
Molecular structures and activity of organic depressants for marmatite, jamesonite and pyrite flotation
CHEN Jian-hua(陈建华)1, LI Yu-qiong (李玉琼)2, LONG Qiu-rong(龙秋容)3
1. College of Resources and Metallurgy, Guangxi University, Nanning 530004, China;
2. College of Chemistry and Chemical Engineering, Guangxi University, Nanning 530004, China;
3. Design and Research Institute of Mining and Metallurgy, Zijin Mining Group Limited Company, Longyan 364200, China
Received 8 September 2009; accepted 20 January 2010
Abstract: Ten kinds of organic depressants were used to investigate the depressing performance on marmatite and pyrite. Flotation results show that the organic compounds only with single group of hydroxyl (—OH), carboxyl (—COOH) or amino (—NH2) in molecule are ineffective in depressing marmatite, jamesonite and pyrite. The combinations of these functional groups still cannot enhance the depressing ability of organic depressant. The thioglycollic acid containing reductive functional group (—SH) has a good depressing performance for marmatite and pyrite. The presence of benzene ring in molecule can enhance the depressing performance. The functional group electronegativity, hydrophilic-hydrophobic indexes and frontier orbital of organic depressants were calculated, and the criterion for the depressing effect of organic depressants to sulphide minerals was proposed.
Key words: marmatite; pyrite; organic depressant; frontier orbital
1 Introduction
In the flotation separation of sulphide minerals, besides the traditional inorganic depressants (such as lime, NaCN, ZnSO4, Na2S and K2Cr2O4), organic depressants have been attracted great attention due to their various types, rich sources and relatively cheap price. Many sulphide minerals can be separated by organic depressants. For example, dextrin can be used as the depressant for pyrite[1]. The use of both the sodium polyacrylate 3223 and the inorganic dispersant sodium hexametaphosphate increased significantly the zinc recovery in lead-zinc sulfide ore flotation[2]. At different pH conditions, the flotation separation of jamesonite, marmatite and pyrrhotite can be achieved by using organic depressants with small molecular mercapto organic depressant[3]. The organic depressant DMPS[4] containing —SH and —SO3- has a good depressing effect on pyrrhotite, but can hardly depress jamesonite. PALA with functional groups of —COO- and O=C—NH2[5] has a selective depressing effect on arsenopyrite but not on marmatite after being activated by Cu2+. Using butyl-xanthate as collector, marmatite can be activated by Cu2+ in the presence of glyceryl-xanthate[6],while pyrite is depressed. The effects of small molecular organic depressants on depressing jamesonite and marmatite were studied[7].
The hydrophilic-hydrophobic index of organic depressants proposed by WANG[8] can explain well the structures and activity of organic depressants in the flotation of nonmetallic ore, whereas some studies indicate that the depressing activity of organic depressants for sulfide minerals is not only related to the hydrophilic-hydrophobic index, but also related to their electrochemistry property[9-10]. In order to further study the relationship between molecular structure and depressing activity of sulfide organic depressants, in this work, the effect of ten kinds of organic depressants with different molecular structures and functional groups on the marmatite and pyrite is tested.
2 Experimental
2.1 Materials
Pure samples of marmatite and pyrite were obtained from Dachang Mine in Guangxi Province, China. Marmatite sample has a purity of 93.4%, and the hand-picked pyrite sample has a purity of 95.1%. The minerals with a size range of <0.074 mm selected for flotation tests were sealed in glass bottle.
2.2 Flotation tests
The flotation tests on single mineral were carried out in a suspended style flotator with a capacity of 60 mL. In each test, 2.0 g of sample was used. The sample was cleaned by the supersonic apparatus for 8 min before flotation test. During flotation, the reagents were added in order. The organic depressant was first added with agitating time of 3 min; and then butyl-xanthate was added with a concentration of 10-4 mol/L and agitating time of 2 min; lastly, 2# oil was added with a consumption of 40 g/t and agitating time of 1 min. The total flotation time was 5 min. In addition, CuSO4 was used as the activator of marmatite, and the activating time was 4 min.
2.3 Computational methods
The frontier orbital calculations were performed within the framework of DFT[11] using the program DMol3[12-13]; the generalized gradient approximation (GGA)[14] with PW91[15] exchange correlation functional was used. Effective core potentials that replaced core electrons by a single effective potential were used in core treatment, and the DNP basis set was used within a fine quality whose self-consistent field tolerance is 1.0×10-6 eV/atom. The marmatite crystal model was constructed by substitution of iron for
zinc in the sphalerite crystal[16]. Fig.1 shows the computational models of unit cells of marmatite, jamesonite and pyrite. The molecules were constructed in the program. All the structures were optimized before analysis.
3 Results and discussion
3.1 Flotation test results and effects of functional groups
Ten organic depressants with different functional groups and molecular structures were used to investigate the effect on depressing marmatite and pyrite in the flotation tests. The results are shown in Figs.2, 3 and 4.
Figs.2, 3 and 4 show the flotation recovery of marmatite and pyrite in the presence of organic depressants. The molecular structures of organic depressants and their depressing effects on marmatite and pyrite are shown in Table 1. It is indicated from Table 1 that the organic compounds only with single group of hydroxyl, carboxyl or amino in the molecular structures are ineffective in depressing marmatite, jamesonite and pyrite, such as glycerine, oxalic acid and melocol. The combinations of these functional groups still cannot enhance the depressing ability of organic depressant, such as lactic acid and nitrilotriacetic acid. The thioglycollic acid containing reductive functional group (—SH) has good depressing performance. The presence of benzene ring in molecule can enhance the depressing performance, for example, marmatite and jamesonite can be depressed by congo red, 4-amino-hydroxybenzene and pyrogallic acid, and pyrite can be depressed by 4-aminobenzene-sulfonic acid. The flotation tests show that the organic depressants with sulfydryl or benzene ring have better effect on depressing marmatite, jamesonite and pyrite than those without these functional groups.
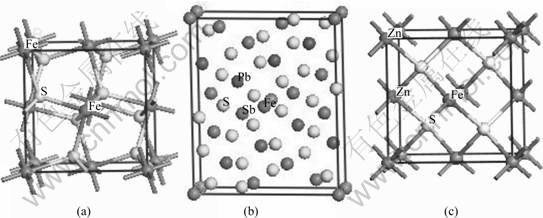
Fig.1 Calculational models of pyrite (a), jamesonite (b) and marmatite (c)
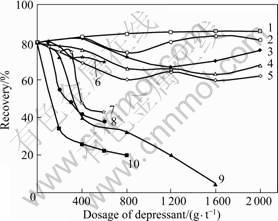
Fig.2 Effect of depressant dosage on marmatite recovery: 1—Oxalic acid; 2—Lactic acid; 3—Nitrilotriacetic acid; 4—Melocol; 5—Glycerine; 6—4-aminobenzene-sulfonic acid; 7—Pyrogallic acid; 8—4-amino-hydroxybenzene; 9—Congo red; 10—Thioglycollic acid
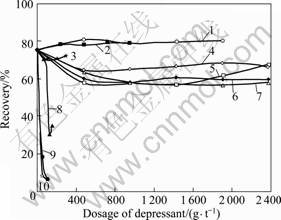
Fig.3 Effect of depressant dosage on jamesonite recovery: 1—Lactic acid; 2—Thioglycollic acid; 3—4-aminobenzene- sulfonic acid; 4—Glycerine; 5—Oxalic acid; 6—Nitrilotriacetic acid; 7—Melocol; 8—Congo red; 9—4-amino- hydroxybenzene; 10—Pyrogallic acid
3.2 Influence of functional group electronegativity of organic depressant
The electronegativity of functional group (signed as xg) involves the influence of other atoms on bonding atom, which can quantitatively describe the reaction activity of functional group with mineral surface. WANG[8] proposed that the smaller the value of the functional group electronegativity, the stronger the interaction of functional group with sulfide mineral surface is. CHEN et al[17] calculated the energies of functional groups interacting with different minerals by thermodynamics, and showed that only the functional group with xg less than 3.5 can interact with the surface of sulfide minerals, and the smaller the value of xg, the stronger the interaction is.
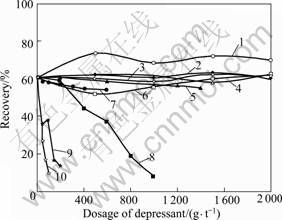
Fig.4 Effect of depressant dosage on pyrite recovery: 1—Lactic acid; 2—Nitrilotriacetic acid; 3—Glycerine; 4—Melocol; 5—Congo red; 6—Oxalic acid; 7—4-amino-hydroxybenzene; 8—Thioglycollic acid; 9—4-aminobenzene-sulfonic acid; 10—Pyrogallic acid
It is suggested that the electronegativity depends on the effective number of valence electrons and the covalent radius of the binding atom[8] as follows:
(1)
where xg is the electronegativity of the functional group; n* is the effective number of the valence electrons; r is the covalent radius of the binding atom. Table 2 lists the values of xg calculated by Eq.(1) (some organic depressants have more than one functional group, and only the functional group with the smallest value of xg is listed in Table 2).
Table 2 shows that marmatite, jamesonite and pyrite cannot be depressed by the functional groups with electronegativity values more than 3.5 (except for —OH (3.80) in pyrogallic acid), such as —OH (4.00) in glycerine, —COOH (4.10) in oxalic acid, —NH2 (3.62) in melocol, —COOH (4.10) in lactic acid, and —COOH (4.00) in nitrilotriacetic acid. Table 2 also shows that sulfide minerals can be depressed by the functional groups with electronegativity values less than 3.5, such as —NH2 (2.70) in congo red, —NH2 (3.20) in 4-amino-hydroxybenzene or —NH2 (2.80) in 4-aminobenzene-sulfonic acid, especially, —SH (2.64) in thioglycollic acid with reductive property can depress marmatite and pyrite well.
3.3 Influence of hydrophilic-hydrophobic indexes of organic depressants
The hydrophilic-hydrophobic index (signed as i) proposed by WANG[8] can be used to classify the flotation reagent. The reagents with index values of i > 3 can be used as depressants, and the larger the value, the
Table 1 Depressing performance of organic depressants with different functional groups
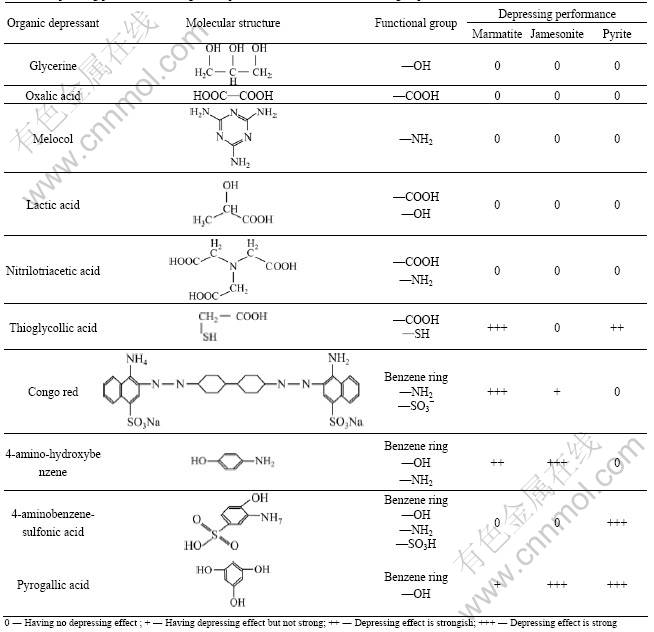
Table 2 Electronegativity and depressing performance of functional group
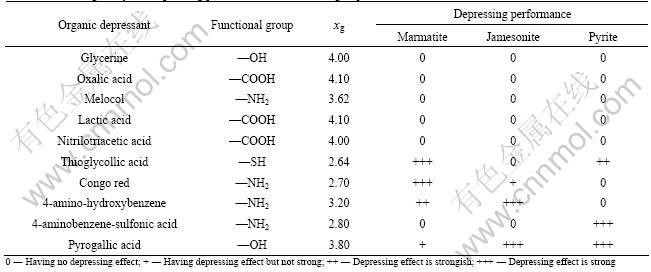
better the depressing effect is. The equation is as follows:
(2)
where xg is the electronegativity of the functional group, xH is the electronegativity of hydrogen, n is the number of carbon in non-polar group, and
is the associated-energy of every —CH2—,and here is given the value of 0.8. Table 3 lists the values of i of different depressants.
Table 3 shows that glycerine (4.50), oxalic acid (10.0), lactic acid (4.76) and nitrilotriacetic acid (3.94) with hydrophilic-hydrophobic indexes greater than 3.0 have no depressing effect on marmatite, jamesonite and pyrite. However, the depressants with benzene rings with small values of i, such as congo red (0.42), 4-amino-hydroxybenzene (0.88), 4-aminobenzene- sulfonic acid (1.80) and pyrogallic acid (1.80), have strong depressing effect on marmatite, jamesonite or pyrite. This result indicates that the hydrophilic- hydrophobic index is not suitable as the criterion of sulfide organic depressants. Thioglycollic acid, whose value of i is 5.2, can depress marmatite and pyrite. This strong depressing performance is mainly ascribed to the presence of reductive group (—SH) in molecule but not to the great value of hydrophilic-hydrophobic index.
For oxidized minerals of strong polarity, depressants with strong polarity are easy to interact with oxidized mineral surface by electrostatic and chemical interaction. The values of i represent the polarity of an organic depressant, hence the greater the value of i, the stronger the interaction of organic depressants with oxidized mineral surface is. However, for the sulfide minerals of weak surface polarity, the interaction of reagent with mineral surface involves the electrochemistry, therefore, not only the hydrophilic-hydrophobic indexes but also the electrochemical activity of molecules of sulfide mineral organic depressants should be considered. The presence of benzene ring can enhance the electrochemical activity of the molecule, and its presence is useful to make the molecule polarized, as well as electrochemically adsorbed on sulfide minerals surface.
3.4 Frontier orbital calculations
The difference (ΔE) between frontier orbitals reflects the extent of interaction between molecules. Table 4 lists the calculational results of ΔE between reagents and minerals.
It has been confirmed[9] that the mechanism of sulfide organic depressants is an electrochemical process. Electron transfer between organic depressants and sulfide minerals results in the desorption of collector coatings from sulfide mineral surface. The frontier orbital theory proposed that the energy differences (ΔE) between the highest occupied molecular orbital (HOMO) and the lowest unoccupied molecular orbital (LUMO) should be small enough to cause the reaction easily.
The calculational results using DMol3 program show that the ELUMO values of marmatite, jamesonite and pyrite are -4.35, -3.92 and -5.70 eV, respectively. Based on the frontier orbital theory that the smaller the absolute difference (|ΔE|) between EHOMO of depressants and ELUMO of mineral, the stronger the interaction of depressant with mineral, it is shown in Table 4 that the depressants with |ΔE| values greater than 1.14 eV have no effects on depressing marmatite (thioglycollic acid is an exception and will be discussed later). For jamesonite and pyrite, likewise, depressants with |ΔE| values greater than 1.57 and 0.21 eV, respectively, are ineffective on depressing jamesonite and pyrite.
Table 3 Values of i and depressing performances of organic depressants
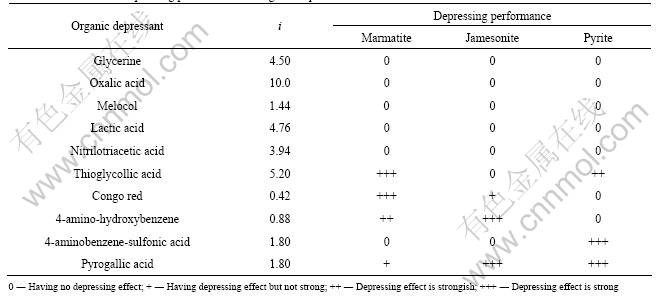
Table 4 Frontier orbital energies of reagents and minerals and depressing performance
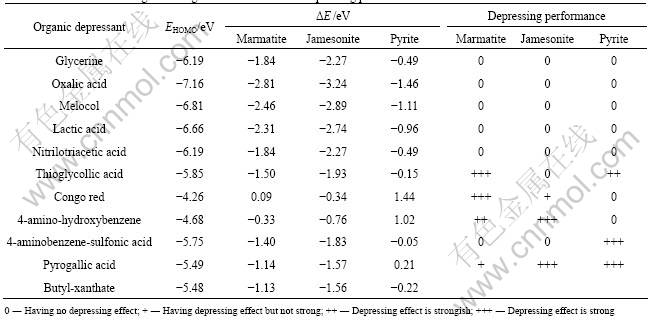
Xanthate and depressant will compete against each other when adsorbing on mineral surface in the flotation process. The energy of HOMO of butyl-xanthate was calculated and the result is listed in Table 4. The |ΔE| value can be used to estimate the extent of the interaction between depressant and mineral, hence, the absolute energy difference value of frontier orbitals between organic depressant (Y) and sulphide minerals (MS) (i.e. |ΔE(Y-MS)|) and between xanthate (X) and sulphide minerals (MS) (i.e., |ΔE(X-MS)| ) can be used as the criterion for the extent of the competing adsorption between depressant and xanthate on mineral surface, i.e.
|ΔE(Y-MS)| ≤ |ΔE(X-MS)| (3)
The depressants consistent with Eq.(3) will be advantageous in adsorbing on mineral surface when competing with xanthate, and consequently have effects on depressing sulphide minerals, i.e. the organic depressants consistent with Eq.(3) that the |ΔE(Y-MS)| is close to or smaller than |ΔE(X-MS)| will have depressing effects on sulphide minerals. Table 4 shows that the ten depressants consistent with Eq.(3) (with the exception of thioglycollic acid) agree well with the flotation tests, i.e. when MS is marmatite, all the organic depressants that have the |ΔE(Y-MS)| values close to or less than 1.13 eV (|ΔE(X-MS)| ) have depressing effects on marmatite (thioglycollic acid is an exception and will be discussed later); when MS is jamesonite, all the organic depressants that have the |ΔE(Y-MS)| values close to or less than 1.56 eV (|ΔE(X-MS)|) have depressing effects on jamesonite; while MS is pyrite, all the organic depressants that have the |ΔE(Y-MS)| values close to or less than 0. 22 eV (|ΔE(X-MS)| ) have depressing effects on pyrite.
The flotation test results clearly show that the presence of benzene ring in molecule can, to some extent, enhance the depressing ability of organic depressant, however, it is not absolutely true. This is due to the fact that, in different molecules, the contribution of benzene ring to the frontier orbital of the molecule is different, whereas the frontier orbitals between organic depressant and mineral should match each other when interaction occurs between them. For instance, congo red, 4-amino- hydroxybenzene and pyrogallic acid all containing
bonds have the |ΔE(Y-MS)| values smaller than 1.13 eV, hence can depress marmatite, however, melocol that also contains large
bond and has the |ΔE(Y-MS)| value (2.46) considerably greater than 1.13 eV, cannot depress marmatite.
Eq.(3) is not suitable for explaining the depressing effect of thioglycollic acid on marmatite because the depressing effect of thioglycollic acid on marmatite is ascribed to the strongly reductive group (—SH) in molecule. Thioglycollic acid has a standard redox potential of -0.33 eV, which can lower the potential of slurry and the rest potential of sulphide minerals, hence the minerals are depressed[18]. Other organic depressants that also contain reductive groups, such as oxalic acid with hydroxyl, 4-amino- hydroxybenzene with hydroxyl and pyrogallic acid with carboxyl, cannot depress marmatite and pyrite because the reductive ability of these groups is too weak to produce a reductive potential that can de sorb the xanthate from mineral surface.
In addition, Table 4 shows that there is no positive correlation between the |ΔE(Y-MS)| value and the extent of depressing effect. For instance, when MS is jamesonite, |ΔE(Y-MS)| value of congo red (0.34 eV) is considerably smaller than that of pyrogallic acid (1.57 eV), however, the latter has a stronger depressing effect on jamesonite (see Fig.3) than the former. The actions of depressant in flotation include the following items. 1) Depressant can interact with mineral. The extent of interaction can be revealed by the extent of the interaction between frontier orbitals (as discussed above). 2) Depressant should be hydrophilic. Thus, the hydrophilic ability of organic depressant should also be considered when considering the interaction among depressant, collector and mineral. Table 3 shows that pyrogallic acid containing three hydroxyls has a stronger hydrophilic ability (with i value of 1.80) than congo red (with i value of 0.42).
4 Conclusions
1) Flotation results show that the organic compounds only with single group of hydroxyl (—OH), carboxyl (—COOH) or amino (—NH2) in molecule are ineffective in depressing marmatite, jamesonite and pyrite, such as glycerine, oxalic acid and melocol. The combinations of these functional groups still cannot enhance the depressing ability of organic depressant, such as lactic acid and nitrilotriacetic acid.
2) The thioglycollic acid containing strongly reductive functional group (—SH) has a good depressing performance. The presence of benzene ring in molecule can enhance the depressing performance, for example, marmatite and jamesonite are well depressed by 4-amino-hydroxybenzene and pyrogallic acid, and pyrite is well depressed by 4-aminobenzene-sulfonic acid and pyrogallic acid.
3) The frontier orbital calculations can well explain the interaction between sulfide mineral and organic depressant. It is shown that the depressants with small |ΔE(Y-MS)| values (the absolute difference of EHOMO of organic depressant (Y) and ELUMO of sulphide minerals (MS) ) can depress marmatite or pyrite, and only when |ΔE(Y-MS)| value is close to or less than |ΔE(X-MS)| value (the absolute difference of EHOMO of xanthate (X) and ELUMO of sulphide minerals (MS)), the depressant can depress marmatite or pyrite.
4) The hydrophilic ability of organic depressants should be considered when applying the frontier orbitals to study their depressing effects on sulphide minerals.
References
[1] VALDIVIESO A L, CERVANTES T C, SONG S, CABRERA A R, LASKOWSKI J S. Dextrin as a non-toxic depressant for pyrite in flotation with xanthates as collector [J]. Minerals Engineering, 2004, 17(9/10): 1001-1006.
[2] SILVESTREA M O, PEREIRA C A, GALERY R, PERES A E C. Dispersion effect on a lead–zinc sulphide ore flotation [J]. Minerals Engineering, 2009, 22 (9/10): 752-758.
[3] LIU Run-qing, SUN Wei, HU Yue-hua, XIONG Dao-ling. Depression mechanism of small molecular mercapto organic depressants on flotation behavior of complex sulfide [J]. The Chinese Journal of Nonferrous Metals, 2006, 16(4): 746-751. (in Chinese)
[4] SUN Wei, LIU Run-qing, HU Yue-hua. Research on depression mechanism of jamesonite and pyrrhotite by organic depressant DMPS [J]. Mining and Metallurgical Engineering, 2005, 25(6): 31-34. (in Chinese)
[5] XIONG Dao-ling, HU Yue-hua, QIN Wen-qing, SUN Wei, LIU Run-qing. Selective flotation separation of marmatite from arsenopyrite by organic depressant [J]. Journal of Central South University (Science and Technology), 2006, 37(4): 670-674. (in Chinese)
[6] HE Ming-fei, XIONG Dao-ling, CHEN Yu-ping, LIU Ya-long, QIN Wen-qing. Mechanism of a novel depressant glyceryl-xanthate sodium in depressing sulfide minerals [J]. Mining and Metallurgical Engineering, 2007, 27(3): 30-32. (in Chinese)
[7] JIAN Sheng, LI Xue-zhi, MU Xiao, WEI Zong-wu. Effect of small molecule organic depressors on flotation behavior of lead and zinc minerals [J]. Metal Mine, 2006(7): 37-39. (in Chinese)
[8] WANG Dian-zuo. Mineral flotation and reagent—Principle and application [M]. Changsha: Central South University of Technology Press, 1986: 30-48, 146-153. (in Chinese)
[9] CHEN Jian-hua, FENG Qi-ming, LU Yi-ping. Energy band model of electrochemical flotation and its application (III)—Effects of organic depressants on structure of sulphide minerals [J]. The Chinese Journal of Nonferrous Metals, 2000, 10(4): 529-533. (in Chinese)
[10] CHEN Jian-hua, FENG Qi-ming, LU Yi-ping. Molecular structure design and interaction principle of sulphide mineral organic depressant [J]. Journal of Guangxi University (Nat Sci Ed), 2002, 27(4): 276-280. (in Chinese)
[11] XIE Xi-de. Energy band theory of solids [M]. Shanghai: Fudan University Press, 2007: 12-55. (in Chinese)
[12] DELLEY B. From molecules to solids with the DMol3 approach [J]. Journal of Chemical Physics, 2000, 113(18): 7756-7764.
[13] DELLEY B. DMol3 DFT studies: From molecules and molecular environments to surfaces and solids [J]. Computational Materials Science, 2000, 17(2/3/4): 122-126.
[14] PERDEW J P, BURKE K, ERNEZERHOF M. Generalized gradient approximation made simple [J]. Physical Review Letters, 1996, 77(18): 3865-3868.
[15] PERDEW J P, CHEVARY J A, VOSKO S H, JACKSON K A, PEDERSON M R, SINGH D J, FIOLHAIS C. Atoms, molecules, solids, and surfaces: Applications of the generalized gradient approximation for exchange and correlation [J]. Physical Review B, 1992, 46(11): 6671-6687.
[16] CHEN Jian-hua, CHEN Ye, ZENG Xiao-qin, LI Yu-qiong. First principle study of effect of Fe impurity on electronic structure and activation of sphalerite surface [J]. The Chinese Journal of Nonferrous Metals, 2009, 19(8): 1517-1523. (in Chinese)
[17] CHEN Jian-hua, FENG Qi-ming, LU Yi-ping. Design of functional groups of flotation reagent [J]. Nonferrous Metals, 1999, 51(2): 19-23. (in Chinese)
[18] CHEN Jian-hua, FENG Qi-ming, LU Yi-ping. Electrochemical mechanism on interaction of TGA with sulphide minerals [J]. Conservation and Utilization of Mineral Resources, 2000(6): 34-38. (in Chinese)
(Edited by YANG Bing)
Foundation item: Project(50864001) supported by the National Natural Science Foundation of China; Project(0991082) supported by Guangxi Natural Science Foundation, China
Corresponding author: LI Yu-qiong; Tel: +86-771-3232200; Fax: +86-771-3233566; E-mail: lyq198205@163.com
DOI: 10.1016/S1003-6326(09)60407-6