
Factors governing heat affected zone during wire bonding
SUN Li-ning(孙立宁)1, LIU Yue-tao(刘曰涛)1, 2, LIU Yan-jie(刘延杰)1
1. State Key Laboratory of Robotics and System, Harbin Institute of Technology, Harbin 150080, China;
2. School of Mechanical Engineering, Shandong University of Technology, Zibo 255049, China
Received 10 June 2009; accepted 15 August 2009
Abstract: In the wire bonding process of microelectronic packaging, heat affect zone (HAZ) is an important factor governing the loop profile of bonding. The height of loop is affected by the length of the HAZ. Factors governing the HAZ were studied. To investigate this relationship, experiments were done for various sizes of wire and free air ball (FAB). Electric flame-off (EFO) current, EFO time, EFO gap and recrystallization were also studied. The results show that as the size of FAB becomes larger, the length of HAZ increases. With the increase of EFO current and time, the length of HAZ becomes longer. When FAB forms at the same parameter the length of HAZ becomes shorter with the high temperature of recrystallization.
Key words: heat affected zone; wire bonding; electric flame off; free air ball
1 Introduction
The wire bonding packaging process uses electric flame-off (EFO) discharge between two electrodes, causing a portion of one of them (the cylindrical wire anode) to melt, roll up into a ball, and later solidify. The purpose of wire bonding is to connect the chip with a lead frame, which is ultimately connected to the other external electrical components[1-2]. The most effective method of achieving this interconnection is the ball-edge bonding process that consists of ball bonding step at one end of the interconnection and wedge bonding step at the other end. A typical wire bonding cycle is shown in Fig.1. The bonding cycle commences with electrical breakdown of the air gap between wire and wand followed by the discharge that heats and melts the wire. Surface tension causes the melted part to roll up into a ball and the capillary presses the solidified ball onto the bond pad to make a ball bond through intermetallic compound formation between gold ball and aluminum pad. The gold-aluminum intermetallic compound is formed by applying ultrasonic and thermal energy to the interface. The ultrasonic energy is applied through the capillary mounted on a transducer. The thermal energy is applied through the heating block underneath the device. After the ball is bonded, the capillary rises to the loop height position while wire is fed out through the end of the capillary. The capillary next travels to the lead frame, forms a loop of wire between the chip and lead frame, and then squashes the wire against the frame to produce a wedge bond. After the wedge bond is made, the capillary rises and the wire is torn off from the bond. The length of wire between the two bonds is kept at a minimum so as to have the smallest possible chip profile as this length forms a loop from the bond pad on the chip to the lead frame. The height of this loop is affected by the length of heat affected zone (HAZ) along wire axis from the ball. Therefore, many researchers are focused on decreasing the length of HAZ because the length of HAZ has a direct relationship with the ball neck damage of wire loop[3-5].
Because the breakage sites of EFO wire are in the neck between the HAZ and the ball, it is clear that the structure of the neck zones and stress result in the breakage[6]. HUNG et al[7-8] studied the microstructure of the free air ball (FAB) after an EFO. CHANG et al[9] investigated the characteristic of HAZ on both Au wire and Cu wire. In this work, the mechanical and electrical properties of wire, and factors governing the length of
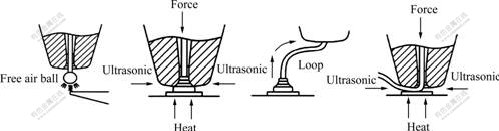
Fig.1 Schematic diagram of wire bonding process
HAZ were investigated.
2 Theory
The most important parameters governing the melting and resolidification of the gold wire are the wire diameter(d), the discharge current(I), the gap(G) between the electrodes, and the duration of discharge(t). In wire bonding process, the breakdown between wire anode and wand cathode typically occurs over very short time scales (≤1 μs) and small length scales (≤25 μm). COHEN and AYYASWAMY[10] performed a dimensional analysis to identify the principal dimensionless parameters governing such process.
The extent of the HAZ is determined by both the rate of heat transferred to the wire tip and the heat flux along the axis of the wire. The axial(one-dimensional) conduction, storage, and heat loss by convection and radiation from the surface of the wire segment during the discharge, are taken into account by a transient energy balance, of the form,
(1)
The initial condition is
(2)
The boundary conditions are
(3)
where T(z, t) is the temperature at any location z along the axis of the wire at time t; z is measured from the location at the end of the discharge and located closely to the neck between the ball and straight wire; ρ is the density of the gold wire; Cp is the variation ratio of enthalpy; α is the thermal diffusivity; k is the thermal conductivity; q″ is the heat flux at the wire/melt bottom.
The tip of the wire (ztip) is set to 0 initially and remains at 0 until melting begins. ztip then increases with time while the wire starts to roll up into the ball. As heat is applied to the bottom of the wire, the temperature of the wire will increase and melting will start at certain time. ztip, A(z) and P(z) are also functions of time. After each time step, the volume of the melt will be calculated, and ztip, A(z) and P(z) will be updated too.
3 Experimental
3.1 Wire fabrication
The process of wire fabrication is shown in Fig.2. The wires used in these experiments are fabricated in the production line of Kulicke & Soffa Corporation. According to the breaking load of wires, three types of wires are selected, which are FP2, AW14 and AW28. The characteristics of wires are shown in Table 1[11-13].
Table 1 Wire characteristics
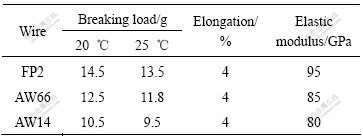
3.2 Machine and bonding conditions
The Kulicke & Soffa wire bonder 8020 is used to perform this experiment. The package type is 208-lead QFP. Bonding temperature is 220 ℃. The capillary is K&S 414FA-0310-R35. And the ball parameters include wire diameter, EFO current, EFO time, EFO gap and the tail extension[14-17]. The unchanged parameters are the EFO gap (30 μm) and tail extension (10 μm).
3.3 Etching procedure for viewing grain structure of HAZ
3.3.1 Mixing solution procedure
1) Add 4 g potassium iodide in a 50 mL beaker.
2) Add 2.54 g iodine.
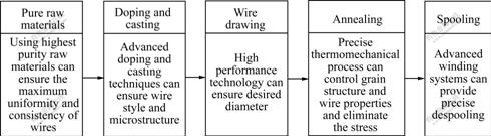
Fig.2 Wire fabrication process
3) Add 20 mL deionized water.
4) Mix and pour into a petri-dish.
3.3.2 Etching procedure
1) Place the wire with the FAB to be etched in a petri-dish.
2) Add the solution of potassium iodide and iodine to the petri-dish.
3) After 7 min of etching, take off the wire from petri-dish and submerge in water to remove iodine residue.
4) Dry the wire completely.
5) Place the wire in SEM and take photograph.
4 Results
4.1 FAB size with respect to HAZ
The relationship between the FAB size and HAZ is shown in Fig.3. As FAB size increases, the length of HAZ decreases. It is also observed that wire FP2 has shorter HAZ compared with the wire AW66 and AW14. Due to the larger heat input, the FAB size increases. From the measurement of microhardness at about 20 μm from the neck of the FAB, the microhardness decreases as the FAB size increases.
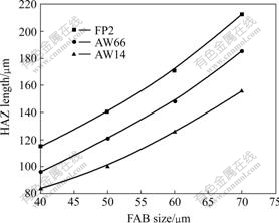
Fig.3 HAZ length of different wires as function of FAB size
4.2 EFO conditions with respect to HAZ
In this step, balls are formed from 25 μm diameter gold wire(FP2) on an automatic wire bonder (K&S 8020). Nine measurements are taken at each of three current settings (15, 30 and 45 mA) for several different EFO times. The variation of the EFO current level is ±0.5 mA, the variation of the EFO time is ±2 μs according to the specification of the EFO generation system. Measurement of the ball size is done with Hisomet, a measurement microscope with encoders and 400× magnifications. The results of the 9 measurements are listed in Table 2. From Table 2, it is clear that the wire has longer HAZ due to the larger current and time. Under the same looping motion, a shorter HAZ will result in a smaller bend radius and a lower loop. Thus, the loop shape can be greatly affected by the current and firing time. As explained above, a higher current will result in a shorter HAZ, therefore a lower loop.
Table 2 FAB sizes with respect to current and time
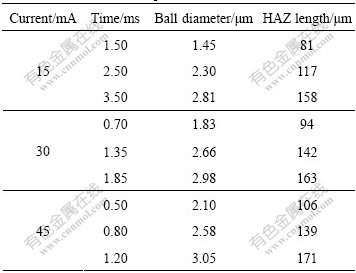
4.3 Wire diameter with respect to HAZ
As the wire size decreases, the fusing current reduces. For example, the melting current of gold wire with 1.0 μm in diameter and 5 mm in length is 0.56 A, and for gold wire with 0.6 μm in diameter, the current is 0.2 A. The fusing current is calculated by the following theoretical equation[18-19].
(4)
where If, tf, d and l are fusing current (A), fusing time (s), wire diameter (mm), and wire length (mm), respectively. Consequently, the reduction of the wire size causes the deterioration of electrical performance.
Fig.4 shows the HAZ length variation against the FAB size when FAB is two times larger than wire diameter. The thinner the wire is, the shorter the HAZ length is. The hard wire (FP2) including more Ca-dopants has high activation energy in the process of EFO and makes shorter HAZ length. HAZ length of hard wire is shorter than that of other wires for the wires with the same diameter.
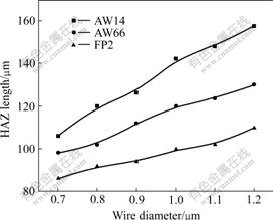
Fig.4 Relationship between HAZ length with wire diameter
4.4 Recrystallization temperature with respect to HAZ
DIETER [20] pointed out that among the variables that influence the recrystallization process (including prior deformation, temperature, time, initial grain size), the temperature plays a very important role. Doubling the annealing time is approximately equivalent to increasing the temperature of 10 ℃. Therefore, the relationship between the length of HAZ and the temperature during heating is obtained. The recrystallization temperature is the critical temperature at which the strain free grain is generated. In this experiment, AW66 wire is used. Through measuring the length of HAZ after EFO, the length is 120 μm at the recrystallization temperature of 200 ℃, and the length is 158 μm at the recrystallization temperature of 250 ℃. From the SEM images of longitudinal section of etched wire, the section is at a distance from the ball, which affects the extent of the HAZ. In the lower half of the section near the ball, the grains are equiaxed and the grain boundaries are well defined. In the upper region, the grains are less defined and etched darkly. These observations lead to a conclusion that the lower part of the section towards the ball consists of recrystallized grain while the upper region of the section corresponds to relatively unaffected region.
5 Conclusions
1) Comparison among the length of HAZ of the three types of wires at the same EFO time shows that FAB size influences the HAZ length. For the same wire, the HAZ length increases as the FAB size becomes larger. The larger FAB size needs more heat input.
2) The HAZ length shows a decreasing trend with increasing current. As the current increases, the arc duration required to form a ball decreases. This in turn implies that there is less time available for heat effect (e.g. grain recrystallization) to penetrate axially along the wire away from the ball. Due to the finite thermal diffusivity of the metal, the length of penetration of heat effects or the extent of HAZ also decrease with higher currents.
3) The heat-transferring section area of wire and the volume of FAB are proportional to the square and the cube of wire diameter, respectively. In order to form FAB with the constant ratio of wire diameter, the corresponding heat should be applied. Thus, the thinner the wire is, the shorter the HAZ length is.
4) The recrystallization temperature is inversely proportional to the HAZ length, because a high temperature means that the wire has to get hotter to recrystallize the grain structure. The EFO power affects the HAZ because of the energy and subsequent temperature at which the wire near the melted ball reaches.
References
[1] SAIKI H, MARUMO Y, NISHITAKE H, UEMURA T, YOTSUMOTI T. Deformation analysis of Au wire bonding[J]. Journal of Materials Processing Technology, 2006, 177(1/3): 709-712.
[2] LEE J, MAYER M, ZHOU N, PERSIC J. Microelectronic wire bonding with insulated Au wire: Effects of process parameters on insulation removal and crescent bonding[J]. Materials Transactions, 2008, 49(10): 2437-2353.
[3] JONGSIK L, SEONGMIN O, CHUNSEON P, YONGHO L, DAL A. A new wire bonding technique for high power package transistor[J]. Transactions of the Korean Institute of Electrical Engineers, 2008, 57(4): 653-659.
[4] ZHONG Z W. Wire bonding of low-k devices[J]. Microelectronics International, 2008, 25(3): 19-25.
[5] STURMANN H. Wire bonding process control[J]. Advanced Packaging, 2008, 17(1): 32-34.
[6] WULFF F, BREACH CD, DITTMER K. Crystallographic texture of drawn gold bonding wires using electron backscattered diffraction[J]. Journal of Materials Science Letters, 2007, 22(19): 1373-1376.
[7] HUNG Fei-yi, LUI Truan-sheng, CHEN Li-hui, LIN Yi-chang. Electric flame-off characteristics and fracture properties of 20 μm thin copper bonding wire[J]. Materials Transactions, 2009, 50(20): 293-298.
[8] HUNG Fei-yi, WANG Yuan-tin, CHEN Li-hui, LUI Truan-sheng. Recrystallization effect and electric flame-off characteristic of thin copper wire[J]. Materials Transactions, 2006, 47(7): 1776-1781.
[9] CHANG Wei-yao, HSU Hsiang-chen, FU Shen-li, YEH Chang-lin, LAI Yi-shao. Characteristic of heat affected zone for ultra thin gold wire/copper wire and advanced finite element wire bonding model[C]//The 10th Electronics Packaging Technology Conference. New York: Inst Elec Elec Eng Computer Society, 2008: 419-423.
[10] COHEN I M, AYYASWAMY P S. Ball formation processes in aluminum bonding wire[J]. Solid State Technology, 1985, 28(12): 89-92.
[11] Gold bonding wire for universal use-AW14 [R]. Singapore: Kulicke and Soffa, 2007.
[12] 4 N gold ball bonding wire for high reliability-AW66[R]. Singapore: Kulicke and Soffa, 2007.
[13] Gold bonding wire for enhanced reliability-FP2[R]. Singapore: Kulicke and Soffa, 2007.
[14] IWATA S, ISHIZAKA A, YAMAMOTO H. High-speed thermo- compression bonding of Au wires to Al electrode on semiconductor devices[J]. Japan Institute of Metals, 1977, 41(5): 1161-1165.
[15] DAWES C J. Ultrasonic ball wedge bonding of aluminum wires[J]. Electrocomponent Science and Technology, 1979, 7(1/3): 119-124.
[16] COHEN I M, HUANG L J, AYYASWAMY P S. Melting and solidification of thin wires: a class of phase-change problems with a mobile interface-Ⅱ. Experimental confirmation[J]. International Journal of Heat Mass Transfer, 1995, 38(9): 1647-1659.
[17] CHEN J L, LIN Y C. A new approach in free air ball formation process parameters analysis[J]. IEEE Transactions of Electronics Packaging Manufacturing, 2000, 23(2): 116-122.
[18] QIN W, COHEN I M. Ball size and HAZ as functions of EFO parameters for gold bonding wire[C]//Proceedings of the Pacific Rim/ASME International Intersociety Electronic and Photonic Packaging Conference. New York: American Society of Mechanical Engineers, 1997: 391-398.
[19] ATILA M M. Estimation of aluminum and gold bond wire fusing current and fusing time[J]. IEEE Transactions on components, packaging, and manufacturing technology, 1995, 18(1): 84-91.
[20] DIETER G E. Mechanical metallurgy[M]. New York: McGraw-Hill Book Company, 1986: 245-253.
(Edited by CHEN Wei-ping)
Foundation item: Project (50705027) supported by the National Natural Science Foundation of China; Project (2007AA04Z315) supported by the National High-Tech Research and Development Program of China
Corresponding author: LIU Yue-tao; Tel: +86-451-86414462-15; E-mail: yuetao.liu@gmail.com