
Fabrication and characterization of high dense Mo/Cu composites for
electronic packaging applications
CHEN Guo-qin(陈国钦), JIANG Long-tao(姜龙涛), WU Gao-hui(武高辉),
ZHU De-zhi(朱德志), XIU Zi-yang(修子扬)
School of Materials Science and Engineering, Harbin Institute of Technology, Harbin 150001, China
Received 15 July 2007; accepted 10 September 2007
Abstract: For electronic packaging applications,Mo/Cu composites with volume fractions of 55%-67% Mo were fabricated by the patented squeeze-casting technology. The microstructures and properties of the Mo/Cu composites were investigated. The results show that Mo particles are homogeneous and uniformly, and the Mo-Cu interfaces are clean and free-from interfacial reaction products and amorphous layers, the densifications of the Mo/Cu composites are higher than 99%. The mean linear coefficients of thermal expansion (20-100 ℃) of Mo/Cu composites range from 7.9 to 9.3×10-6/℃ and decrease with increasing volume fraction of Mo. The experimental coefficients of thermal expansion agree well with predicted values based on Kerner’s model. The thermal and electric conductivities of Mo/Cu composites are in range of 220-270 W/(m?℃) and 22-28 MS/m, respectively, and decrease with increasing volume fraction of Mo. The achievement of higher thermal and electric conduction is attributed to the full densities and high purity, which are obtained through the cost-effective squeeze-casting technology processes.
Key words: Mo/Cu composites; densification; thermal expansion coefficient; thermal conductivity; electric conductivity
1 Introduction
For applicability in the field of semiconductor, it is important that the thermal expansion coefficient of heat sink materials approximates to that of semiconductor chip and peripheral material. In addition, high thermal conductivity and densification also is required[1-3]. The Mo/Cu composites have excellent thermal conductivity, electric conductivity and tailorable coefficient of thermal expansion. Recently, they were developed rapidly on the large integrate circuit and high-power microwave apparatus as substrates, quadrats, joint parts and thermal dissipation components[4-6].
The property requests to the Mo/Cu composites become higher and higher as the electronic apparatus and products were developed to high-power, high performance and miniaturization, especially for a high densification (>98%) and a low gas content. Thereinto, the densification was the key factor to effect the thermal
and electric conductivity of materials[7-8]. And now, the Mo/Cu composites produced by conventional infiltration sintering and liquid-phase sintering method are hard to meeting the requests of high densification, while the chemical activated sintering technique and machine-alloying method can accelerate the course of densification and improve the degree of densification. But the addition of impurities such as active agent and the ball milling media has a serious effect on the thermal conductivity and electric conductivity of Mo/Cu composites[9-10]. In contrast to them, squeeze-casting method is believed to be an effective technique because of higher production rates, higher densification, elimination of activated agent and impurities, feasibility of mass production[11-12].
In this work, the Mo/Cu composites with volume fractions of 55%, 60% and 67% Mo were fabricated by the patented squeeze casting technology. Meanwhile, the microstructures and properties were discussed.
2 Experimental
The reinforcements used in this work were Mo spherical particles with nominal diameter of 3.5 μm, and the reinforcement volume fraction ranged from 55% to 67%. The copper matrix was commercially available pure copper w(Cu)≥99.7%, mass fraction). This pure copper was chosen for the purpose of high thermal conductivity and low cost.
The Mo/Cu composites were fabricated by squeeze casting technology[13], as illustrated in Fig.1. Firstly, the Mo particles were filled and pressed into a mold to produce a Mo preform according to the given volume fraction. After that, the preform was pre-heated in a die. At the same time, the copper was melt, degassed, cleaned in a graphite crucible and heated to 1 200-1 400 ℃. When the preform was heated to 900-1 100 ℃, the molten copper was poured into the die and a vertical pressure up to 30 MPa was applied immediately to force molten copper to infiltrate into Mo preform completely. The pressure was maintained about 5 min until the solidification was complete. Then the composites were annealed in vacuum at 700 ℃ for 1.5 h and furnace cooled to release the residual stress within the composites.
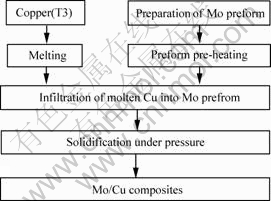
Fig.1 Fabrication process of Mo/Cu composites
An S-570 scanning electron microscope (SEM) was used to examine the microstructure of as-fabricated Mo/Cu composites. The measured density was obtained using the buoyancy (Archimedes) method and compared with the theoretical density to obtain various degree of densification. The CTE was measured on a DIL 402C (NETZSCH Corp.) with a heating rate of 5 ℃/min. Thermal conductivity was measured by the laser flash method with the NETZSCH LFA427 thermal constant measuring equipment. The testing sample was cylindrical, 12.7 mm in diameter and 3 mm in thickness. Electric conductivity was measured by vortex method with the Forster Sigmatest 2.068 electric conductivity measuring equipment. The testing sample was machined into several blocks of 40 mm×40 mm×2 mm.
3 Results and discussion
3.1 Densification
The effect of Mo volume fraction on densification for Mo/Cu composites is shown in Fig.2. The densification of the Mo/Cu composites decreases with increasing volume fraction of Mo under the same processing conditions. The densification of three composites are in range of 99.2%-99.5%, which can completely meet the high dense requests for electronic package materials.
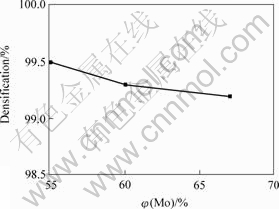
Fig.2 Dependence of volume fraction of Mo on densification for Mo/Cu composites
3.2 Microstructure observation
Fig.3 shows the SEM micrographs of the polished Mo/Cu composites produced by the squeeze-casting technology. It is found that the Mo/Cu composite is all dense and homogenous compound structures of adhesive phase Cu linking Mo grains. A dense microstructure is beneficial to electronic packaging applications because of the improvement in mechanical strength and thermal conductivity.
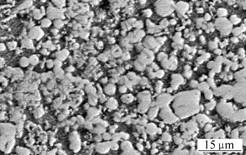
Fig.3 Microstructure of Mo/Cu composite
The interface and the existing of interface effect are the important factors which can affect the properties of composite. Fig.4 illustrates the typical TEM micrographs of the interfaces in Mo/Cu composites. A large mount of observations indicate that the Mo-Cu interfaces are clean, smooth and free-from interfacial reaction products and amorphous layers, dissolved Mo particles were not observed.
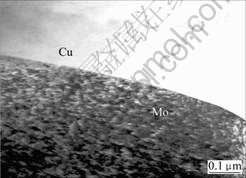
Fig.4 TEM micrographs of Mo-Cu interfaces
3.3 Thermal expansion analysis
The measured CTEs of Mo/Cu composites are 7.9, 8.6 and 9.3×10-6/℃ for the 67%, 60% and 55% composites, respectively. The CTEs were reduced with an increase in volume fraction of Mo. In a Mo/Cu composite, the thermal expansion behavior is influenced by the thermal expansion of copper matrix and the tightened restriction of Mo particles. Several theoretical models were proposed to predict the CTE of particulate composite[14-17]. If the matrix modulus is much smaller than that of reinforcement, the CTE of a composite is expressed as rule-of-mixture (ROM):
(1)
where α is the CTE, φ is the volume fraction, and subscripts c, m and p refer to the composite, matrix and particle, respectively.
Turner’s model considers the uniform hydrostatic stresses and gives the CTE of a composite as:
(2)
where K is the bulk modulus.
Both the normal and shear stress are taken into account in Kerner’s model, and the CTE of a composite is expressed as:
(3)
where G is shear modulus.
Fig.5 shows the comparison between above theoretical predictions and experimental data. As could be seen, experimental data were in good agreement with the predicted values based on Kerner’s model, but deviated from ROM and Turner’s model. This may be attributed to the fact that normal and shear stress were included in Kerner’s model, and ROM or Turner’s model could not describe the complicated internal stresses inside a composite.
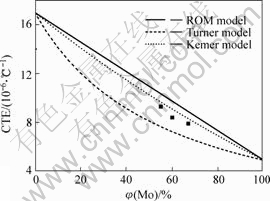
Fig.5 Comparison between theoretical predictions and experimental CTEs
3.4 Thermal and electric conductivity
The measured thermal and electric conductivities of Mo/Cu composites are shown in Fig.6. The thermal conductivity and electric conductivity of Mo/Cu composites decrease with increasing Mo content. Because the thermal conductivity and electric conductivity of Mo are lower so far than those of copper, and with increasing Mo content, the interfaces within the composites increases as well as the interface thermal and electric resistance.
As shown in Fig.6, one of the largest advantages of the Mo/Cu composites produced by patent squeeze- casting technology is that the thermal conductivities are all higher than 220 W/(m·℃), which are good enough to satisfy high thermal conductivity for electronic packaging application. Although the thermal conductivity of PMMCs is mainly decided by the thermal conductivity and content fraction of constituent components, it connects with the densification of materials, interface condition, the figures and distribution of particles[18-20]. So, the achievement of higher thermal and electric conduction was attributed to the full densities, and the Mo-Cu interfaces are clean, smooth and free-from interfacial reaction products and amorphous layers, which were attained through the cost-effective squeeze-casting technology processes.
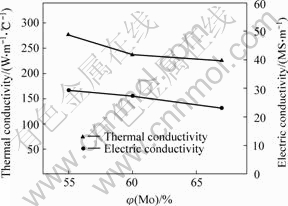
Fig.6 Variations of thermal conductivity and electrical conductivity with Mo particle volume fraction
4 Conclusions
1) The Mo/Cu composites reinforced with 55%- 67% Mo particles were produced by squeeze casting process, the composites are full densities and porosity-free macroscopically. The Mo-Cu interfaces are clean, smooth and free-from interfacial reaction products and amorphous layers.
2) The linear CTEs of Mo/Cu composites range from 7.9 to 9.3×10-6/℃, depending on the volume fraction of Mo. The experimental CTEs agree well with the predicted values based on Kerner’s model.
3) The composites have higher thermal and electric conduction. It is attributed to the full densities and high purity Mo/Cu composites, which were obtained through the patent cost-effective squeeze-casting technology.
References
[1] Shammas N Y A. Present problems of power module packaging technology[J]. Microelectronics Reliability, 2003, 43(4): 519-527.
[2] Zweben C. Advances in composite materials for thermal management in electronic packaging[J]. JOM, 1998, 50(6): 47-51.
[3] Lostetter A B, Barlow F, Elshabini A. An overview to integrated power module design for high power electronics packaging[J]. Microelectron Reliab, 2000, 40(3): 365-379.
[4] Johnson J L. Powder metallurgy processing of Mo-Cu for thermal management applications[J]. International Journal of Powder Metallurgy, 1999, 35(8): 39-48.
[5] Ali Z A, Drury O B, Cunningham M F. Fabrication of Mo/Cu multilayer and bilayer transition edge sensors[J]. IEEE Transactions on Applied Superconductivity, 2005, 15(2): 52-69.
[6] Hocine R, Boudghene A, Saidane A. A three-dimensional TLM simulation method for thermal effect in high power insulated gate bipolar transistors[J]. Microelectron Eng, 2003, 65(3): 293-306.
[7] Aguilar C, Ordonez S, Marin J. Study and methods of analysis of mechanically alloyed Cu–Mo powders[J]. Mater Sci Eng A, 2007, 464(1/2): 288-294.
[8] Kirk T W, Caldwell S G, Oakes J J. Mo-Cu composites for electronic packaging applications[J]. Advances in Powder Metallurgy, 1992, 9: 115-122.
[9] Arikawa T, Ichida A, Takeqoshi E. Mechanical properties of Cu-Mo composites[J]. Journal of the Society of Materials Science, 1999, 48(3): 295-300. (in Japanese)
[10] Yih P, Chung D D L. Brass-matrix silicon carbide whisker composites prepared by powder metallurgy[J]. Journal of Materials Science, 1999, 34: 359-364.
[11] Zweben C. High performance thermal management materials[J]. Electronic Cooling Magazine, 1999, 5(3): 36-42.
[12] Gopalakrishnan M V, Metzgar K, Rosetta D.Structural characterization and strength evaluation of spray formed ceramic composite near-net shapes[J]. J Mater Process Technol, 2003, 135(2): 228-234.
[13] WU Gao-hui, CHEN Guo-qin. A high dense Mo-Cu composite and fabrication method: CN, 200410028822.7[P]. (in Chinese)
[14] Geiger L, Jackson M. Low-expansion MMCs boost avionics[J]. Advanced Materials & Process, 1989, 136(7): 23-28.
[15] Turner P S. Thermal expansion stresses in reinforced plastics[J]. Journal of Research of the National Bureau of Standards, 1946, 37: 239-243.
[16] Kerner E H. The elastic and thermo-elastic properties of composite media[C]// Proceedings of the Physical Society, 1956, 69: 808-814.
[17] Hwang K S, Yang S C, Wang W.S. Mo-Cu heat sinks made by co-sintering and infiltration techniques[J]. Advances in Powder Metallurgy and Particulate Materials, 1995,3(1): 251-257.
[18] Hasselman D P H, Lloyd F J. Effective Thermal conductivity of composites with interfacial thermal barrier resistance[J]. Journal of Composites, 1987, 21(6): 508-515.
[19] TAO H J, XIE Y Q, PENG H J. Electronic structures and physical properties of FCC and metastable liquid Cu metals[J]. Journal of Central South University: Science and Technology, 2007, 38(1): 1-8. (in Chinese)
[20] LI Y P, QU X H, ZHANG Z S. Manufacture of high-dispersed W-Cu composite powder by mechano-thermal process[J]. Journal of Central South University, 2003, 10(3): 168-173.
Foundation item: Project(2003AA305110) supported by the National Hi-tech Research and Development Program of China; Project(2003AA5CG041) supported by the Key-Tech Research and Development Program of Harbin Province, China
Corresponding author: CHEN Guo-qin; Tel: +86-451-86402372-5058; E-mail: chenguoqin@hit.edu.cn
(Edited by YNAG Hua)