Trans. Nonferrous Met. Soc. China 23(2013) 1633-1638
Phase diagram of Sm-Nd-Fe ternary system
Bo-wen WANG1,2, Wen-mei HUANG1, Zhi-chao JI1, Zhi-hua WANG1, Ying SUN1, Ling WENG1
1. Province-Ministry Joint Key Laboratory of Electromagnetic Field and Electrical Apparatus Reliability,Hebei University of Technology, Tianjin 300130, China;
2. International Center for Materials Physics, Chinese Academy of Sciences, Shenyang 110016, China
Received 4 May 2012; accepted 11 March 2013
Abstract: Phase equilibria of the Sm-Nd-Fe ternary system were determined in an isothermal section of 500 °C and vertical sections of SmFe2–NdFe2 and (Sm0.86Nd0.12)Fex (1.6≤x≤2.4) by optical microscopy, X-ray diffraction analysis, electron probe microanalysis (EPMA), and differential thermal analysis (DTA) techniques. There are four intermetallic phases: (Sm,Nd)Fe2, (Sm,Nd)Fe3, (Sm,Nd)5Fe17 and (Sm,Nd)2Fe17 in the Sm-Nd-Fe ternary system, and no (Sm,Nd)6Fe23 phase exists in this system. The isothermal section of 500 °C possesses 7 single-phase regions, 8 two-phase regions, and 4 three-phase regions. The vertical section of SmFe2-NdFe2 contains 2 single-phase regions, 4 two-phase regions, and 7 three-phase regions, and that of (Sm0.88Nd0.12)Fex consists of 2 single-phase regions, 5 two-phase regions, and 2 three-phase regions. The peritectic temperatures for (Sm1-xNdx)Fe2 alloys decrease with increasing the Nd content when x≤0.5, and the substitution of Nd for Sm makes the stability of (Sm,Nd)Fe2 phase decrease.
Key words: Sm-Nd-Fe system; phase diagram; structure; compound
1 Introduction
Intermetallic compounds of rare-earth and transition metal have been widely utilized as permanent magnets, magnetostrictive materials, and magnetic recording media. Among these systems, the Sm–Fe system has been the focus of considerable attention and extensive studies because its compounds have high spontaneous magnetization and remain magnetic at relative high temperatures [1,2]. In actual magnets, other rare-earth elements are added in order to induce special magnetic properties. For example, the replacement of Sm by Dy or Nd in SmFe2 compound can make the magnetocrystalline anisotropies of RFe2 compounds reduced because the magnetocrystalline anisotropies are dominated by the rare-earth ions. The investigation of the magnetostriction and anisotropy compensation for Sm1-xNdxFe2 [3] and Sm0.88Nd0.12(Fe1-xCox)1.93 [4] has found that the (Sm,Nd)Fe2 compounds possess very high saturation magnetostriction. Structure, magnetic properties and magnetostriction of Sm1-xNdxFe1.55 alloys were studied in Ref. [5], and it was found that the alloys with 0≤x≤0.48 contain mainly cubic Laves phase, besides minor rare earths. However, the metallurgical process of Sm-Fe alloys is very complex [6] and it is necessary to have the knowledge of solidification process of Sm-Nd-Fe alloys. Therefore, it is interesting to investigate the phase diagram of the Sm-Nd-Fe ternary system in order to develop a new type of magnetostrictive material and permanent magnetic rare earth-iron alloys.
The Sm-Fe binary system contains SmFe2, SmFe3 and Sm2Fe17 phases [7,8]. Two phases, Nd5Fe17 and Nd2Fe17, exist in the Nd-Fe system [7,8], nevertheless, the cubic Laves compound NdFe2 has been successfully prepared by high pressure technique. There are α-Nd and α-Sm phases in the Sm-Nd system at room temperature [7]. The crystallographic data of their compounds are summarized in Table 1 [7-9]. Up to now, the phase diagram of the Sm-Nd-Fe ternary system has not been published. In this work, the isothermal section of 500 °C, and vertical sections of SmFe2-NdFe2 and (Sm0.86Nd0.12)Fex (1.6≤x≤2.4) are investigated for the Sm-Nd-Fe ternary system.
2 Experimental
Alloys were prepared from materials with the following purities: Sm, Nd, 99.9% (mass fraction) and Fe 99.8%. Pure metals are made into small pieces, mixed according to the stoichiometry and compacted into pellets. The pellets were heated in an alumina crucible to 400 °C and kept at 400 °C for 10 h under high-purity argon in order to obtain composition-homogenized samples. To compensate for evaporation loss of Sm during melting, 10% more of Sm was added in excess of the stoichiometric amount. Then, they were melted three times in an arc furnace under high-purity argon. During the melting, the melting current should be suitably controlled in order to reduce the loss of Sm. 54 specimens were prepared and the mass loss of each sample was kept below 1%. The as-cast samples wrapped in Mo foil were sealed in silica tube filled with high-purity argon. Specimens were homogenized at different temperatures (Table 2) on the basis of the Sm-Fe and Nd-Fe binary phase diagrams [6-8] and then quenched in water.
Table 1 Crystallographic data of compounds in Sm-Fe and Nd-Fe binary systems
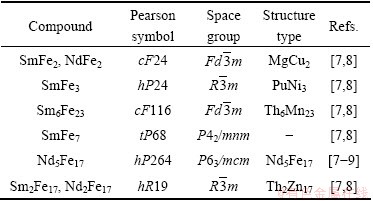
Table 2 Composition and heat treatment conditions of alloys in Sm-Nd-Fe system
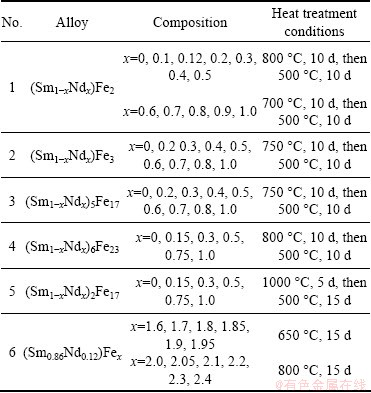
Characterization of the specimens was performed using optical microscopy. The etchant used was 2% nital. Differential thermal analysis (DTA) was carried out with heating and cooling rates of 10 K/min by using an LCP-1-type high temperature differential thermal dilatometer. The DTA furnace was evacuated with a pressure of 5×10-3 Pa and then kept under high-purity argon atmosphere in order to prevent the oxidation of samples in the alumina crucibles during the experiment. Pure Cu (99.99%) was used to check the temperature. X-ray diffraction analysis was carried out in a D/max-rA diffractometer equipped with a pyrolytic graphite monochromator. Cu Kα radiation was used. Software Jade 5.0 was used to analyze the XRD data of all samples. Electron probe microanalysis (EPMA) was performed in a Camebax-micro analyzer.
3 Results and discussion
3.1 Intermetallic phases
Metallographic examination, X-ray diffraction analysis, and EPMA confirm that there are four intermetallic phases: (Sm,Nd)Fe2, (Sm,Nd)Fe3, (Sm,Nd)5Fe17 and (Sm,Nd)2Fe17.
3.1.1 (Sm,Nd)Fe2
According to Sm-Fe and Nd-Fe systems, SmFe2 phase is stable, and the NdFe2 phase does not exist. Metallographic examination and X-ray diffraction analysis confirm that the structure of as-cast (Sm1-xNdx)Fe2 alloys consists of the majority of (Sm,Nd)Fe2 phase with MgCu2-type cubic structure when x≤0.55. Small amounts of phases are the (Sm,Nd)Fe3 and rare earth-rich phase when x≤0.4 and the (Sm,Nd)5Fe17 phase occurs when x=0.5 (Fig. 1).
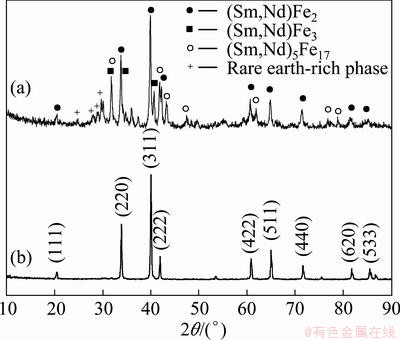
Fig. 1 X-ray diffraction patterns of as-cast (a) and annealed (b) (Sm0.5Nd0.5)Fe2 alloy
After being annealed, the (Sm1-xNdx)Fe2 alloys are the single (Sm,Nd)Fe2 phase when x≤0.5. As we know, the NdFe2 compound does not exist in the equilibrium phase diagram and our result shows that only after the sample was annealed at 800 °C for 10 d, the microstructure of (Sm0.5Nd0.5)Fe2 alloys becomes a single (Sm,Nd)Fe2 phase, as shown in Fig. 1(b). PINKERTON et al [10] reported that they were unsuccessful in forming the MgCu2-type structure in Sm0.5Nd0.5Fe2 ingots by annealing at 700 °C for 72 h. BABU et al [3] investigated the structure of Sm1-xNdxFe1.93 alloys and found that the single (Sm,Nd)Fe2 phase with the cubic Laves phase structure is at x=0.32 and a small quantity of the (Sm,Nd)Fe3 phase is observed for the x=0.36. The structural, magnetic, and magnetostrictive properties of Tb1-xNdx(Fe0.9B0.1)2 alloys were investigated in Ref. [11] and it was found that the alloys, homogenized at 700 °C for 7 d, consist predominantly of the cubic Laves phase with a MgCu2-type structure and a small amount of rare earth-rich phase when x≤0.55. Our result indicates that we have successfully obtained the single (Sm,Nd)Fe2 phase with 50% Nd by suitably annealing. When x>0.7, the main phase becomes (Sm,Nd)5Fe17 and rare earth-rich phase is a small amount of phase. The (Sm,Nd)5Fe17, (Sm,Nd)Fe2, and rare earth-rich phase coexist in the range of 0.52 phase for (Sm1-xNdx)Fe2 alloys is shown in Fig. 2 and it linearly increases with increasing Nd content up to x=0.5. The increase in the lattice parameter is attributed to the large size of Nd atoms (the radius of Nd atom is 0.182 nm, and that of Sm atom is 0.181 nm).
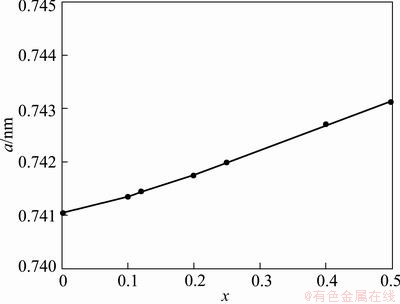
Fig. 2 Composition dependence of lattice parameter of (Sm,Nd)Fe2 phase for (Sm1-xNdx)Fe2 alloys
3.1.2 (Sm,Nd)Fe3
From the Sm-Fe binary system [7,9], the SmFe3 phase is stable. However, the NdFe3 phase does not exist in the Nd-Fe system. X-ray diffraction analysis confirms that the annealed (Sm1-xNdx)Fe3 alloys are a single (Sm,Nd)Fe3 phase with PuNi3-type structure when x<0.3. The structure of (Sm0.6Nd0.4)Fe3 alloy consists of (Sm,Nd)Fe2, (Sm,Nd)Fe3, and (Sm,Nd)5Fe17 phases. The (Sm,Nd)Fe3 phase disappears in the range of 0.5≤x≤ 0.6, the main phase is (Sm,Nd)5Fe17 and the small amount of second phase is (Sm,Nd)Fe2, as shown in Fig. 3. With increasing the Nd content further, a small amount of (Sm,Nd) phase appears when x=0.7. The structure of (Sm1-xNdx)Fe3 alloys consists of duplex phases in the range of 0.8≤x≤1.0, the main phase is (Sm,Nd)5Fe17 and the small amount of second phase is the rare earth-rich phase. This result indicates that the substitution of Nd for Sm makes the stability of (Sm,Nd)Fe3 phase decrease.
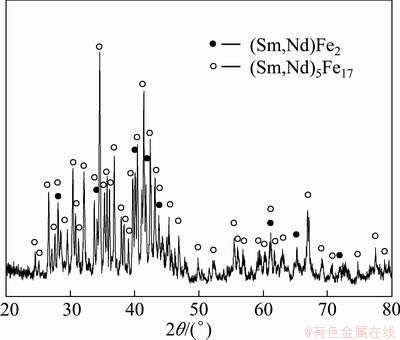
Fig. 3 X-ray diffraction pattern of annealed (Sm0.4Nd0.6)Fe3 alloy
3.1.3 (Sm,Nd)5Fe17
The Nd5Fe17 phase is stable only in the Nd–Fe binary system [9] and is of the hexagonal structure. The metallographic examination and X-ray diffraction analysis confirm that the structure of annealed (Sm1-xNdx)5Fe17 alloys consists of the (Sm,Nd)Fe3 phase and a small amount of (Sm,Nd)2Fe17 phase when x≤0.2. It was reported that the Sm5Fe17 phase cannot be formed in the Sm5Fe17 alloy ingot produced by induction melting [12]. By annealing of the amorphous melt-spun ribbon, the Sm5Fe17 phase has been synthesized and unlike the Nd5Fe17 phase, it is the metastable phase. When x=0.3, the (Sm,Nd)5Fe17 phase appears and the structure consists of the (Sm,Nd)Fe3, (Sm,Nd)5Fe17, and (Sm,Nd)2Fe17 phases. The (Sm,Nd)Fe3 and (Sm,Nd)2Fe17 phases disappear and the structure is a single (Sm,Nd)5Fe17 phase when x=0.4,. With further increasing the Nd content, the alloys keep a single (Sm,Nd)5Fe17 phase up to x=1.0.
3.1.4 (Sm,Nd)6Fe23
The Sm6Fe23 or Nd6Fe23 phase with the Th6Mn23-type structure does not exist in the corresponding system. The X-ray diffraction analysis, metallographic examination, and EPMA (Table 3) have shown that the microstructure of homogenized (Sm1-xNdx)6Fe23 alloys consists of (Sm,Nd)Fe3 and (Sm,Nd)2Fe17 phases when x<0.25. In the range of 0.25≤x ≤ 0.35, the (Sm,Nd)5Fe17 phase occurs in addition to (Sm,Nd)Fe3 and (Sm,Nd)2Fe17 phases. The structure of homogenized (Sm1-xNdx)6Fe23 alloys consists of (Sm,Nd)5Fe17 and (Sm,Nd)2Fe17 phases when x>0.4, and no (Sm1-xNdx)6Fe23 phase occurs in the alloys.
Table 3 EPMA data of Sm6Fe23, (Sm0.85Nd0.15)6Fe23, and (Sm0.7Nd0.3)6Fe23 alloys
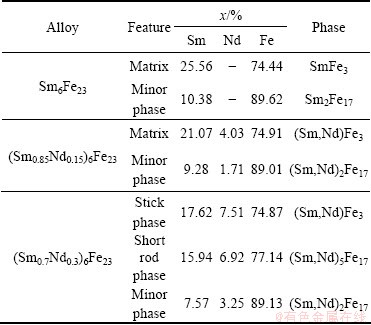
3.1.5 (Sm,Nd)2Fe17
According to the Sm-Fe and Nd-Fe systems, there are Sm2Fe17 and Nd2Fe17 phases. The Sm2Fe17 phase in annealed Sm2Fe17 alloy is stable and has a rhombohedral Th2Zn17 structure. When Nd is substituted for Sm, the miscibility of Sm and Nd of (Sm,Nd)2Fe17 phase in annealed (Sm1-xNdx)2Fe17 alloys is unlimited (Fig. 4). This result is in a good agreement with that reported in Ref. [13].
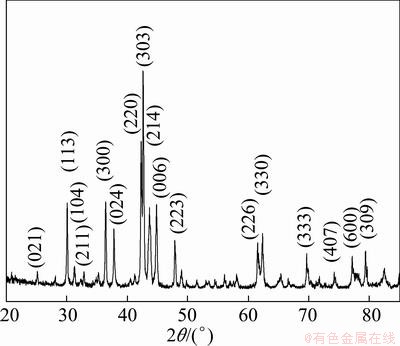
Fig. 4 X-ray diffraction pattern of (Sm0.6Nd0.4)2Fe17 alloy
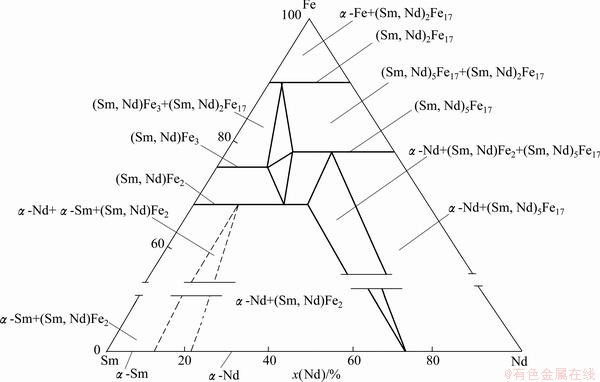
Fig. 5 Isothermal section of Sm-Nd-Fe ternary system at 500 °C
3.2 Isothermal section of 500 °C
The 500 °C isothermal section of the Sm-Nd-Fe ternary system is shown in Fig. 5. This isothermal section consists of 7 single-phase regions: α-Sm, α-Nd, (Sm,Nd)Fe2, (Sm,Nd)Fe3, (Sm,Nd)5Fe17, (Sm,Nd)2Fe17, and α-Fe; 8 two-phase regions: α-Sm+(Sm,Nd)Fe2, α-Nd+(Sm,Nd)Fe2, α-Nd+(Sm,Nd)5Fe17, (Sm,Nd)Fe2+ (Sm,Nd)Fe3, (Sm,Nd)Fe2+(Sm,Nd)5Fe17, (Sm,Nd)5Fe17+ (Sm,Nd)2Fe17, (Sm,Nd)Fe3+(Sm,Nd)2Fe17, and (Sm, Nd)2Fe17+α-Fe; 4 three phase regions: α-Nd+α-Sm+ (Sm,Nd)Fe2, α-Nd+(Sm,Nd)Fe2+(Sm,Nd)5Fe17, (Sm, Nd)Fe2+ (Sm,Nd)Fe3+(Sm,Nd)5Fe17, and (Sm,Nd)Fe3+(Sm,Nd)5Fe17+(Sm,Nd)2Fe17. The isothermal section of Sm-Nd-Fe ternary system contains (Sm,Nd)5Fe17 phase, and the homogeneity range of (Sm,Nd)Fe3 phase is less than that of (Sm,Nd)Fe2 phase.
3.3 Vertical section of SmFe2-NdFe2
The DTA curves for (Sm1-xNdx)Fe2 samples are shown in Fig. 6, and the peritectic reaction temperature and melting temperature can be determined. Based on the results of DTA, optical metallography and X-ray diffraction, a vertical section of the SmFe2-NdFe2 in Sm-Nd-Fe ternary system is tentatively drawn in Fig. 7. It consists of 2 single-phase regions: L and (Sm,Nd)Fe2; 4 two-phase regions: L+(Sm,Nd)Fe3, L+(Sm,Nd)5Fe17, L+(Sm,Nd)2Fe17, and α-Nd+(Sm,Nd)5Fe17; and 7 three- phase regions: L+(Sm,Nd)Fe3+(Sm,Nd)2Fe17, L+(Sm, Nd)5Fe17+(Sm,Nd)2Fe17, L+(Sm,Nd)Fe3+(Sm,Nd)5Fe17, L+(Sm,Nd)Fe2+(Sm,Nd)Fe3, L+(Sm,Nd)Fe2+(Sm, Nd)5Fe17, L+α-Nd+(Sm,Nd)5Fe17, and α-Nd+(Sm, Nd)Fe2+ (Sm,Nd)5Fe17.
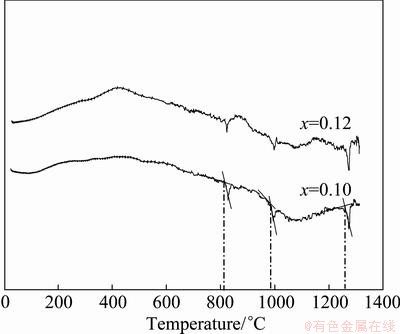
Fig. 6 DTA heating curves for (Sm1-xNdx)Fe2 samples
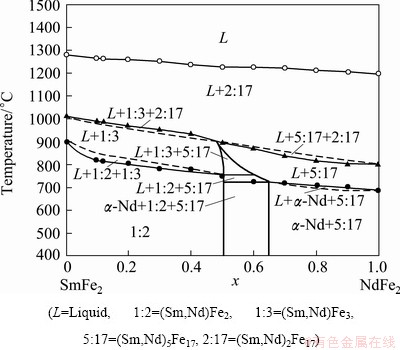
Fig. 7 Vertical section of SmFe2-NdFe2 in Sm-Nd-Fe ternary system
The peritectic temperatures of L+(Sm, Nd)Fe3→ (Sm,Nd)Fe2 for (Sm1-xNdx)Fe2 alloys decrease with increasing the Nd content when x≤0.5, which indicates that the substitution of Nd for Sm makes the stability of (Sm,Nd)Fe2 phase decrease. From Fig. 7, the temperature range between the reaction of L+(Sm,Nd)2Fe17→ (Sm,Nd)Fe3 and reaction of L+(Sm,Nd)Fe3→ (Sm,Nd)Fe2 becomes larger from x=0 to x=0.12, and it is difficult to prepare the orientation (Sm0.88Nd0.12)Fe2 sample. This result is consistent with that reported in Ref. [6].
3.4 Vertical section of (Sm0.88Nd0.12)Fex (1.6≤x≤2.4)
The vertical section of (Sm0.88Nd0.12)Fex (1.6≤x≤2.4) in the Sm-Nd-Fe system is shown in Fig. 8. It consists of 2 single-phase regions: L and (Sm,Nd)Fe2; 5 two-phase regions: L+(Sm,Nd)Fe2, L+(Sm,Nd)Fe3, L+(Sm,Nd)2Fe17, α-Sm+(Sm,Nd)Fe2, and (Sm,Nd)Fe2+ (Sm,Nd)Fe3; 2 three-phase regions: L+(Sm,Nd)Fe3+ (Sm,Nd)2Fe17, and L+(Sm,Nd)Fe2+(Sm,Nd)Fe3. According to the optical metallography and differential thermal analysis, the microstructure of (Sm0.88Nd0.12)Fe2 alloy is a single phase (Sm,Dy)Fe2 and its peritectic temperature is 815 °C.
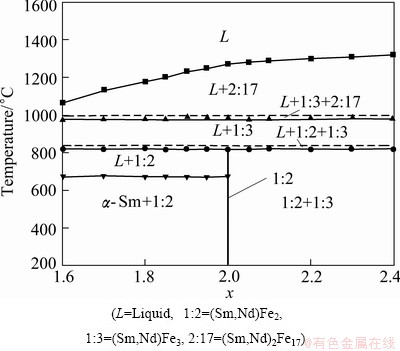
Fig. 8 Vertical section of Sm0.88Nd0.12Fex in range of 1.6≤x≤ 2.4 in Sm-Nd-Fe ternary system
4 Conclusions
The 500 °C isothermal section of the Sm-Nd-Fe ternary system consists of 7 single-phase regions, 8 two-phase regions and 4 three-phase regions. The complete miscibility of Sm and Nd is found for the (Sm,Nd)2Fe17 phase, whereas only limited Nd exists in (Sm,Nd)Fe2, (Sm,Nd)Fe3, and (Sm,Nd)5Fe17. No (Sm,Nd)6Fe23 phase occurs in the system. The vertical section of the SmFe2-NdFe2 in Sm-Nd-Fe ternary system consists of 2 single-phase regions, 4 two-phase regions and 7 three-phase regions. The peritectic temperature for (Sm1-xNdx)Fe2 alloys decreases with increasing the Nd content when x≤0.5. The temperature range between the reaction of L+(Sm,Nd)2Fe17→ (Sm,Nd)Fe3 and reaction of L+(Sm,Nd)Fe3→ (Sm,Nd)Fe2 becomes larger from x=0 to x=0.12, and it is difficult to prepare the orientation (Sm0.88Nd0.12)Fe2 sample.
References
[1] GUO Z J, ZHANG Z D, WANG B W, ZHAO X G. Giant magnetostriction and spin reorientation in quaternary (Sm0.9Pr0.1)(Fe1-xCox)2 [J]. Phys Rev B, 2000, 61(5): 3519-3523.
[2] WANG B W, LI W J, SONG J S, MIN B K. Structure, magnetic properties, and magnetostriction of (Sm0.5R0.5)(Fe1-xCox)2 compounds (R=Nd, Pr) [J]. J Appl Phys, 2002, 91(11): 9246-9250.
[3] BABU V H, MARKANDEYULU G, SUBRAHMANYAM A. Giant magnetostriction in Sm1-xNdxFe1.93 compounds [J]. Appl Phys Lett, 2007, 90: 252513-1-252513-3.
[4] LV X K, LIU W, YANG F, LI J, ZHANG Z D. Magnetic transitions and magnetostrictive properties of Laves compounds (Sm0.88Nd0.12)(Fe1-xCox)1.93 [J]. J Magn Magn Mater, 2010, 322: 3173-3177.
[5] YANG F, LIU W, LV X K, LI B, LI S Q, LI J, ZHANG Z D. Structural, magnetic properties and magnetostriction studies of Sm1-xNdxFe1.55 alloys [J]. J Magn Magn Mater, 2010, 322: 2095-2098.
[6] XIE J W, FORT D, ABELL J S. The preparation, microstructures and magnetostrictive properties of Samfenol-D [J]. J Alloys and Compounds, 2004, 366: 241-247.
[7] MASSALSKI T B, SUBRAMANIAN P R, OKAMOTO H, KACPRZAK L. Binary alloys phase diagrams [M]. Materials Park, OH: American Society for Metals, 1990.
[8] VILLARS P, CALVERT L D. Pearson’s handbook of crystallographic data for intermetallic phases [M]. Materials Park, OH: American Society for Metals, 1983.
[9] MARAZZA R, RIANI P, CACCIAMANI G. Critical assessment of iron binary systems with light rare earths La, Ce, Pr, and Nd [J]. Inorganica Chimica Acta, 2008, 361(14-15): 3800-2774.
[10] PINKERTON F E, HERBST J F, CAPEHART T W, MEYER M S, FELLBERG W A. Magnetostrictive Sm1-xNdxFe2/Fe composites from melt-spun precursors [J]. J Appl Phys, 1999, 85(3): 1654-1656.
[11] REN W J, OR S W, CHAN H L W, ZHAO X G, LIU J J, ZHANG Z D. Structural, magnetic, and magnetostrictive properties of Tb1-xNdx(Fe0.9B0.1)2 alloys [J]. IEEE Trans Magn, 2004, 40(4): 2772-2774.
[12] TETSUJI S, MASAKI I. Synthesis and magnetic properties of Sm5Fe17 hard magnetic phase [J]. Scripta Materialia, 2007, 57(6): 457-460.
[13] WANG Bo-wen, YAN Rong-ge, HAO Yan-ming, CAO Shu-ying, WENG Ling. Structural and magnetic properties of (Sm0.5Nd0.5)2(Fe1-xCox)17 compounds [J]. Rare Metals, 2003, 22(1): 55-59.
Sm-Nd-Fe三元系相图
王博文1,2,黄文美1,纪志超1,王志华1,孙 英1,翁 玲1
1. 河北工业大学 电磁场与电器可靠性省部共建重点实验室,天津 300130;
2. 中国科学院 国际材料物理中心,沈阳 110016
摘 要:采用金相显微镜、X射线衍射、电子探针和差热分析等技术确定了Sm-Nd-Fe三元系相图,包含Sm-Nd-Fe三元系500 °C等温截面、SmFe2-NdFe2变温截面和(Sm0.88Nd0.12)Fex(1.6 ≤ x ≤ 2.4)变温截面。Sm-Nd-Fe三元系含有4种金属间化合物:(Sm,Nd)Fe2,(Sm,Nd)Fe3,(Sm,Nd)5Fe17,(Sm,Nd)2Fe17,不含(Sm,Nd)6Fe23相。500 °C等温截面含有7个单相区,8个两相区,4个三相区。SmFe2-NdFe2变温截面含有2个单相区,4个两相区,7个三相区。(Sm0.88Nd0.12)Fe1.6-(Sm0.88Nd0.12)Fe2.4含有2个单相区,5个两相区,2个三相区。当x≤0.5时,(Sm1-xNdx)Fe2合金的包晶转变温度随着Nd含量的增加而降低,表明以Nd代替Sm从而使(Sm,Nd)Fe2相的稳定性降低。
关键词:Sm-Nd-Fe系;相图;结构;化合物
(Edited by Hua YANG)
Foundation item: Projects (50971056, 51171057) supported by the National Natural Science Foundation of China
Corresponding author: Bo-wen WANG; Tel: +86-22-60204363; Fax: +86-22-60204409; E-mail: bwwang@hebut.edu.cn
DOI: 10.1016/S1003-6326(13)62641-2