Trans. Nonferrous Met. Soc. China 24(2014) 250-256
Current efficiency of recycling aluminum from aluminum scraps by electrolysis
Jun-li XU1, Jing ZHANG2, Zhong-ning SHI3, Bing-liang GAO3, Zhao-wen WANG3, Xian-wei HU3
1. School of Science, Northeastern University, Shenyang 110819, China;
2. Huatian Engineering and Technology Corporation, MMC, Maanshan 243005, China;
3. School of Materials and Metallurgy, Northeastern University, Shenyang 110819, China
Received 19 November 2012; accepted 12 March 2013
Abstract: Extracting aluminum from aluminum alloys in AlCl3-NaCl molten salts was investigated. Al coating was deposited on the copper cathode by the method of direct current deposition using aluminum alloys as anode. The purity of the deposited aluminum is about 99.7% with the energy consumption of 3-9 kW·h per kg Al, and the current efficiency is 44%-64% when the deposition process is carried out under 100 mA/cm2 for 4 h at 170 °C. The effects of experimental parameters, such as molar ratio of AlCl3 to NaCl, cathodic current density and electrolysis time, on the current efficiency were studied. The molar ratio of AlCl3 to NaCl has little effect on the current efficiency, and the increase of deposition temperature is beneficial to the increase of current efficiency. However, the increase of current density or electrolysis time results in the decrease of current efficiency. The decrease of current efficiency is mainly related to the formation of dendritic or powder deposit of aluminum which is easy to fall into the electrolyte.
Key words: aluminum recycling; aluminum alloy; current efficiency; electrolysis; chlorides molten salts
1 Introduction
Due to the increased use of aluminum alloys, an increased amount of Al scrap has been generated. Recycling aluminum alloys is both cost effective and environmentally friendly. Today, aluminum scraps are mainly recycled by remelters, which are generally integrated into rolling mills and extrusion plants. It is not very efficient to recycle old scrap by simple re-melting of unsorted waste metal. Moreover, it is challenge for direct reusing recycled aluminum alloys in wrought alloys or in cast alloys since some elements are up-limited in the recycled aluminum alloys [1,2]. Sorting of aluminum alloys is a key factor for optimum recycling [3,4]. Even though recycling aluminum alloys is highly attractive from an economic point of view, the pure aluminum has to come from primary production for some high-tech applications today.
There are two methods currently in use for the production of high purity aluminum, viz. three-layer electrolysis process and segregation process. The three-layer process uses molten salt electrolysis to produce aluminum of greater than 99.99% purity. However, high temperature (700-900 °C) and an energy consumption of 17-18 kW·h per kg Al are needed for this process. Segregation process is a less energy- consuming process compared to the three-layer one but the purity of aluminum produced is usually not higher than 99.98%-99.99%. Additional refining steps are required in order to achieve high purity levels. Development of methods to further refine the recycled aluminum at low energy costs is needed.
R.G. Reddy group reported refining aluminum from aluminum alloys and aluminum metal matrix composites by electrolysis in ionic liquids. High purity aluminum (higher than 98%) deposits were obtained on copper or aluminum cathode [5-11]. The energy consumptions for recycling pure aluminum in their studies were 3-7 kW·h per kg Al [7,8,11]. The process has the advantage of low energy consumption compared to the existing industrial aluminum refining process.
Compared with ionic liquids, inorganic molten salts have higher electricity. Electroplating of aluminum or aluminum alloys has been widely investigated in alkali chloroaluminate melts. ROLLAND and MAMANTOU [12] and JAFARIAN et al [13] suggested that the electrodeposition of Al in alkali chloroaluminate melts proceeds via instantaneous nucleation followed by a growth controlled by hemispherical diffusion of ions to the nuclei. LI et al [14-16] suggested that the nucleation process was progressive in equimolar NaCl-AlCl melts and the reduction reaction was quasireversible. NAYAK and MISRA [17] reported that the cathodic current efficiency of the aluminum deposition process in AlCl3-NaCl molten salts approached 100% except when flakes were formed. WANG et al [18,19] found that the electrodeposits of Al were dense and well adherent to the Al substrate that were obtained between 50 and 100 mA/cm2. However, to the author’s knowledge, the recycling of aluminum from aluminum alloys scraps in alkali chloroaluminate melts has never been reported yet. In this study, the direct aluminum extraction process from aluminum alloys in AlCl3-NaCl molten salts was investigated, and the effects of the variation of current density, electrolyte composition and deposition time on current efficiency were studied.
2 Experimental
The electrolysis tests were performed in a 100 mL beaker fitted with a rubber cap. Aluminum alloys and Cu plates were used as anode and cathode. The aluminum alloy compositions are presented in Table 1. The electrodes surface was both 2 cm2, and the electrode interpolar distance was 3 cm. NaCl was dried at 200 °C for 5 h, and it was kept in a glove box with Ar atmosphere. AlCl3 (without water) was unsealed and kept in the glove box too. The electrolysis cell was assembled in the air condition.
Deposits obtained on the cathode plate were washed in water by ultrasonic and dried at room temperature. The deposit structures were examined by scanning electron microscopy (SEM) and the phases of the deposits were characterized by X-ray diffraction (XRD) using Cu Kα radiation. The impurity contents in the electrolyte were analyzed using inductively coupled plasma (ICP).
The current efficiency was evaluated through the determination of the electrodeposited mass, by comparison with the theoretical value.
η=△m/mm (1)
where △m is the actual mass of metal deposited; m is theoretical mass of metal deposited.
The actual mass of metal deposited (△m) was determined by the mass change observed in the cathode before and after electrolysis. The theoretical mass of metal (mm) was determined using the Faraday’s law.
mm=ItAm/(nF) (2)
where I is the applied current, t is the time, n is the number of electrons transferred in the elementary act of the electrode reaction, Am is the mole mass of the metal and F is the faraday constant.
The energy consumption (E) during the electrorefining was determined using
E=V/(0.3356η) (3)
where V is the cell voltage and η is the current efficiency.
3 Results and discussion
3.1 Characterization of electrodeposits
Commercial aluminum alloys (2024, 3004, 7075 type) plates were used as anode separately, and copper plate was used as cathode. The recycling processes were carried out at 170 °C under 100 mA/cm2 in NaCl-AlCl3 molten salts with a AlCl3 to NaCl mole ratio of 1.3. The process lasted for 4 h.
AlCl3-NaCl melts exhibit Lewis acid-base properties. When AlCl3 is mixed with NaCl, the ionic constituents of the resultant ionic liquid are determined by the mole ratio of AlCl3 to NaCl (q/p).
qAlCl3+pNaCl=qNaAlCl4+(p-q)NaCl, q/p<1 (4)
qAlCl3+pNaCl=(2p-q)NaAlCl4+(q-p)NaAl2Cl7,
1<q/p<2 (5)
is a strong Lewis acid, while
is a Lewis base. The melts with q/p>1 are acidic and those with q/p<1 are basic, whereas the melt with q/p=1 is neutral.
Table 1 Compositions of aluminium alloys

In our work, acidic melt was used to extract Al from Al alloy. Al alloy was used as anode, and Cu plate was used as cathode. As there are two aluminum-containing complexes (
and
) in acidic melt, the cathodic deposition of aluminum at the cathode is possible by discharge of either of these two aluminum- containing complexes.
+3e→ Al+
(6)
+3e→Al+4Cl- (7)
It was reported that the deposition reaction is due to the discharge of
anions in a basic melt, while it is the
anion which is reduced to give the aluminum deposit in an acidic melt [20,21]. Meanwhile, Al in the alloy anode dissolves into the melt.
Al(anode)→Al3++ 3e- (8)
Then, Al3+ reacts with
to form
.
Al3++7
→4
(9)
The deposits obtained on the cathode plate were stripped off from the substrate, and washed in water by ultrasonic and dried at the room temperature. XRD result shows that the deposit is aluminum as shown in Fig. 1. The impurity contents in the deposits are shown in Table 2, and the calculated current efficiencies and energy consumptions of these aluminium alloys by electrorefining are shown in Table 3.
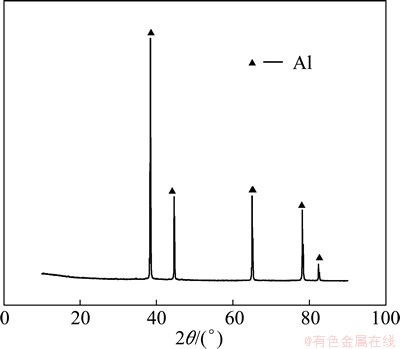
Fig. 1 XRD pattern of aluminum deposit
Table 2 Composition of deposited aluminum on copper cathode
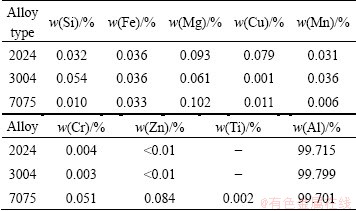
It can be seen in Table 2 that the purity of aluminum deposits is about 99.7%. The impurities are mainly Si, Mg and Fe, which come from the anode alloy. The energy consumption for aluminum recycling from aluminium alloy is only 3-9 kW·h per kg Al as shown in Table 3. It is lower than that of the aluminum production. However, the current efficiency is only 44%-64%. It is quite low compared with aluminum production process. The cause for the low current efficiency will be discussed in the following section.
Table 3 Current efficiency and energy consumption of aluminium alloys electrorefined at 170 °C, n(AlCl3)/n(NaCl)=1.3, current density 100 mA/cm2 for 4 h
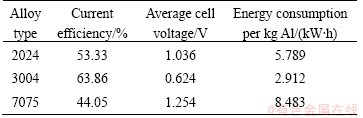
3.2 Effects of AlCl3 to NaCl ratio on current efficiency
The current efficiencies of the Al electrodeposition reactions were measured at various molar ratio of AlCl3 to NaCl and temperature. The electrodeposition process was carried out at 50 mA/cm2 for 0.5 h. The current efficiencies were evaluated through the determination of the deposits mass, by comparing with the theoretical values, and the results are shown in Fig. 2. It can be seen that the current efficiencies are 93%-96% under the conditions. The relative error of the current efficiencies is about 0.6%. From Fig. 2, we can see that AlCl3 to NaCl molar ratio has little effect on the current efficiency at the same temperature in 0.5 h electrolysis time. High current efficiencies indicate that there are no side reactions taking place during the deposition of aluminum on the cathode. The loss of current efficiency may be due to the chemical corrosion reaction between the deposited aluminium with the melt.
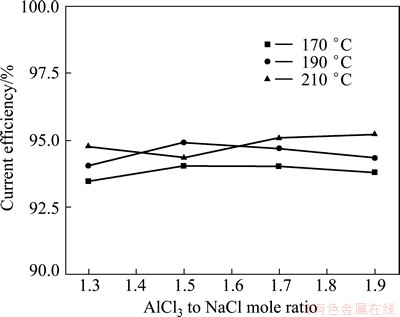
Fig. 2 Relationship between AlCl3 to NaCl molar ratio and current efficiency for aluminum deposits at current density of 50 mA/cm2 for 0.5 h
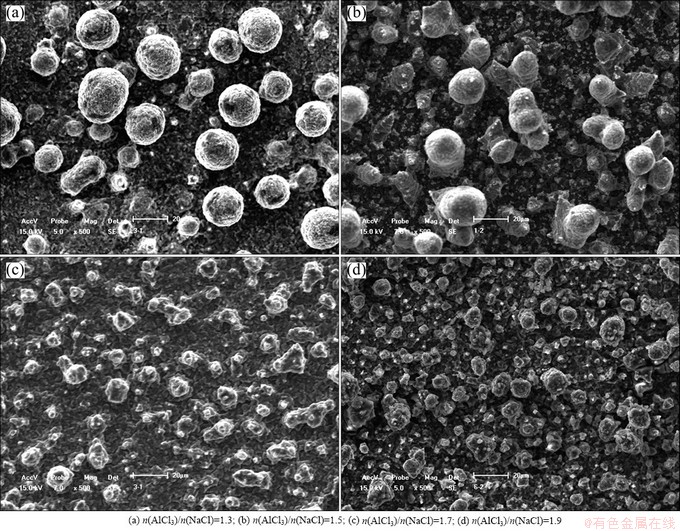
Fig. 3 SEM micrographs of aluminum deposits prepared on Cu electrodes in AlCl3-NaCl molten salts with different molar ratio at constant current density of 50 mA/cm2 and temperature of 170 °C for 0.5 h
The microstructures of the deposits obtained at 50 mA/cm2 with different n(AlCl3)/n(NaCl) are shown in Fig. 3. It can be seen that the morphology of the deposit is sensitive to the value of n(AlCl3)/n(NaCl). The grain becomes smaller and denser with the increase of n(AlCl3)/n(NaCl). It is in a close range of 15-20 μm when n(AlCl3)/n(NaCl) is 1.3 or 1.5, while it is in a close range of 5-10 μm when n(AlCl3)/n(NaCl) is 1.7 or 1.9. Moreover, all of the deposits present spherical structure. There is no dendritic structure formed under the conducted condition. This maybe can explain the close high current efficiency at the conducted condition for different n(AlCl3)/n(NaCl) values.
3.3 Effects of current density on current efficiency
Figure 4 shows the variation of current efficiency as a function of applied current density or temperature. It can be seen that cathodic current efficiency is 85%-96%. The current efficiency decreases with the increase of current density at a certain temperature.
The influence of current density on the current efficiency can be explained from the microstructure change of deposits with current density. Figure 5 shows the microstructure of the deposits obtained at different cathodic current densities. As seen in Fig. 5, the microstructures of deposits obtained at 25 and 50 mA/cm2 are spherical in the size varying from 5 μm to 10 μm, while it is dendritic when the applied current density is 75 mA/cm2. However, with the further increase of current density (100 mA/cm2), the microstructure is spherical again. Dendritic or powdery deposits are easy to fall off the electrode, which causes the decrease of current efficiency of the process.
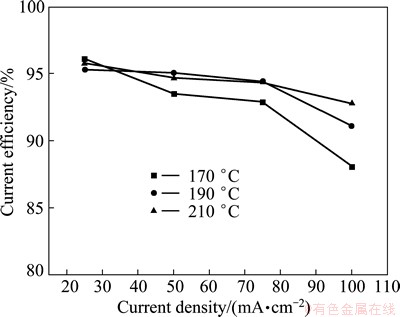
Fig. 4 Relationship between current density and current efficiency for aluminum deposits (n(AlCl3)/n(NaCl)=1.3; Electrolysis time 0.5 h)
3.4 Effects of electrolysis time on current efficiency
Figure 6 shows the relationship between current efficiency and electrolysis time at a n(AlCl3)/n(NaCl) of 1.3 and current density of 25 mA/cm2. It can be seen that the current efficiency decreases rapidly from 96% to 55% with the prolonging of electrolysis time.
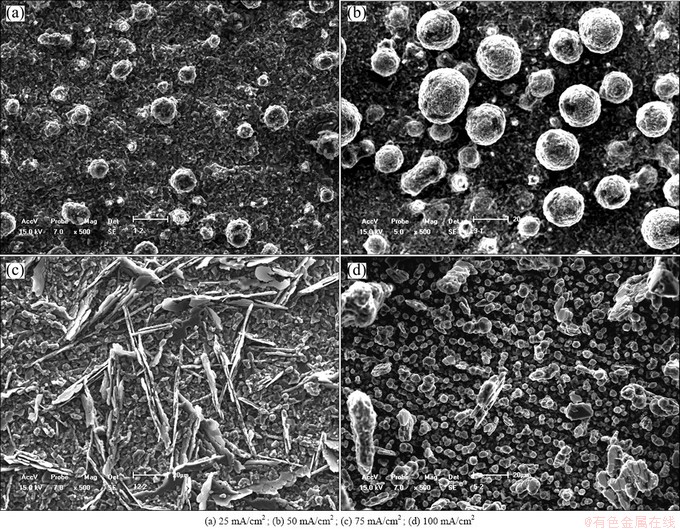
Fig. 5 SEM micrographs of aluminum deposits prepared on Cu electrode from AlCl3-NaCl molten salts with n(AlCl3)/n(NaCl) of 1.3 at 170 °C for 0.5 h at applied current densities
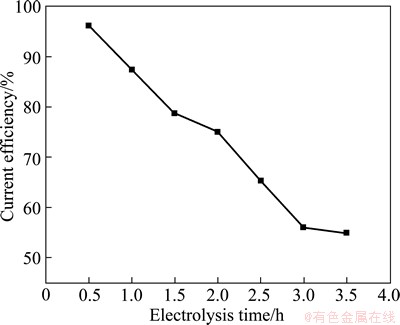
Fig. 6 Relationship between electrolytic time and current efficiency for aluminum deposits (25 mA/cm2, n(AlCl3)/ n(NaCl)=1.3, 170 °C)
Figure 7 shows the micrographs of the deposited aluminum obtained in different deposition time in AlCl3-NaCl molten salt with n(AlCl3)/n(NaCl) of 1.3 and current density of 25 mA/cm2 at 170 °C. It can be seen that the deposit has spherical structure in 1.5 h, and it turns to dendritic-like ones after prolonging the deposition time. As mentioned above, dendritic deposit is easy to fall off from the cathode, which causes the rapid decrease of current efficiency. This may be the main cause for the low current efficiency when the electrolysis process is carried out for 4 h (Table 2).
3.5 Effects of electrolysis temperature on current efficiency
As presented in Fig. 2 and Fig. 4, the current efficiency increases with the increase of temperature at a certain AlCl3 to NaCl ratio or current density. This is because the increase of temperature is beneficial to the transportation of anions in the solution, which will increase the reaction rate. However, as seen in Fig. 8, the increase of temperature accelerates the volatilization of electrolyte, which causes the instability of electro- deposition process. The volatile substance is AlCl3 detected by XRD, as shown in Fig. 9.
4 Conclusions
1) Aluminum was successfully extracted from aluminum alloy by electrolysis in AlCl3-NaCl molten salts. High purity aluminum (about 99.7%) deposits were obtained. The energy consumption was 3-9 kW·h per kg Al and the current efficiency is only 44%-64% when the deposition process was carried out under current density of 100 mA/cm2 for 4 h at 170 °C.
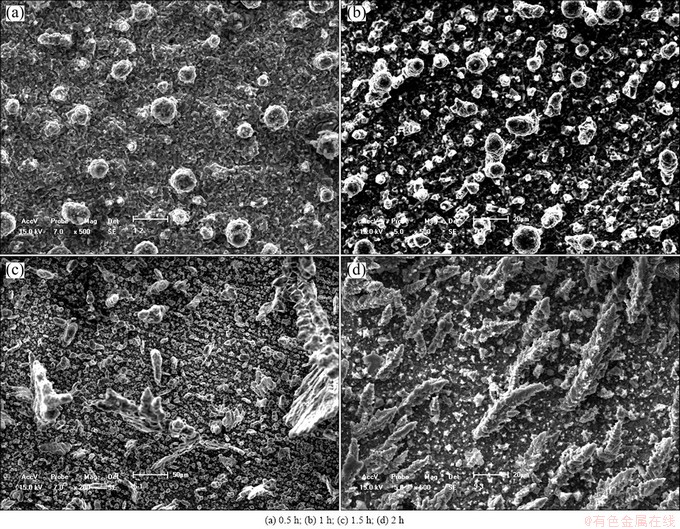
Fig. 7 SEM micrographs of aluminum deposits prepared on Cu electrode in AlCl3-NaCl molten salt with n(AlCl3)/n(NaCl) of 1.3 and current density of 25 mA/cm2 at 170 °C for different electrolysis time
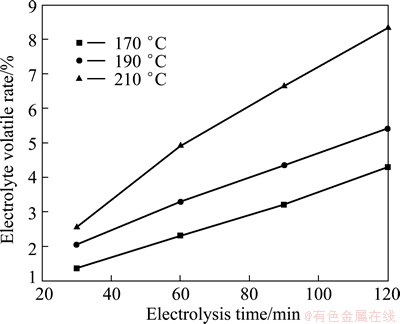
Fig. 8 Relationship between electrolysis time and volatile rate of electrolyte at various temperature when n(AlCl3)/n(NaCl) is 1.7
2) The molar ratio of AlCl3 to NaCl has little effect on the current efficiency, while the increase of deposition temperature is beneficial to the increase of current efficiency. However, the increase of current density or electrolysis time results in the decrease of current efficiency.
3) The dendritic microstructure resulting in the
falling of the deposit to the electrolyte is the main cause for the lower current efficiency.
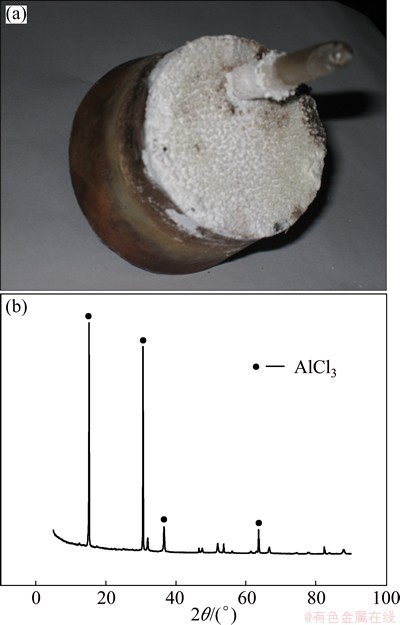
Fig. 9 Real photo (a) and XRD pattern (b) of electrolyte volatile on rubber cap surface
References
[1] HESS J B. Physical metallurgy of recycling wrought aluminum alloys [J]. Metallurgical Transactions A, 1983, 14: 323-327.
[2] CUI Ji-rang, ROVEN H J. Recycling of automotive aluminum [J]. Transactions of Nonferrous Metals Society of China, 2010, 20: 2057-2063.
[3] KEVORKIJAN V. The recycle of wrought aluminum alloys in Europe [J]. JOM, 2002, 54: 38-41.
[4] DAS S K. Designing aluminum alloys for a recycling friendly world [J]. Materials Science Forum, 2006, 519-521: 1239-1244.
[5] WU B, REDDY R G, RODEGERS R D. Aluminum reduction via near room temperature electrolysis in ionic liuqids [C]//The Fourth International Symposium on Recycling of Metals and Engineered Materials. Warrendale, PA: TMS, 2000: 845-856.
[6] REDDY R G. Emerging technologies in extraction and processing of metals [J]. Metallurgical and Materials Transactions B, 2003, 34: 137-152.
[7] KAMAVARAM V, MANTHA D, REDDY R G. Electrorefining of aluminum alloy in ionic liquids at low temperatures [J]. Journal of Mining and Metallurgy, 2003, 39: 43-58.
[8] KAMAVARAM V, MANTHA D, REDDY R G. Recycling of aluminum metal matrix composite using ionic liquids: Effect of process variables on current efficiency and deposit characteristics [J]. Electrochimica Acta, 2005, 50: 3286-3295.
[9] ZHANG M, KAMAVARAM V, REDDY R G. Ionic liquid metallurgy: Novel electrolytes for metals extraction and refining technology [J]. Minerals and Metallurgical Processing, 2006, 23: 177-186.
[10] PRADHAN D, MANTHA D, REDDY R G. The effect of electrode surface modification and cathode overpotential on deposit characteristics in aluminum electrorefining using EMIC-AlCl3 ionic liquid electrolyte [J]. Electrochimica Acta, 2009, 54: 6661-6667.
[11] PRADHAN D, REDDY R G. Dendrite-free aluminum electrodeposition from AlCl3-1-ethyl-3-methyl-imidazolium chloride ionic liquid electrolytes [J]. Metallurgical and Materials Transactions B, 2012, 43: 519-531.
[12] ROLLAND P, MAMANTOV G. Electrochemical reduction of Al2Cl7- ions in chloroaluminate molts [J]. J Electrochem Soc, 1976, 123: 1299-1303.
[13] JAFARIAN M, MAHJANI M G, GOBAL F, DANAEE I. Effect of potential on the early stage of nucleation and growth during aluminum electrocrystallization from molten salt (AlCl3-NaCl-KCl) [J]. J Electroanal Chem, 2006, 588: 190-196.
[14] LI Q F, HJULER H A, BERG R W, BJERRUM N J. Electrochemical deposition of aluminium from NaCl-AlCl3 melts [J]. J Electrochem Soc, 1990, 137: 593-597.
[15] LI Q F, HJULER H A, BERG R W, BJERRUM N J. Influence of substrates on the electrochemical deposition and dissolution of aluminum in NaAlCl4 melts [J]. J Electrochem Soc, 1991, 138: 763-766.
[16] LI Qing-feng, QIU Zhu-xian. Electrochemical deposition of aluminium from NaCl-AlCl3 molten salt system [J]. Rare Metal Materials and Engineering, 1995, 24(3): 59-63. (in Chinese)
[17] NAYAK B, MISRA M M. The electrodeposition of aluminum on brass from a molten aluminum chloride-sodium chloride bath [J]. J Appl Electrochem, 1977, 7: 45-50.
[18] WANG Zhao-wen, KAN Hong-min, SHI Zhong-ning, GAO Bing-liang, BAN Yun-gang, HU Xian-wei. Electrochemical deposition and nucleation of aluminum ontungsten in aluminum chloride-sodium chloride melts [J]. J Mater Sci Technol, 2008, 24: 915-920.
[19] KAN Hong-min, WANG Zhao-wen, WANG Xiao-yang, ZHANG Ning. Electrochemcial deposition of aluminum on W electrode from AlCl3-NaCl melts [J]. Transactions of Nonferrous Metals Society of China, 2010, 20: 158-164.
[20] STAFFORD G R, HAARBERG G M. The electrodeposition of Al-Nb alloys from chloroaluminate electrolytes [J]. Plasmas & Ions, 1999, 1: 35-44.
[21] LI Qing-feng, QIU Zhu-xian. Applied electrochemistry of aluminum—Production and applications [M]. Shenyang: Northeastern University Press, 2003: 138-144.
电解废旧铝合金回收铝的电流效率
徐君莉1,张 净2,石忠宁3, 高炳亮3,王兆文3, 胡宪伟3
1. 东北大学 理学院,沈阳 110819;
2. 中冶华天工程技术有限公司,马鞍山 243005;
3. 东北大学 材料冶金学院,沈阳 110819
摘 要:研究在 AlC13-NaCl熔融盐体系中进行电精炼废旧铝合金回收金属铝。以铝合金为阳极,通过直流电沉积在铜阴极上得到铝涂层。在170 °C、电流密度100 mA/cm2 下电解4 h,得到的沉积物铝的纯度大约为99.7%,电流效率为44%~64%,每千克铝消耗电能3~9 kW·h。探讨阴极电流密度、电解质成分和电解时间及温度等对铝沉积电流效率的影响。结果表明:在AlCl3和NaCl摩尔比为1.3~1.9时,AlCl3和NaCl摩尔比对电流效率的影响很小,升高电解温度有利于提高电流效率;但是延长电解时间或增大电流密度会导致电流效率降低。电流效率的降低主要是由于沉积的铝呈现枝状晶或粉化而易从阴极上脱落到电解质中所致。
关键词:铝回收;铝合金;电流效率;电解;氯化物熔盐
(Edited by Hua YANG)
Foundation item: Projects (51104042, 51074046) supported by the National Natural Science Foundation of China; Project (N120405006) supported by the Fundamental Research Funds for the Central University, China
Corresponding author: Zhong-ning SHI; Tel: +86-24-83686464; Fax: +86-24-83686464; E-mail: znshi@mail.neu.edu.cn
DOI: 10.1016/S1003-6326(14)63054-5