Trans. Nonferrous Met. Soc. China 20(2010) s798-s804

Mechanical properties of thixo-formed austempered ductile iron
B. HEIDARIAN, M. NLILI-AHMADABADI, M. MORADI
School of Metallurgy and Materials Engineering, University of Tehran, 14174 Tehran, Iran
Abstract: Semi-solid processing of metallic materials has been investigated for some time; however, this innovative production process has not fully established for ferrous alloys like ductile cast iron in the industry yet. Ferrous alloys-specially ductile cast iron-because of increasing complexity due to high temperature processing and some other limitations have been allocated a small share of this useful forming process to themselves. However, this work describes that thixo-forming of ductile iron despite all of the limitations is possible even for thin sections (less than 1 mm). Die temperature and injection velocity are the most effective parameters to thixo-forming particularly for high melting point materials such as ductile cast iron. Filling behavior, shape factor of graphites and fluidity of thixo-formed specimens were characterized. In addition, defects as well as shrinkage and cold laps were examined to achieve sound specimen. Subsequence to successful thixo-forming, two step austempering was performed to accomplish a uniform structure with minimum untransformed austenite volume (UAV). Following austempering, tensile test was carried out and the results show good combination of tensile strength and elongation for treated specimens (1 137 MPa strength and 12% elongation in best situation).
Key words: semi-solid; ductile iron; high pressure mold filling; segregation; mechanical properties
1 Introduction
Ductile irons because of spherical graphite have specific properties such as good mechanical properties, strength and toughness together as well as suitable castability. This engineering alloy along with growing applications has several shortcomings such as non-formability, dendritic structure, alloying element segregation, micro-porosity and production of thin section parts which have limited its applications. In addition, ductile iron can not be hot worked—like steel—to produce thin walled parts because of reduction of mechanical properties as a result of spheroidal graphite deformation[1].
Dendritic structure and micro-porosity are intrinsic properties for solidification of ductile irons. Therefore, semi-solid processing seems to be the proper solution to improve ductile iron microstructure and its mechanical properties without deformation in the graphite spheroids. It is worthwhile to note that graphite deformation for more than 15% decreases mechanical properties dramatically; 40% and more leads to decrease in mechanical properties by more than 50% because of stress concentration at sharp edges of deformed graphites[2-3]. Besides that, semi-solid processing of ductile iron offers the capability to produce near net shape and thin section parts.
Prior researches have reported semi-solid forming of gray cast iron[4-5]. In the case of ductile iron, the process is more complicated because of degeneration of graphite during conventional semi-solid processing (mechanical stirring) and deformation of graphite during thixo-forming. With the development of the inclined cooling plate technique, it is possible to prevent Mg fading in ductile iron, which may happen in conventional semi-solid processing[6-7]. Hence, this process offers better structural control and reduced complexity.
Having an alloy with suitable freezing range and low liquid fraction sensitivity are the most important parameters to semi-solid forming of the ductile irons. According to previous works, although hypoeutectic ductile iron has a long freezing range, it is not suitable for semi-solid forming due to the dissolution of graphite during re-heating[8]. This phenomenon was also observed in hypoeutectic gray iron[4-5]. Conversely, in hypereutectic alloy, graphite spheroids remain in the microstructure during reheating and after semi-solid forming.
Austempering treatment is proved as a most effective treatment to improve mechanical properties of cast irons. Therefore, the contribution of alloying elements to provide sufficient austemperability is very important. However, sever inhomogeneity caused by segregation of alloying elements (such as Mn and Mo) prohibits from highly alloying[9]. Segregation of alloying elements in the intercellular regions causes to reduce bainitic transforming temperature of high alloy regions and consequently to remain untransformed austenite volume (UAV) in the mentioned regions which results in reduction of toughness. In the case of high alloy ductile cast iron, a two step austempering treatment can be used for improvement in toughness. Two step austempering was conducted in both high and low temperature for achieving complete transformation of low and high alloy regions respectively and resultant microstructure included upper and lower bainite in the areas close to the graphites and in intercellular regions, respectively. This method is developed for UAV free austempering of high Mn ductile iron and a combination of good toughness and strength is expected after suitable two step austempering.
Following previous works[10-11],present work is an attempt to study thixo-forming of hypereutectic ductile iron alloyed with Mn and Mo. Special attention was paid to microstructure control and mechanical properties of the products.
2 Experimental
2.1 Semi-solid Casting
Ductile cast iron with the chemical composition shown in Table 1 was prepared in a medium frequency induction furnace. Magnesium was added by the sandwich method with 2.5% Fe-Si-Mg as inoculant and 0.4% Fe-Si as post inoculant.
Table 1 Feedstock chemical composition (mass fraction/%)

Hypereutectic ductile iron containing Mn and Mo produced by inclined cooling plate has been used for thixo-forming. Processing of semi-solid feedstock of ductile iron was carried out with inclined cooling plate method.
A water cooled copper plate with sizes of 1 000 mm×50 mm×20 mm served as an inclined cooling plate (Fig.1) coated with boron nitride. Important variables such as superheat of the melt, melt flow rate, and temperature, length and angle of the inclined cooling plate were selected according to the previous studies[7]. The slurry after flowing down from the inclined cooling plate collected in a sand mold.
2.2 Reheating process
Feed stock samples were reheated in a graphite tube in a resistance furnace under Ar atmosphere at 1 150 ?C for 40-45 min to achieve about 50% liquid fraction.
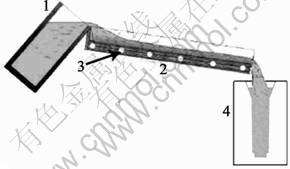
Fig.1 Schematic of inclined cooling plate process: 1-Pouring system; 2-Inclined cooling plate; 3-Water cooling system; 4-Mold
2.3 Semi-solid forming
Semi-solid forming was carried out at different temperatures (450, 500 and 600 ?C) in a step die having interchangeable gates with different V/A modules. The die material was quenched and tempered H13 tool steel coated with TiO2, shown in Figs. 2 and 3. This die with stepped sections was designed for the characterizations of the fluidity of ductile iron, influence of the wall thickness and the metal velocity on the filling behavior, phase segregation, and microstructure in the semi-solid state. Semi-solid slurry after passing through the gate entered the 30 mm step and was pushed to the thinner steps in the both side of the gate. A wedge part was included for studying flow behavior in longer length and subsequent tensile test.
Metal was introduced into the die cavity with a hydraulic press with maximum ram speed of 100 mm/s and 1.2×105 N force. At the final step of forming process, the same as high pressure die casting techniques; pressure was maintained for 5 s after injection in order to complete solidification.
2.4 Two-step Austempering
The specimens were initially austenitized at 920 ?C for 2 h, then austempered at 350 ?C and 270 ?C, for 50 min and 1-10 h respectively and then air cooled. Austempering was performed for all sections of stepped die and the treatment which resulted in the best microstructure was selected for tensile test specimens.
2.5 Microstructure analysis
Optical microscopy (ZEISS model) and image analyzer software (UTHSCSA image tool Ver. 1.27) used for microstructural study (calculation of graphite’s shape factor and liquid fraction). Shape factor of graphite particles were calculated according to Eq.1[12] and using images taken at 100 magnification. In this equations A and P are area and perimeter of solid particles respectively and N is the number of particles. Images were taken from the top, middle, bottom, left, center and right of each step after polishing.
(1)
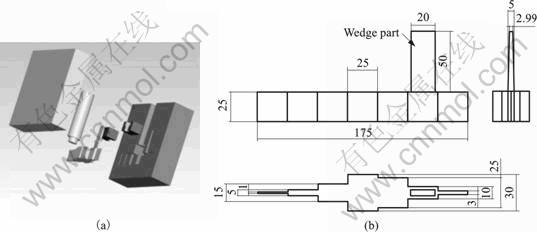
Fig. 2 Schematic of step die showing sections with different thicknesses used to assess fluidity (Unit: mm)
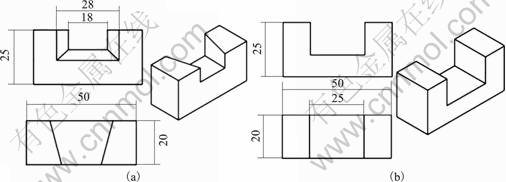
Fig. 3 Gate dimensions: (a) Gate No.1; (b) Gate No.2 (Unit: mm)
Polished samples were color etched with a solution of sodium hydroxide (NaOH), potassium hydroxide (KOH) and picric acid for liquid segregation study for thixo-formed specimens also 2% Nital was used for analysis of austempered specimen. Liquid fraction of thixo-formed and UAV of austempered specimens were investigated according to the images taken from the top, middle, bottom, left, center and right of each step.
3 Results and discussion
With gate No. 1 and 450 and 500 ?C die temperature, 1-10 mm steps are not filled and 15-30 mm steps are filled partially (Fig.4).
It is expected that solidification occurred in the gate and prevented complete filling. Low ram speed and consequent long filling time led to premature solidification. Filling time must be obviously less than solidification time. To this, end ram speed and die temperature should be increased.
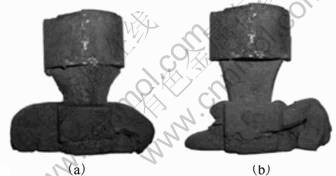
Fig.4 Thixo-formed samples with gate No.1 at different die temperatures: (a) 450 ?C; (b) 500 ?C
Inasmuch as incomplete filling is due to the short solidification time, the die temperature and gate modulus increased Fig.5 shows thixo-formed specimens at 600 ?C die temperature and gate No.2. The sample filled completely and all of the steps (30 to 1 mm) were filled without miss-run or visible defect. So, with increasing die temperature, solidification time increases and leads to prevention of early solidification and complete filling of the cavity.
Defects were seen in front of the gate at the bottom of 30 mm step (Fig.6). It is expected that non-globular particles and segregation of solid-liquid during reheating process are responsible for defect formations as a consequence of this inhomogeneity, segregated liquid solidified in the bottom of the part with dendritic microstructure. The interface of solidified liquid and semi-solid encourages crack and porosity formation. Fig.7 shows the microstructure of thixo-formed sample. Except for 30 mm step, all of the other steps are defect free and graphite remains spheroidal.
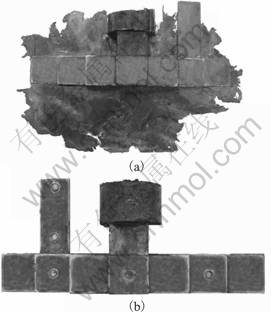
Fig.5 Thixo-formed samples with gate No.2 and die temperature 600 ?C before (a) and after trimming (b)
Fig. 8 shows un-etched microstructure and graphites shape of thixo-formed samples. Except for the 30 mm step, all of the other steps are defect free and graphite remains spheroidal without considerable changes in their shape.
According to Fig.5(a) and Fig.9, ductile iron shows good fluidity in the semi-solid state and has capability of filling to 1 mm thickness and less, such as flash and vents (Fig.9).
Table 2 shows that graphite shape-factor changes slightly in different steps, varying by less than 15%. This matter clearly indicates the advantages of thixo-forming in comparison to the other deformation processes of ductile iron. It must be considered that in 30, 25 and 15 mm thickness steps (under the gate); shape factor slightly decreases due to turbulence and lack of the liquid because of solid-liquid segregation.
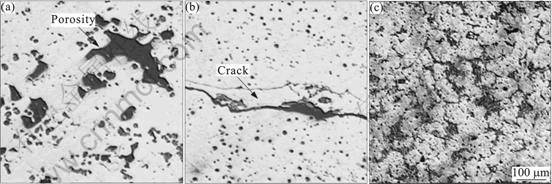
Fig.6 Formation of defects in 30 mm step with gate No.2 and die temperature 600 ?C: (a) Porosity; (b) Crack; (c) Dendritic solidification
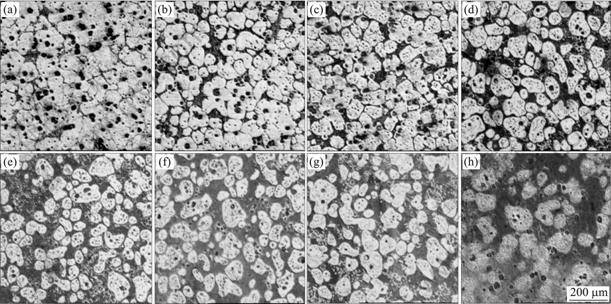
Fig.7 Microstructures of thixo-formed samples with gate No.2 and die temperature 600 ?C in different steps: (a) 30 mm; (b) 25 mm; (c) 15 mm; (d) 10 mm; (e) Wedge; (f) 5 mm; (g) 3 mm; (h) 1 mm
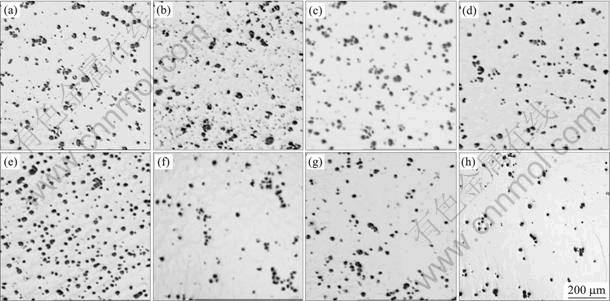
Fig.8 Microstructures of thixo-formed samples with gate No.2 and die temperature 600 ?C in different steps: (a) 30 mm; (b) 25 mm; (c) 15 mm; (d) 10 mm; (e) Wedge; (f) 5 mm; (g) 3 mm; (h) 1 mm
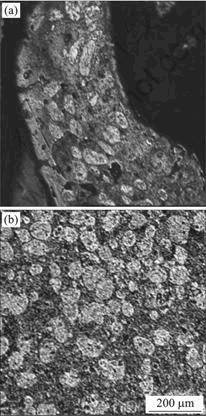
Fig.9 Microstructures of flash and vents with thickness below 1 mm: (a) Air-vent; (b) Flash
Table 2 Graphite shape-factor before and after thixo-forming
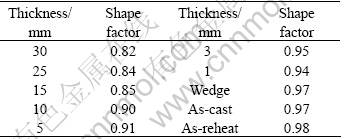
Results of austempering demonstrate that increase in the time of second step austempering leads to the increase in the fraction of lower bainite instead of UAV in the whole sections. They also illustrate that thick sections need longer time for the second step of austempring due to the longer solidification time and consequently more segregation of alloying elements. Figs.10 and 11 show the effect of second step austempering time and the effect of the thickness of specimens after 10 h austempering respectively.
Tensile test results (Fig.12) depict that all specimens have a good combination of strength and toughness properties. In addition, results show decrease in the austempering time causes to reduce the toughness due to incomplete austempering process. The best result (1 137 MPa strength and 12% elongation) pertains to prepared specimen from 3 and 10 mm steps.
The concluded results indicate that two-step austempering is a suitable heat treatment for thixo-formed ductile cast iron with heavy segregation. Also results confirm that thixo-formed and austemperd ductile iron can be used, even instead of forged carbon and low alloy steel in automotive parts.
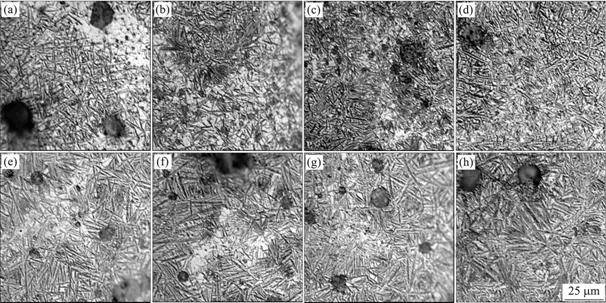
Fig.10 Effects of second step austempering time on fraction of UAVof 5 mm section (a)-(d) and 15 mm section (e)-(h):(a) 1 h;
(b) 2 h; (c) 3 h; (d) 4 h; (e) 4 h; (f) 6 h; (g) 8 h; (h) 10 h
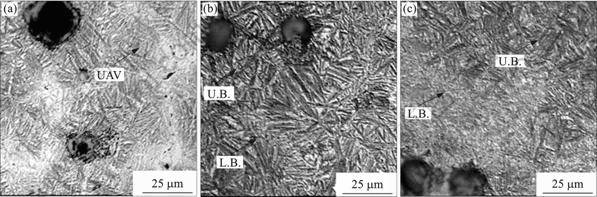
Fig.11 Effects of thickness on fraction of UAV after 10 h austempering: (a) 30 mm; (b) 15 mm; (c) 10 mm
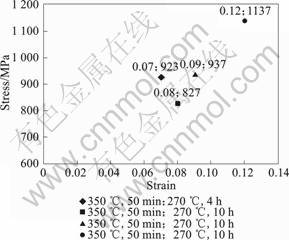
Fig.12 Tensile test results of thixo-formed and austempered specimens
More investigations can open window into usage of traditional casting material (ductile iron) as a new generation of tough and high strength materials.
4 Conclusions
1) Thixo-forming of ductile iron in thick and thin sections (less than 1 mm) was successfully developed.
2) Die temperature and injection velocity are the most effective parameters to thixo-forming particularly for high melting point materials such as ductile cast iron.
3) Ductile iron shows good fluidity in the semi-solid state.
4) Shape factor of graphite indicates a minor change (less than 15%) in thixo-forming of ductile iron.
5) Thick sections need longer time for second step of austempring due to the longer solidification time and consequently more segregation of alloying elements.
6) Two-step austempering is a suitable heat treatment for thixo-formed ductile cast iron with heavy segregation.
References
[1] LABRECQUE C, GAGNé M. Optimizing the mechanical properties of thin-wall ductile iron castings[J]. AFS Transactions, 2005, 113, 677-686.
[2] JI Cheng-chang, ZHU Shi-gen. Study of a new type ductile iron for rolling: composition design (1)[J]. Materials Science and Engineering A, 2006, 419: 318-325.
[3] ACHARY J. Tensile properties of austempered ductile iron under thermomechanical treatment[J]. Journal of Material Engineering and Performance, 2000, 9(1): 56-61 .
[4] POOLTHONG N, QUI P, NOMURA H. Primary particle movement and change of property of cast iron by centrifugal effect in semi-solid processing[J]. Science and Technology of Advanced Materials, 2003, 4: 481-489.
[5] TSUCHIYA M, UENO H, TAKAGI I. Research of semi solid casting of iron[J]. JSAE Review, 2003, 24: 205-214.
[6] FAN Z. Semisolid metal processing[J]. International Material Reviews, 2002, 47: 49-85.
[7] PAHLEVANI F, NILI-AHMADABADI M. Development of semi-solid ductile cast iron[J]. International Journal of Cast Metals Research, 2004, 17(3): 157-161.
[8] HEIDARIAN B. BSc thesis[D]. Tehran, Iran: School of Metallurgy and Materials Engineering, University of Tehran, 2007.
[9] NILI A M, NIYAMA E, OHIDE T. Bainitic transformation in austempered ductile iron with reference to untransformed austenite volume phenomenon[J]. AFS Trans, 1992, 94: 269-278.
[10] MORADI M, NILI-AHMADABADI M, HEIDARIAN B, ASHOURI S. Investigation of thin wall casting made of semi-solid A356 using back extrusion and die cast[J]. Solid State Phenomena, 2008, 141/143: 67-72
[11] MORADI M, NILI-AHMADABADI M, HEIDARIAN B, ASHOURI S. Defects Control and Mechanical Properties of Thixofromed Al-Si Alloy[J].Journal of Alloys and Compounds, 2009, 487: 768-775.
[12] SEO P K, KANG CG. The effect of raw material fabrication process on microstructural characteristics in reheating process for semi-solid forming[J]. Journal of Material Processing Technology, 2005, 162/163: 402-409.
(Edited by CHEN Ai-hua)
Corresponding author: M. NILE-AHMADABADI; Tel: +98-21-82084078; E-mail: nili@ut.ac.ir