
Effects of TiN film coating on electrochemical behaviors of
nanotube formed Ti-xHf alloys
Kang LEE1, Won-Gi KIM1, Joo-Young CHO1, Sang-Won EUN2, Han-Cheol CHOE1
1. Department of Dental Materials & Research Center of Nano-Interface Activation for Biomaterials,
College of Dentistry, Chosun University, Gwangju, 520-825, Korea;
2. Department of Applied Advanced Materials, Korea Polytechnic Ⅴ Colleges, Gwangju, 501-759, Korea
Received 18 June 2008; accepted 10 March 2009
Abstract: Ti-xHf (x=10%, 20%, 30% and 40%, mass fraction) alloys were prepared by arc melting, and the microstructure was controlled for 24 h at 1 000 ℃ in argon atmosphere. The formation of nanotube was conducted by anodizing on Ti-Hf alloys in 1.0 mol/L H3PO4 electrolytes with small amounts of NaF at room temperature. And then TiN coatings were coated by DC-sputtering on the anodized surface. Microstructures and nanotube morphology of the alloys were examined by field emission scanning electron microscopy(FE-SEM) and X-ray diffractometry(XRD). The corrosion properties of the specimens were examined through potentiodynamic test (potential range from -1 500 to 2 000 mV) in 0.9 % NaCl solution by potentiostat. The microstructure shows the acicular phase and α′ phase with Hf content. The amorphous oxide surface is transformed to crystalline anatase phase. TiN coated nanotube surface has a good corrosion resistance.
Key words: Ti alloy; nanotube; TiN coating; corrosion behaviors
1 Introduction
The electrochemical formation of self-organized porous nanostructures has attracted much interest, since they possess wide applications in biological nano- patterning, high-density recording media and templates for nanomaterials. Especially, hafnium belongs to the noble metal group and hafnium oxides have numerous interesting properties, for example, a high chemical stability, a high thermal stability, a high refractive index and a relatively high dielectric constant[1-2].
It is known that titanium nitride (TiN) has superior mechanical and chemical properties, such as high hardness, good wear resistance and chemical stability. TiN is commonly used as a wear and corrosion resistant film on cutting tools[3], machine components[4], and also biomaterials[5]. The TiN-coated Ti with sufficient biocompatibility is promising as an implant material[6]. Although titanium nitride coatings on Ti have been widely studied for biomedical applications in simulated human body environments[6-9], its application in oral environments is still limited.
In this work, the effects of TiN film on the electro- chemical behaviors of nanotube formed Ti-xHf alloys have been researched by using various electrochemical methods.
2 Experimental
Ti-Hf alloys with alloying element content from 10% to 40% (mass fraction) were melted by using a vacuum furnace and then homogenized for 24 h at 1 000 ℃. The surface of the plate specimen (3 mm thick) was polished mechanically with 1 μm Al2O3 paste. Ultrasonic cleaning with ethanol, distilled water, and drying with air followed this process. Electrochemical experiments were carried out with conventional three-electrode configuration with a platinum counter electrode and a saturated calomel reference electrode. The sample was embedded with epoxy resin, leaving a square surface area of 1 cm2 exposed to the anodizing electrolyte, 1 mol/L H3PO4 containing 0.5% NaF. Anodization treatments were carried out using a scanning potentiostat (EG&G Co, Model 362, USA). All experiments were conducted at room temperature. The electrochemical treatment was first swept from the open-circuit potential to desired final potential with a sweep rate of 500 mV/s, and then the potential was held for 2 h. After the treatments the anodized samples were rinsed with distilled water and dried with dry air stream. The TiN coatings were produced by DC-sputtering on anodized layer, using a target of Ti(99.99%). DC power of 100 W was applied to the target during 40 min for all the depositions, with a constant substrate temperature of 150 ℃. A set of coating was obtained with constant argon and N2 mixed gas flow of 40 cm3/min.
Film surface topology, chemical composition, and crystal structure were determined using FE-SEM and XRD. The corrosion behaviors were investigated using potentiostat (Model 2273, EG&G Co., USA) in 0.9% NaCl solution at (36.5±1) ℃.
3 Results and discussion
Fig.1 shows the microstructures of Ti-Hf alloys after performing homogenization heat treatment. One can assume that the variation of Ti-Hf alloys is due to the changes in microstructure caused by Hf content. The microstructure of Ti-40%Hf alloy is related to its needle- like structure of α′-phase. Needle-like traces were observed at all specimens, and the amount of needle-like traces in quenched alloy was more than that of furnace cooled alloys[10]. The fine needle-like structure was observed in the image of Ti-40Hf alloy. It was confirmed that Hf elements acted to form a martensitic phase and the grain growth was effectively suppressed [11].
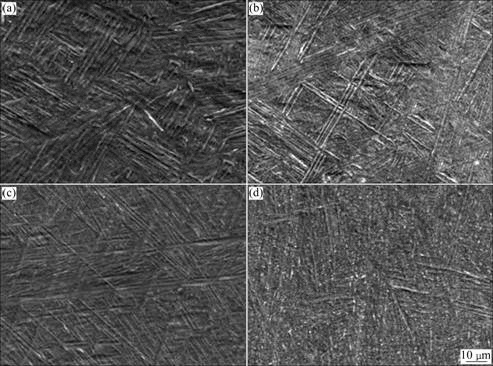
Fig.1 FE-SEM images of heat treated Ti-xHf alloys: (a) Ti-10Hf; (b) Ti-20Hf; (c) Ti-30Hf; (d) Ti-40Hf
Fig.2 shows the X-ray diffraction patterns of water quenched Ti-(10%, 20%, 30% and 40%)Hf alloys. The peaks were indexed using the JCPDS diffraction data. It can be seen that only the reflections from α′-phase were identified by XRD. The α′-phase in Ti-(10%, 20%, 30% and 40%)Hf alloys proceeded through a phase reaction leading to the formation of hcp structure[12].
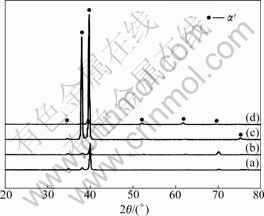
Fig.2 XRD patterns of Ti alloys with different Hf contents: (a) Ti-10Hf; (b) Ti-20Hf; (c) Ti-30Hf; (d) Ti-40Hf
Fig.3 shows the top images of the porous layer formed at 10 V. The nanotube size was changed with Hf contents. Evident nanotube structures with large diameter formed, with an average inner diameter of 100 nm and a tube wall thickness of about 20 nm. The small tube was formed in specimen containing high Hf content. Although HfO2 is probably the most stable metal oxide against chemical dissolution in many environments, it can be dissolved in HF-containing electrolytes, forming a soluble fluoro-complex [13]. The reactions for the nanotube formation on Ti-30Hf alloy can be represented as follows:
[M]O2+4H++6F-→
+2H2O (1)
where M is the metal. Therefore, M plays an important role for the growth of nanotubes and their initial nucleation in HF-containing electrolytes. It was confirmed that the anodic oxide layer (HfO2) on Ti-Hf interrupted the formation of TiO2 nanotubes on the anodized Ti-Hf surface. The chemical dissolution rate was small due to the formation of HfO2, therefore, smaller-sized nano- tubes were formed[14].
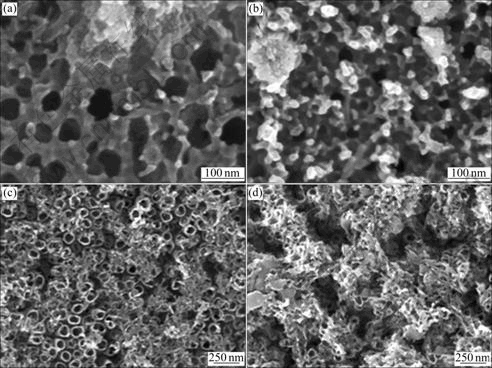
Fig.3 FE-SEM images of Ti-Hf alloy surface in 1 mol/L H3PO4+0.5% NaF solution after nanotube formation for 2 h at 10 V: (a) Ti-10Hf; (b) Ti-20Hf; (c) Ti-30Hf; (d) Ti-40Hf
Fig.4 shows the XRD patterns of nanotube formed Ti-xHf alloys by crystallizing in Ar atmosphere for 1 h at 550 ℃. It can be seen that anatase peak was detected predominantly with increasing Hf content. That is, hafnium dioxide plays role to affect the crystallization of nanotube formed surface.
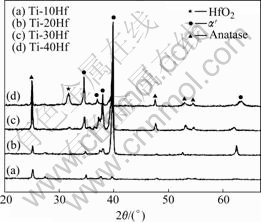
Fig.4 XRD patterns of Ti-xHf alloys after nanotube formation at 10 V and then heat treatment at 550 ℃ for 1 h.
The crystallinity of nanotube is important to improve the biocompatibility and corrosion resistance. After annealing of Ti-40Hf at 550 ℃, anatase structure appeared predominantly, but the major TiO2 phase remains to be anatase. It may be noteworthy that in general the mixed oxide layers on Ti-Hf have a higher mechanical and chemical stability than pure TiO2 nanotubes.
Fig.5 shows the potentiodynamic polarization curves for Ti-xHf alloys tested in NaCl solution at (36.5± 1) ℃. The corrosion potentials(φcorr) ranged from -500 to -570 mV for all specimens. The φcorr, and corrosion current densities(Jcorr) for the alloys obtained from their polarization curves are summarized in Table 1. The Jcorr values obtained from the polarization curves using the Tafel extrapolation method were 0.538, 0.206, 0.149 and 0.354 μA/cm2 for Ti-10Hf, Ti-20Hf, Ti-30Hf and Ti-40Hf, respectively. Ti-30Hf alloy showed a good corrosion resistance due to the formation of HfO2 on the surface [15].
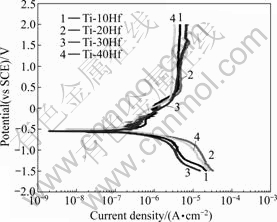
Fig.5 Potentiodynamic polarization curves for Ti-xHf alloys tested in 0.9% NaCl solution at (36.5±1) ℃
Table 1 Corrosion potential(φcorr) and current density(Jcorr) of Ti-xHf alloys after anodic polarization test in 0.9% NaCl solution at (36.5±1) ℃
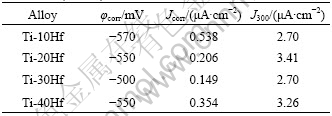
Fig.6 shows the potentiodynamic polarization curves for the nanotube formed Ti-xHf alloys tested in NaCl solution at (36.5±1) ℃. The φcorr ranged from -610 to -1 320 mV for all alloys. The corrosion potential of Ti-40Hf alloy was higher than that of the other alloys in 0.9% NaCl solution due to the formation of HfO2 on the surface[15].
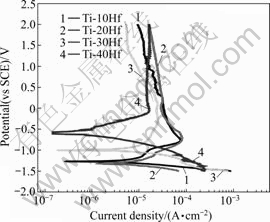
Fig.6 Potentiodynamic polarization curves of nanotube formed Ti-xHf after potentiodynamic test in 0.9% NaCl solution at (36.5±1) ℃
The φcorr, and Jcorr for the alloys obtained from their polarization curves are summarized in Table 2. The Jcorr values obtained from the polarization curves using the Tafel extrapolation method were 8.844, 16.85, 12.10 and 0.957 μA/cm2 for Ti-10Hf, Ti-20Hf, Ti-30Hf and Ti-40Hf, respectively. The corrosion resistance of nanotube formed surface was lower than that of non-nanotube surface. It was confirmed that nanotube surface served the site of corrosion by Cl- ion.
Table 2 Corrosion potential(φcorr) and current density(Jcorr) of nanotube formed Ti-xHf alloys after potentiodynamic test in 0.9% NaCl solution at (36.5±1) ℃
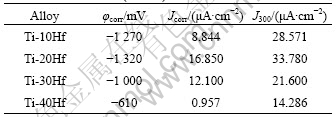
Fig.7 shows the potentiodynamic polarization curves for TiN coated and nanotube formed Ti-xHf alloys in NaCl solution at (36.5±1) ℃. The φcorr ranged from -660 to -960 mV for all alloys. The corrosion potential of TiN coated and nanotube formed Ti-40Hf alloy was higher than that of the other alloys in 0.9% NaCl. It was confirmed that TiN coated and nanotube formed surface played role to prohibit the attack of Cl- ion.
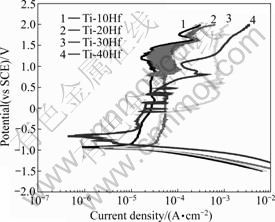
Fig.7 Potentiodynamic polarization curves of TiN coated and nanotube formed Ti-xHf after potentiodynamic test in 0.9% NaCl solution at (36.5±1) ℃
The φcorr and Jcorr for the alloys obtained from their polarization curves are summarized in Table 3. The Jcorr obtained from the polarization curves using the Tafel values extrapolation method were 2.081, 33.19, 26.10 and 3.857μA/cm2 for Ti-10Hf, Ti-20Hf, Ti-30Hf and Ti-40Hf, respectively.
Table 3 Corrosion potential(φcorr) and current density(Jcorr) of TiN coated and nanotube formed Ti-xHf alloys after potentio- dynamic test in 0.9% NaCl solution at (36.5±1) ℃
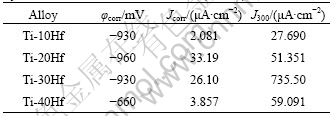
In the case of TiN coating and nanotube formation, Ti-Hf alloys have a good potential for biomedical applications.
4 Conclusions
1) The microstructures of Ti-Hf alloys were changed to acicular and α′-phase with increasing amount of Hf in the Ti-xHf alloys.
2) The amorphous oxide surface can be transformed to crystalline anatase phase by appropriate heat treatment.
3) From the results of polarization behavior in the Ti-xHf alloys, the current density of nanotube formed Ti-40Hf in the passive region was lower than that of the other alloys. The polarization curves of the TiN-coated and nanotube formed alloys were shifted to the right side due to the formation of protection layer against Cl- ion.
Acknowledgement
This research was supported by National Research Foundation of Korea (2009-0074672).
References
[1] THOMPSON G E. Porous anodic alumina: Fabrication, characterization and application [J]. Thin Solid Films, 1997, 297: 192-201.
[2] SUNDQUVIST J, HARSTA A, AARIK J, KUKLI K, AIDLA A. Atomic layer deposition of polycrystalline HfO2 films by the HfI4-O2 precursor combination [J]. Thin Solid Films, 2003, 427: 147-151.
[3] TUFFY K, BYRNE G, DOWLING D. Determination of the optimum TiN coating thickness on WC inserts for machining carbon steels [J]. J Mater Process Technol, 2004, 155: 1861-1866.
[4] CUI Z D, ZHU S L, MAN H C, YANG X J. Microstructure and wear performance of gradient Ti/TiN metal matrix composite coating synthesized using a gas nitriding technology [J]. Surf Coat Technol, 2005, 190: 309-313.
[5] CZARNOWSKA E, WIEZCHON T, MARAN-NIEDBA A. Properties of the surface layers on titanium alloy and their biocompatibility in in vitro tests [J]. J Mater Process Technol, 1999, 92: 190-194.
[6] WATARI F, TAMURA Y, YOKOYAMA A, UO M, KAWASAKI T. Mechanical properties and biocompatibility of surface-nitrided titanium for abrasion resistant implant [J]. Key Eng Mater, 2004, 254/256: 873-876.
[7] GOLDBERG J R, GILBERT J L. In vitro corrosion testing of modular hip tapers [J]. J Biomed Mater Res B: Appl Biomater, 2003, 64B: 78-93.
[8] PASCHOAL A L, VANANCIO E C, CANALE L, DE CAMPOS F, DA SILVA O L, HUERTA-VILCA D, DE JESUS M A. Metallic biomaterials TiN-coated: Corrosion analysis and biocompatibility [J]. Artif Organs, 2003, 27: 461-464.
[9] TAMURA Y, YOKOYAMA A, WATARI F, KAWASAKI T. Surface properties and biocompatibility of nitrided titanium for abrasion resistant implant materials [J]. Dent Mater J, 2002, 21: 355-372.
[10] JEONG Y H, CHOE H C, KO Y M, PARK S J, KIM D K, BRANTLEY W. Mechanical properties of nano-structure controlled Ti-Hf alloy for dental [J]. NSTI-Nanotech, 2007, 2: 721-724.
[11] HE G, HAGIWARA M. Ti alloy design strategy for biomedical applications [J]. Mater Sci Eng C, 2006, 26: 14-19.
[12] HON Y H, WANG J Y, PAN Y N. Influence of hafnium content on mechanical behaviors of Ti-40Nb-xHf alloys [J]. Mater Lett, 2004, 58: 3182-3186.
[13] WEI W, MACAK J M, SCHUMUKI P. High aspect ratio ordered nanoporous Ta2O5 films by anodization of Ta [J]. Electrochem Commun, 2008, 10: 428-432.
[14] KANECO S, CHEN Y, WESTERHOFF P, CRITTENDEN J C. Fabrication of uniform size titanium oxide nanotubes: Impact of current density and solution conditions [J]. Scripta Mater, 2007, 56: 373-376.
[15] CAI Z, KOIKE M, SATO H, BREZNER M, GUO Q, KOMATSU M, OKUNO O, OKABE T. Electrochemical characterization of cast Ti-Hf binary alloys [J]. Acta Biomater, 2005, 1: 353-356.
Corresponding author: Han-Cheol CHOE; Tel: +82-62-230-6896; E-mail: hcchoe@chosun.ac.kr
DOI: 10.1016/S1003-6326(08)60364-7
(Edited by YUAN Sai-qian)