloride and nitrate solutions [J]. Journal of Central South University of Technology, 2004, 11(4): 410-413.
[6] KHMELNITSKAYAO D, BESKROVNAYAV P. A technology of precious metals recovery from gold-bismuth ores [C]// World Gold 2007 by the Co-Products and the Environment-Proceedings. Australasian Institute of Mining and Metallurgy Publication Series, 2007: 245-250.
[7] ZHANG Du-chao. Tungsten and molybdenum separation from bismuth by alkaline pressure oxidation of bismuth sulfide concentrate [D]. Changsha: Central South University, 2012. (in Chinese)
[8] WEI Chang, WANG Jun-zhong, JIANG Qi. Some knowledge of H2SiF6 and its salts [J]. Journal of Kunming University of Science and Technology, 2001(6): 97-100.
[9] ZHANG Chuan-fu, ZHANG Xiang, ZHAN Jing. A new process of fluosilicic acid leaching for recovering bismuth from materials containing bismuth oxide [C]// TMS Annual Meeting. United States: Minerals, Metals and Materials Society, 2014: 413-424.
[10] SWINBOURNE D R. Thermodynamic modeling of bismuth smelting by the coal/iron reduction method [C]// A New Century International Conference on Metallurgical High Technology and New Materials of Heavy Nonferrous Metals. Kunming, China, 2002: 53-61.
[11] YANG Tian-zu,LI Jun, LIU Wei-feng,CHEN Lin,BIN Wan-da. Development of bismuth smeltingtechnology in China [C]// TMS Annual Meeting. United States: Minerals, Metals and Materials Society, 2013: 631-642.
[12] Beijing Nonferrous Metallurgy Design and Research Institute. Heavy non-ferrous metal smelting design manual (Lead, zinc and bismuth volume) [M]. Beijing: BeijingIndustry Press, 1996: 643-652, 672-676. (in Chinese)
[13] PENG Rong-qiu. Non-ferrous metals extraction manual (Zinc, cadmium, lead and bismuth volume) [M]. Beijing: BeijingIndustry Press, 1992: 423-424. (in Chinese)
[14] YANG Jian-guang, HE De-wen, TANG Chao-bo, CHEN Yong-ming, SUN Ya-hui, TANG Mo-tang. Thermodynamics calculation and experimental study on separation of bismuth from a bismuth glance concentrate through a low-temperature molten salt smelting process [J]. Metallurgical and Materials Transactions B: Process Metallurgy and Materials Processing Science, 2011(8): 730-737.
[15] CHEN Lin, BIN Wan-da, YANG Tian-zu, LIU Wei-feng, BIN Shu. Research and industrial application of oxygen-rich side-blow bath smelting technology [C]// TMS Annual Meeting. United States: Minerals, Metals and Materials Society, 2013: 49-55.
[16] XIAO Jian-fei, TANG Chao-bo, TANG Mo-tang, CHEN Yong-ming. Study on a new process for alkaline smelting bismuth sulfide concentrate at low temperature [J]. Mining and Metallurgical Engineering, 2009, 29(5): 82-85.
[17] KHANTURGAEVA G I, ZOLTOEV E V, URBAZAEVA S D. Technology for processing the bismuth-silver concentrates [J]. Fiziko-Tekhnicheskie Problemy Razrabotki Poleznykh Iskopaemykh, 2002(1): 103-107.
[18] MARGULIS, EFIM V. Low temperature smelting of lead metallic scrap [J]. Erzmetall, 2003, 53(2): 85-89.
[19] PICKLES C A, TOGURI J M. Soda ash smelting of lead chloride [J]. Canadian Metallurgical Quarterly, 1988, 27(2): 117-122.
[20] ZHANG Du-chao, YANG Tian-zu, LIU Wei-feng, WU jiang-hua. Pressure leaching of bismuth sulfide concentrate containing molybdenum and tungsten in alkaline solution [J]. Journal of Central South University, 2012, 19(12): 3390-3395.
[21] LI Hong-gui. Metallurgical principles [M]. Beijing: Science Press, 2009: 39-43. (in Chinese)
(Edited by YANG Bing)
Foundation item: Project(134414) supported by the Postdoctoral Funded Program of Central South University, China
Received date: 2015-03-25; Accepted date: 2015-09-23
Corresponding author: YANG Tian-zu, Professor; Tel: +86-731-88830923; E-mail: tianzuyang@csu.edu.cn