J. Cent. South Univ. (2012) 19: 1946-1952
DOI: 10.1007/s11771-012-1230-z
Electrochemical treatment of wastewater containing chlorophenols using boron-doped diamond film electrodes
WANG Jian-gong(王建功)1, LI Xue-min(李学敏)2
1. Key Laboratory of Environmental Protection in Water Transport Engineering of Ministry of Communications
(Tianjin Research Institute of Water Transport Engineering), Tianjin 300456, China;
2. Department of Precision Instruments and Mechanology, Tsinghua University, Beijing 100084, China
? Central South University Press and Springer-Verlag Berlin Heidelberg 2012
Abstract: The electrochemical treatment of wastewater containing chlorophenols (2-monochlorophenol, 4-monochlorophenol, 2,4-dichlorophenol, 2,4,6-trichlorophenol) was carried out experimentally with synthetic boron-doped diamond (BDD) thin film electrodes. Current vs time curves under different cell voltages were measured. Removal rate of COD, instant current efficiency (ICE) and energy consumption were investigated under different current densities. The influence of supporting media is reported, which plays an important role in determining the global oxidation rate. The oxidative chloride is stronger than peroxodisulphate. The electrochemical characteristics of boron-doped diamond electrodes were investigated in comparison with active coating Ti substrate anode (ACT). The experimental results show that BDD is markedly superior to ACT due to its different absorption properties.
Key words: boron-doped diamond; electrochemical treatment; chlorophenol; wastewater
1 Introduction
Wastewater containing chlorinated phenols is highly toxic, and can hardly be treated with conventional microbiological technologies. Compounds in chlorinated phenols are suspected carcinogens and have activity as environmental endocrine disrupters [1]. It has been reported [2] that the toxicity of chlorophenols increases with the number of chloro-substituents but is lowered by the presence of chloro-substituents in the ortho-position. An increase in the number of chloro-substituents can also promote the accumulation of such chemical compounds in fish and other organisms [3], which increase their hazardous properties. Because many of these compounds are non-biodegradable, conventional microbiological technologies cannot be applied in treating them. Although chemical treatments such as wet air oxidation or incineration are effective, these technologies are expensive and, more importantly, not necessarily friendly to the environment. Thus, more efficient and environment-friendly alternatives are needed.
Electrochemical oxidation is one promising and powerful method that has the potential to replace or complete already existing processes [4-5]. This method makes the treatment of liquids, gases and solids possible. It is amenable to automation and compatible with the environment, given the fact that the main reagent, the electron, is a clean one [6].
A number of studies have been done on the electrochemical oxidation of chlorophenol aqueous wastes using different kinds of anodes [7-16]. Most of them have achieved the goal of the total mineralization of the organic compounds, confirming that electrochemical oxidation can be used to treat wastewater containing small amounts of organo- chlorinated aromatic compounds. However, electrochemical oxidation also depends on the type of anode employed. In addition to more traditional materials (DSA, SnO2, PbO2, etc), recently, a new kind of electrode based on boron-doped diamond thin films has been used. This type of electrode exhibits outstanding advantages over others, including good chemical resistance, wide electrochemical window and high efficiency [14, 17-19]. The characteristics of waste have a marked influence on the rate and efficiency of the electrochemical process because the organic compounds contained in the wastewater may have different degrees of oxidisability. Moreover, the pH of the waste greatly influences the current efficiency and, for aromatic compounds, the nature of reaction intermediates. In this sense, the supporting medium also significantly affects the treatment results, since it influences the nature of the mediated reagents electrogenerated at the anode surface [17-18]. In addition to the waste characteristics, operating conditions play an important role in the electrochemical oxidation of organic wastewaters [20-23].
The goal of the present work is to investigate the effect of electrochemical treatment of wastewater containing chlorophenols on boron-doped diamond electrodes. The chlorophenols studied were 4-monochlorophenol (MCP), 2,6-dichlorophenol (DCP) and 2,4,6-trichlorophenol (TCP). Degradation efficiency, energy consumption, removal rate of COD (chemical oxygen demand) and ICE (instant current efficiency) were investigated under different cell voltages. The removal rate of COD on boron-doped diamond electrodes was compared with that on active coating Ti (ACT) electrodes. In this work, ACT electrodes used has been succeeded in engineering practice for wastewater treatment from the ocean recover petroleum terminal station [24].
2 Material and methods
2.1 Diamond film preparation and characteristics
Boron-doped diamond (BDD) films were synthesized by the hot filament chemical vapor deposition technique (HFCVD) on single-crystal p-type Siá100? wafers. The temperature range of the filament was 2 440-2 560 °C, and the temperature of substrate was 830 °C. The reactive gas was methane in excess dihydrogen (1% CH4 in H2). The dopant gas was trinethylboron with a concentration of 3 mg/L. The gas mixture was supplied to reaction chamber at a flow rate of 5 L/min, giving a growth rate of 0.24 cm-1 for the diamond layer. The resulting diamond film thickness was about 1.25 cm. SEM image of the BDD films is shown in Fig. 1. Raman spectra of BDD films at different points in the same sample are presented in Fig. 2. It can be seen that this HFCVD process produces columnar, randomly-textured, and polycrystalline films.
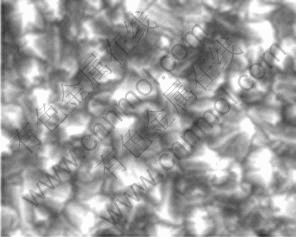
Fig. 1 SEM image of BDD film electrode
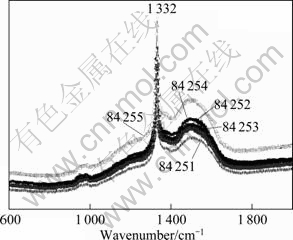
Fig. 2 Raman spectra of BDD film electrode (Different points at same sample)
The process of active coating Ti substrate electrode was sintered in muffle furnace. First, Ti substrate was boiled and etched for 3 h in the solution of oxalic acid with 10% mass fraction. Next, the special solution containing ruthenium, iridium and manganese and stannum compounds was sprayed on Ti substrate with brush. Then, Ti was parcheded under infrared light and sintered for 10 min at the temperature from 420 to 500 °C in furnace. The above steps were repeated until all the solutions were used. Finally, the sample was sintered for 1 h. SEM image of the ACT is shown in Fig. 3. There are a lot of cracks on the surface, resulting from sintering process. Likewise, it has strong absorbed properties due to its rough surface. Active composition in the ACT surface is made up of MnO2 4.49%, SnO2 3.95%, RuO2 33.17%, IrO2 58.40%, according to analysis, besides Ti substrate and TiO2.
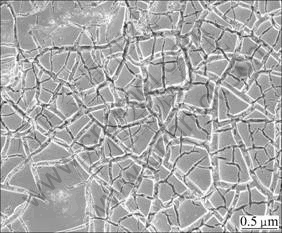
Fig. 3 SEM image of BDD film electrode
2.2 Apparatus
The electrochemical oxidation of chlorophenols was carried out in a single-compartment flow cell (Fig. 4). Anode and cathode are both BDD electrodes. Active coating Ti substrate electrodes were substituted for BDD in comparison with their result of electrochemical oxidation. Both electrodes were circular (100 mm in diameter), with a geometric area of 78.5 cm2 each and an electrode gap of 2 mm. The electrolyte was stored in two glass tanks (5 000 mL): one for treated solutions and the other for untreated solutions. Experiments were conducted under stationary regime and with flow allowing the solution to circulate through the cell with help of a peristaltic pump. The flow velocity could be ranged from 0 to 500 mL/min, and was regulated by the pump and measured by means of a calibrated flowmeter. The cell voltage and current were monitored with two sensors through an AD card in a computer.
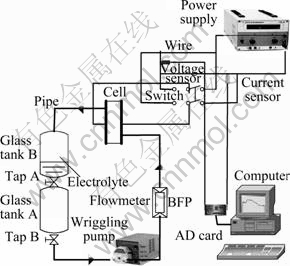
Fig. 4 Scheme of equipment used for bulk oxidation of chlorophenols on BDD electrodes
2.3 Experimental procedures
Galvanostatic electrolyses were conductive at chamber temperature, with an imposed current density range from 76 to 100 mA/cm2. COD was determined using a HACH DR200 analyzer. The concentration of chlorophenols in aqueous solutions during electrolysis was determined by a UV detector set at 270 nm.
All chemicals were of reagent grade. Distilled water was deionized in a millipore unit. The average composition of the wastewater used in the experimental assay was 10 mmol/L of chlorophenol (MCP, DCP and TCP) prepared with distilled water. The supporting electrolyte was 0.25 mol H2SO4. The concentration was chosen to provide adequate conductivity and buffer capacity without requiring excessive neutralization prior to the discharge of the product mixture.
In order to determine the influence of supporting media, an experiment using H2SO4 and NaCl media was carried out. The cell potential was constant during each electrolysis, indicating that neither appreciable deterioration of the electrode nor passivation phenomena took place. The electrolyte flow rate through the cell was 85 mL/min. The interval of sampling was 20 min. The curve of current was recorded by computer.
2.4 Determination of instantaneous current efficiency (ICE)
The ratio of the current effectively used in the electro-oxidation of organics (Ieff) at a given time (t) to the applied current (Iapp) is defined as the instantaneous current efficiency (ICE), and is given by the following relationship:
(1)
The chemical oxygen demand method was used for the determination of the current efficiency for the oxidation of several chlorophenols. In this method, the COD was measured during electrolysis and the instantaneous current efficiency was calculated using the relation:
(2)
where CODt and CODt+Δt are the chemical oxygen demand (g/L) at time t and t+Δt (s), respectively, I is the current intensity (A), F is the Faraday constant (96 487 C/mol), V is the volume of the electrolyte (L-1), 8 is a dimensional factor for unit consistence (32 g(O2)/mol (O2))/ (4 mol (e-1)/mol(O2)).
3 Results and discussion
3.1 Current – time curve
The experiment was conducted to determine the influence of electrolysis time on current intensity under different cell voltages. Figure 5 shows the trend of electrochemical oxidation current with time under cell voltages of 6, 6.5 and 7 V, respectively.
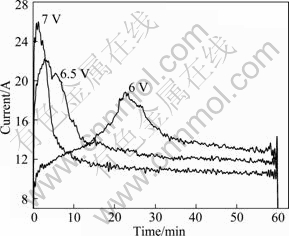
Fig. 5 Relationship between current and time for 2,6-dicholorphenol under different voltages
As shown in Fig. 5, the time to reach the peak of current under different cell voltage varies with voltages. At 6 V, it is 23 min. 6.5 V leads to 3 min. Only 1 min is needed at 7 V. The higher the cell voltage is, the shorter the time is to reach the highest value of current. Initial values of current under three cell voltages are 9.5, 17, and 24 A, respectively. The peak values of current are 18, 22, and 26 A, respectively. Current increases with cell voltage in a nonlinear way. This implies that a cell voltage of 6.5 V equals or exceeds the decomposition voltage of 2,6-dichlorophenol and accelerates the reaction rate of electrochemical oxidation.
3.2 Influence of wastewater characteristics and cell voltage
The galvanostatic electrolysis of MCP and DCP was compared under the same operation conditions in supporting media containing H2SO4 in water. Figure 6 shows the results. The removal rate is calculated by the concentration of pollutant measured by UV spectra (not shown here).
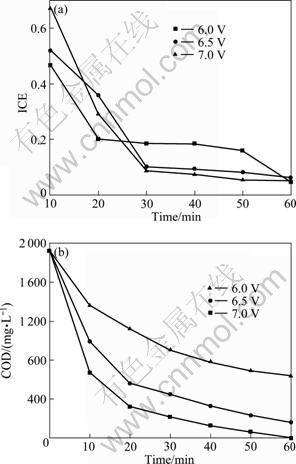
Fig. 6 Variation of ICE (a) and COD (b) with time in electrochemical oxidation of wastes containing DCP under different cell voltages
The oxidation rate and mass energy consumption increase with the increase of cell voltage according to UV spectra (Table 1 and 2). Similarly, ICE decreases with the cell voltage. The lower the cell voltage, the smaller the ICE is.
On one hand, it can be explained by the fact that in such condition, higher initial cell voltage can lead to higher initial current density and it can also increase the formation of indirect reagents like hypochlorite and peroxodisulphate. On the other hand, the formation of hypochlorite depends only on the organochlorinated compound but not on the presence of other redox reagents [25]. Its concentration increases with the chlorine content in the organic pollutant, so it also increases the rate of the oxidation processes. UV spectra also indicate that in all cases some intermediates are generated. Some of them, such as oxalic acid, maleic acid and fumaric acid, have been detected [25].
Table 1 Removal rate and energy consumption of MCP
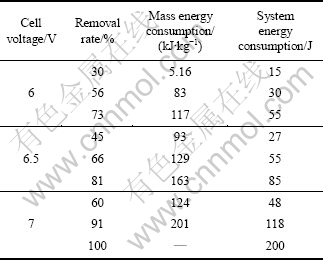
Table 2 Removal rate and energy consumption of DCP
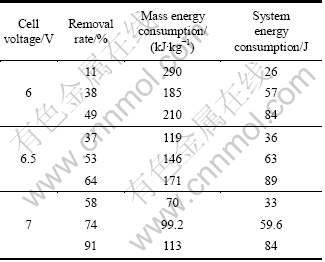
The higher initial rate observed in all cases can be explained by the higher initial concentration of hypochlorite, as can be observed in Fig. 7. Hypochlorite can act as a redox reagent and thus increases the global oxidation rate. In addition, ICE is associated with the concentration of pollutant. Low concentrations limit diffusion and the ICE values are correspondingly low. When high concentration wastes are treated, ICE can achieve values close to 1 at the beginning of the electrolysis before decreasing with time to finally reach a value not far from zero [25]. Therefore, the method is suitable for high concentration organic wastes.
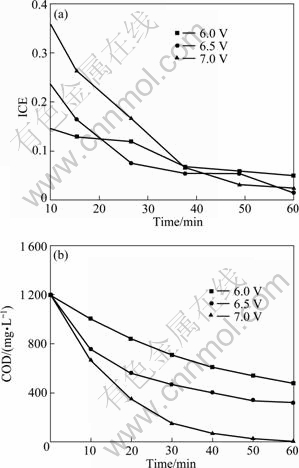
Fig. 7 Variation of ICE and COD with time in electrochemical oxidation of wastes containing MCP under different cell voltages
3.3 Influence of supporting media
The galvanostatic electrolysis of saturated 2,4,6-trichlorophenol was compared under the same operation conditions in supporting media containing H2SO4 and NaCl in water. The results are presented in Fig. 8 and Fig. 9.
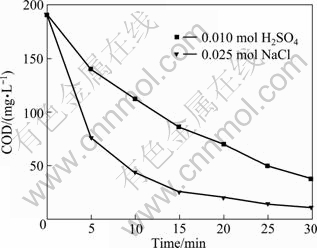
Fig. 8 Variation of COD vs time in electrochemical oxidation of wastes containing TCP on BDD electrodes
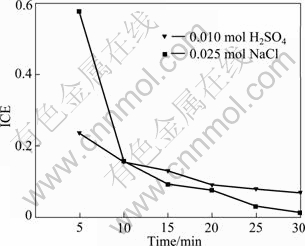
Fig. 9 Variation of ICE vs time in electrochemical oxidation of wastes containing TCP on BDD electrodes
The oxidation rate in media containing sodium chloride is higher than that in media vitriol. The ICE of TCP in media vitriol is in consistent with the one in sulphates [25]. The hypochlorite can be formed by means of the oxidation of the chloride ions released from the sodium chloride and chlorophenols [13, 26]. However, the concentration of hypochlorite from the organochlorinated compound is the same, though some electrogenerated reagents like peroxodisulphate can be formed in media vitriol, which can accelerate oxidation. The oxidation of hypochlorite is stronger than that of peroxodisulphate so that the initial value of ICE in sodium chloride is much higher than that in vitriol. The concentration of hypochlorite increases with the chloride content in media, so it also increases the oxidation rate. When time is beyond 10 min, the difference of ICE in two systems decreases with the decrease of COD and the ICE is even lower in sodium chloride than in vitriol. Thus, the presence of reagents (hypochlorite or peroxodisulphate), which can be oxidized at the anode surface, plays an important role in the global oxidation.
3.4 Influence of electrode materials
The galvanostatic electrolysis of the saturated ortho-chlorophenol in acid solution has been performed with ACT and BDD electrodes under different current densities (51-127 mA/cm2). The results of Figs. 10 and 11 show that COD reaches values independently of the electrode material and investigated condition. This indicates that the electro-combustion of 2-CP can be achieved at BDD anode and ACT anode.
However, the variation of ICE with time (Fig. 10(b) and Fig. 11(b)) suggests that the removal rate is influenced by electrode material and operating conditions like current density. Furthermore, with BDD electrodes, the removal rate and current efficiency are higher than those with ACT electrodes.
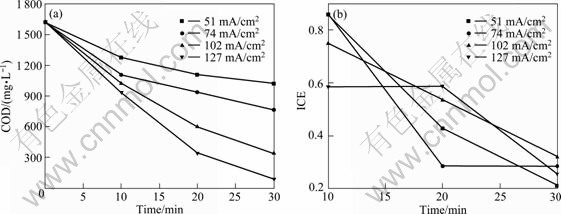
Fig. 10 Variation of COD and ICE vs time in electrochemical oxidation of wastes containing TCP on ACT electrodes (Electrolyte: 0.1 mol NaCl; Condition: c(2-CP) =0.01 mol/L; flow rate: 100 mL/min)
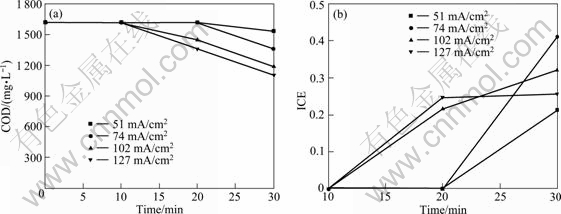
Fig. 11 Variation of COD and ICE vs time in electrochemical oxidation of wastes containing TCP on BDD electrodes (Electrolyte: 0.1 mol NaCl; Condition: c(2-CP) =0.01 mol/L; flow rate: 100 mL/min)
For the boron-doped diamond, the COD reaching very low value must be assured with certain current density. On the contrary, for the active coating Ti substrate anode, it is observed that COD is constant from 0 to 10 min under all current density during electrolysis, even from 10 to 20 under current density of 51 and 74 mA/cm2. However, the results of UV spectra (not shown here) demonstrate that red shift happened and new organic compounds appeared. This agrees with the results of GHERARDINI et al [15] and COMNINELLIS and NERINI [26]. This also shows that though the removal rate is low, the change of chemical structure of pollutant can improve wastewater biodegradation. This fact has been confirmed by the example of its engineering application [24].
Likewise, after electrolysis, the cell is disassembled and it is found that BDD has weak adsorption properties and ACT has strong adsorption properties comparatively. This is probably due to the fact that the oxygen overpotential of BDD is higher than that of ACT, in which the oxygen evolution is favored by the stronger adsorption of hydroxyl radicals than at BDD and consequently less reactive.
4 Conclusions
1) Both direct and indirect processes are involved in the oxidation of the organics. The indirect processes are mediated by oxidizing agents such as hypochlorite and peroxodisulphate, and these agents are generated on the surface of the anode.
2) The cell voltage plays an important role in the global oxidation rate. Higher cell voltages increase in the diffusion limitation. Especially, the ICE of initial stage can be significantly improved under higher cell voltages, largely due to an increase of energy consumption. However, high current densities can also increase the formation of indirect reagents, which consequently makes the oxidation rate increase and the efficiency decrease with applied current density.
3) Waste characteristics are found to have a strong influence on the development of the process. ICE of DCP removal is higher than that of MCP removal, which is related to their chemical structures. Supporting electrolyte can improve removal rate of COD and ICE. The oxidation of hypochlorite is stronger than that of peroxodisulphate.
4) The galvanostatic electrolysis of ortho- chlorophenol on synthetic boron-doped diamond thin film (BDD) and active coating Ti substrate (ACT) electrode under different current densities shows that the properties of BDD are superior to ACT due to the inert surface of BDD and the active surface of ACT. The adsorption on BDD is much weaker than ACT. In comparison with ACT, the electrochemical oxidation index (EOI) is much higher when BDD is used.
5) Intermediate organic compounds are generated at both ACT and BDD electrodes. These compounds can be used for pretreatment of biodegradation. BDD can completely replace ACT in some engineering.
References
[1] HO T L, BOLTON J R. Toxicity changes during the UV treatment of pentachlorophenol in dilute aqueous solution [J]. Water Research, 1998, 32 (2): 489-497.
[2] MARTIN T M, YOUNG D M. Prediction of the acute toxicity (96-h LC50) of organic compounds to the fathead minnow (pimephales promelas) using a group contribution method [J]. Chemical Research in Toxicology, 2001, 14 (10): 1378-1385.
[3] KISHINO T, KOBAYSHI K. Studies on the mechanism of toxicity of chlorophenols found in fish through quantitative structure-activity relationships [J]. Water Research, 1996, 30(2): 393-399.
[4] BRILLAS E, MUR E, SAULEDA R, SACHEZ L, PERAL J, DOMEECH X, CASADO J. Aniline mineralization by AOP’s: Anodic oxidation, photocatalysis, electro-Fenton and photoelectro- Fenton processes [J]. Applied Catalysis B: Environmental, 1998, 16(1): 31-42.
[5] BRILLAS E, CALPE J C, CASADO J. Mineralization of 2,4-D by advanced electrochemical oxidation processes [J]. Water Research, 2000, 34 (8): 2253-2262.
[6] CANIZARES P, DOMNGUEZ J A, RODRIGO M A, VILLASENOR J, RODRGUEZ J. Effect of the current intensity in the electrochemical oxidation of aqueous phenol wastes at an activated carbon and steel anode [J]. Industrial and Engineering Chemistry Research, 1999, 38 (10): 3779-3785.
[7] LERANG U, EBERT K, FLORY K, GALLA U, SCHMIEDER H. Organic waste destruction by indirect electrooxidation [J]. Separation Science and Technology, 1995, 30(7/9): 1883-1899.
[8] POLCARO A M, PALMAS S. Electrochemical oxidation of chlorophenols [J]. Industrial and Engineering Chemistry Research, 1997, 36(5): 1791-1798.
[9] POLCARO A M, PALMAS S, RENOLDI F, MASCIA M. On the performance of Ti/SnO2 and Ti/PbO2 anodes in electrochemical degradation of 2-chloropheno for wastewater treatment [J]. Journal of Applied Electrochemistry, 1999, 29(2): 147-151.
[10] GATRELL M, MACDOUGALL B. Anodic electrochemistry of pentachlorophenol [J]. Journal of the Electrochemical Society, 1999, 146 (9): 3335-3348.
[11] JOHNSON S K, HOUK L L, FENG J, HOUK R S, JOHNSON D C. Electrochemical Incineration of 4-chlorophenol and the identification of products and intermediates by mass spectrometry [J]. Environmental Science and Technology, 1999, 33(15): 2638-2644.
[12] RODGERS J D, JEDRAL W, BUNCE N J. Electrochemical oxidation of chlorinated phenols [J]. Environmental Science and Technology, 1999, 33(9): 1453-1457.
[13] AZZAM M O, AL-TARAZI M, TAHBOUB Y. Anodic destruction of 4-chlorophenol solution [J]. Journal of Hazardous Materials, 2000, 75(1): 99-113.
[14] RODRIGO M A, MICHAUD P A, DUO I, PANIZZA M, CERISOLA G, COMNINELLIS Ch. Oxidation of 4-chlorophenol at boron-doped diamond electrode for wastewater treatment [J]. Journal of the Electrochemical Society, 2001, 148(5): D60-D64.
[15] GHERARDINI L, MICHAUD P A, PANIZZA M, COMNIELLIS Ch, VATISTAS N. Electrochemical oxidation of 4-chlorophenol for wastewater treatment [J]. Journal of the Electrochemical Society, 2001, 148(5): D78-D82.
[16] URETA-ZANARTU M S, BUSTOS P, BERROS C, DIEZ M C, MORA M L, GUTIERREZ C. Electrooxidation of 2,4-dichlorophenol and other polychlorinated phenols at a glassy carbon electrode [J]. Electrochimica Acta, 2002, 47(15): 2399-2406.
[17] MICHAUD P A, MACHE E, HAENNI W, PERRET A, COMNINELLIS Ch. Preparation of peroxodisulfuric acid using boron-doped diamond thin film electrodes [J]. Electrochemical and Solid-State Letters, 2000, 3(2): 77-79.
[18] CANIZARES P, GARCA-GOMEZ J, LOBATO J, PRDROGO M A. Electrochemical oxidation of aqueous carboxylic acid wastes using diamond thin-film electrodes [J]. Industrial and Engineering Chemistry Research, 2003, 42(5): 956-962.
[19] PANIZZA M, MICHAUD P A, CERISOLA G, COMMINELLIS Ch. Anodic oxidation of 2-naphthol at boron-doped diamond electrodes [J]. Journal of the Electrochemical Society, 2001, 207(1/2): 206-214.
[20] KOTZ R, STUCKI S, CARCER B. Electrochemical waste water treatment using high overvoltage anodes. Part I: Physical and electrochemical properties of SnO2 anodes [J]. Journal of Applied Electrochemistry, 1991, 21(1): 14-20.
[21] GATTRELL M, KIRK D W. A study of electrode passivation during aqueous phenol electrolysis [J]. Journal of the Electrochemical Society, 1993, 140(4): 903-911.
[22] STUCKI S, KOTZ R, CARCER B, SUTER W. Electrochemical waste water treatment using high overvoltage anodes. Part II: Anode performance and applications [J]. Journal of Applied Electrochemistry, 1991, 21(2): 99-104.
[23] AWAD Y M, ABUZAID A. The influence of residence time on the anodic oxidation of phenol [J]. Separation and Purification Technology, 2000, 18(3): 227-236.
[24] LI Hai-tao, ZHU Qi-jia, ZU Rong. Electrooxidative degradation of waste water from the ocean’s recover petroleum terminal station [J]. Industrial water treatment, 2002, 22 (6): 23-25. (in Chinese)
[25] CNAIZARES P, CARCIA-GONEZ J, SAEX C, RODRIGO M A. Electrochemical oxidation of several chlorophenols on diamond electrodes: Part II. Influence of waste characteristics and operating conditions [J]. Journal of Applied Electrochemistry, 2004, 34(1): 87-94.
[26] COMNINELLIS C, NERINI A. Anodic oxidation of phenol in the presence of NaCl for wastewater treatment [J]. Journal of Applied Electrochemistry, 1995, 25(1): 23-28.
(Edited by YANG Bing)
Foundation item: Project(20113282241450) supported by the Science and Technology Program from Ministry of Transport of China
Received date: 2011-05-10; Accepted date: 2011-10-21
Corresponding author: WANG Jian-gong, Associate Professor; Tel: +86-22-59812345-306; E-mail: wangjgtks@126.com