
Effects of Mn content on microstructure and electrochemical properties of LaNi4.2-xCo0.6MnxAl0.2 (x=0-0.2) hydrogen storage alloys
JIAO Yong-han(焦永涵), CHENG Yan(成 艳), ZHU Lei(朱 磊),
CHEN Hui(陈 晖), JIAN Xu-yu(简旭宇), WANG Zhong(王 忠)
General Research Institute for Nonferrous Metals, Beijing 100088, China
Received 15 July 2007; accepted 10 September 2007
Abstract: The effects of Mn content on microstructure and electrochemical properties of LaNi4.2-xCo0.6MnxAl0.2 alloys were investigated systematically. The results of XRD analyses indicate that the substitution of Mn does not change the crystal structure, but along with the increase of Mn substitution quantity, the volume of the crystal increases and the ratio of axial to radial lattice parameters (a/c) first increases, then decreases. With the increase of Mn substitution quantity in LaNi4.2-xCo0.6Mnx Al0.2, the discharge capacity, charge efficiency, the self-discharge and high rate discharge capacity increase first and then decrease. The discharge capacity reaches the highest value of 367 mA?h/g (x=0.1) at 0.2C.
Key words: hydrogen storage alloys; crystal structure; electrochemical properties; Mn substitution
1 Introduction
The Ni/MH batteries have been widely applied in many fields, such as portable electronics, electric tools and vehicles due to its high specific energy, high power density, long cyclic life and no pollution[1]. The AB5 type hydrogen storage alloy is the key active materials in negative electrode during the past 30 years. By partial replace the elements in A and B sides by cobalt, aluminum, manganese and other rare earth and transition elements, the electrochemical performance of the hydrogen storage alloys have been significantly improved, especially for the discharge capacity, cycle life stability, electrochemical catalysis activity and hydride decomposition pressure, and anti-oxidation charac- teristics as well[2-6].
It has been found that the manganese substitution can increase the discharge ability, but decrease the cycling stability because the manganese element is liable to precipitate in the alkali solution, which makes the alloys pulverized[7-9]. The common used commercial hydrogen storage alloys contain manganese element in the range of 0.4-0.6[10]. However, no report has been found to reveal the influence of the small quantity substitute of the manganese element to the micro structure and electrochemistry of LaNi5 type alloys. Thus the hydrogen storage materials LaNi4.2-xCo0.6MnxAl0.2 (x=0-0.2) is studied in this paper.
2 Experimental
2.1 Alloy preparation and X-ray diffraction analysis
All samples were prepared by melting the component metals in a water-cooled copper hearth under Ar gas circumstance. The purity of the metals, i.e, La, Ni, Co, Al and Mn is higher than 99.9% (mass fraction), respectively. The ingots were all turned over and remelted three times to ensure good homogeneity. A slight excess of La over composition was needed in order to compensate for evaporative loss under preparation conditions. Thereafter, the ingots were crushed into powder in a stainless steel mortar and screened to a size less than 74 μm.
Crystallographic characteristics of the hydrogen storage alloys were investigated by X-ray diffraction on MSAL–XD 2 X-ray diffractometer (Cu Kα radiation, Bragg–Brentano geometry, 2θ range 10?-90?, step size 0.02?, backscattered rear graphite monochromator).
2.2. Electrochemical measurement
The obtained powders were mixed with Ni powder by mass ratio of 1?3, pressed into disk shaped electrodes of 13 mm in diameter, about 100 mg hydrogen storage alloy in content. The electrode was then tested at 25 ℃ in an open-cell with an electrolyte of KOH (6 mol/L) and a positive electrode of Ni(OH)2/NiOOH. The discharge capacity, high-rate discharge ability, self-discharge and cycling stability of the doped-alloys were measured.
1) For activation behavior and discharge capacity measurements, charging at 60 mA/g for 7.5 h and discharging at 60 mA/g to the end point.
2) For high-rate-discharge ability measurement, charging at 60 mA/g for 7.5 h and discharging at 300 mA/g to the end point.
3) For self-discharge (24 h) measurement, charging at 60 mA/g for 7.5 h, then discharging at 60 mA/g after 24 h.
The end point of the discharge was set to 1.0 V (vs Ni(OH)2/NiOOH)
3 Results and discussion
3.1 Structural characteristics
Fig.1 shows the XRD patterns of the LaNi4.2-xCo0.6- MnxAl0.2 (x=0-0.2) hydrogen storage alloys. It can be seen that the substitution of Ni by Mn does not change the crystal structure which consist of CaCu5-type hexagonal phase, Along with the increase of Mn substitution quantity, the position of main diffraction peak changes a little to the small angle direction.
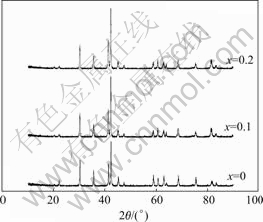
Fig.1 XRD patterns of LaNi4.2-xCo0.6MnxAl0.2 alloy
Table 1 lists the lattice parameters and cell volumes of the LaNi4.2-xCo0.6MnxAl0.2 (x=0-0.2) alloys, which are calculated from the data of Fig.1. It shows that with the increase of Mn substitution, the axes a and c of the alloys crystal cell increase, which are greatly attributed to the atomic radius of Mn (0.179 nm) is bigger than that of Ni (0.162 nm). At the same time, the ratio of axial axis to radial axis (a/c) first increases and then decreases, which is mainly resulting from the change of radial lattice parameter larger than that in the axial direction after the substitution of Mn atoms.
Table 1 Lattice parameters and cell volume (V) of LaNi4.2-x- Co0.6MnxAl0.2 alloys
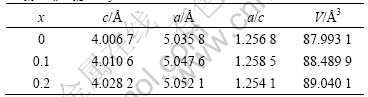
3.2 Electrochemical characteristics
Figs.2 and 3 show the charge characteristics curves of alloy LaNi4.2-xCo0.6MnxAl0.2 (x=0-0.2). It is obvious that charge voltage plateau of the 1st alloy (x=0.1) is lower than that of the 2nd alloy (x=0.2) and higher than that of the 3rd alloy (x=0), its charge voltage keeps lowest value with the increase of the charge capacity. This indicates that small doping content of Mn (x=0.1) can effectively restrain the electrochemical polarization and improve the charge performance of the electrodes.
Figs.4 and 5 show that the discharge plateau of LaNi4.2-xCo0.6MnxAl0.2 (x=0) is the highest of all. This demonstrates the substitution of Mn reduces the discharge discharge plateau of the electrodes.
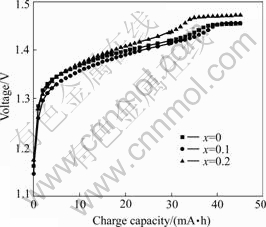
Fig.2 Charge curves of LaNi4.2-xCo0.6MnxAl0.2 alloys
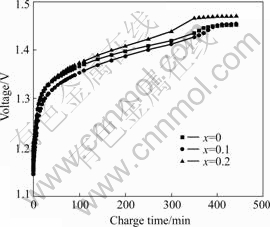
Fig.3 Charge curves of La Ni4.2-xCo0.6MnxAl0.2 alloys
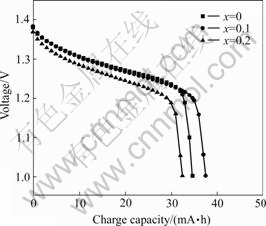
Fig.4 Discharge curves of LaNi4.2-xCo0.6MnxAl0.2 alloys
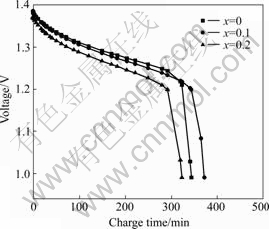
Fig.5 Discharge curves of LaNi4.2-xCo0.6MnxAl0.2 alloys
Table 2 lists the charge and discharge capacities of LaNi4.2-xCo0.6MnxAl0.2 alloys. It shows that the 1st alloy (x=0.1) has the highest discharge capacity (367 mA?h/g) and charge efficiency, while the 2nd alloy (x=0.2) has the lowest discharge capacity (324 mA?h/g) and the charge efficiency. These results indicate that the doping content of Mn has obviously effects on the discharge capacities and charge efficiency. These phenomena are related to the substitution of Mn. As we known, with the increase of the substitution of Ni by Mn, the cell volumes increase as a result of the cell distance of the tetrahedron and the octahedron increase, which contribute to the reduction of the plateau pressure of hydrogen desorption, thus, the discharge characteristic of the electrode is improved. But with the plateau pressure decrease, the dissolving content of the hydrogen in the alloy decreases. As a result, the discharge capacities tend to decrease.v
Table 2 Charge/discharge capacities of LaNi4.2-xCo0.6Mnx Al0.2 alloys
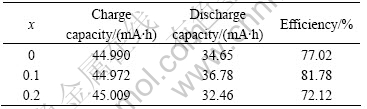
Table 3 lists the self-discharge capacities of the LaNi4.2-xCo0.6MnxAl0.2 alloys. It can be seen that the charge conservation rate(CCR) and the self-discharge capacity(SDC) of the 1st alloy (x=0.1) are the highest (322 mA?h/g, 87%) and the 2nd alloy (x=0.2) is the lowest (257 mA?h/g, 79%), demonstrating a little substitution of Mn can remarkably improve the self- discharge performance of the LaNi4.2-xCo0.6MnxAl0.2 alloys.
Table 3 Self-discharge capacities of LaNi4.2-xCo0.6MnxAl0.2
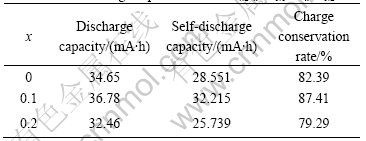
Table 4 and Fig.6 show the high rate discharge capacity of the alloy of LaNi4.2-xCo0.6MnxAl0.2. The substitution of Mn reduces the discharge plateau of electrode, the high-rate discharge capacity and the charge
Table 4 High rate discharge capacities of LaNi4.2-xCo0.6Mnx- Al0.2 alloys
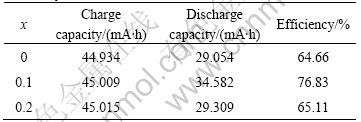
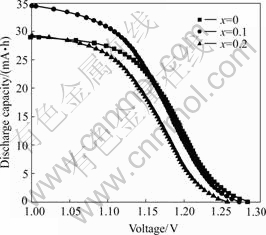
Fig.6 High rate (1C) discharge capacity of alloys LaNi4.2-x- Co0.6MnxAl0.2
efficiency of the 1st alloys (x=0.1) are the highest. Whereas the charge efficiency of the 2nd alloy (x=0.2) is a little higher than that of the 3rd alloy without Mn. This represents that little substitution of Mn could improve the high-rate discharge capacity of the electrodes. In addition, it has been reported that Mn element can be easily dissolved in the electrolyte, which aggravates the brittleness of the alloys and accelerates its pulverization, causing the increase of the specific surface area of alloy. Therefore, the high-rate discharge capacity of the alloy is increased.
4 Conclusions
1) The Mn substitution can not change the crystal structure of LaNi4.2-xCo0.6MnxAl0.2 (x=0-0.2) alloy. With the increase of Mn substitution content, the volume of the crystal increases and the ratio of axial to radial lattice parameter (a/c) first increases and then decreases.
2) The discharge capacity, charge efficiency, self-discharge and high rate discharge capacities of LaNi4.2-xCo0.6MnxAl0.2 behave the same tendency of first increasing and then decreasing with the increase of Mn amount.
References
[1] WILLEMS J J G, PHILIPS J. Metal hydride electrodes stability of LaNi5 related compounds [J]. Res Suppl, 1984, 39(Suppl.l): 35-38.
[2] QIAN Cun-fu, DU Hao, WANG Hong-xiang. The mechanism of action of Co and Mn to the LaNi5 type hydrogen storage alloy [J]. Rare Metal Materials and Engineering, 1999, 28: 68-370.
[3] SENOH H, MORIMOTO K, INOUE H, IWAKURA C, NOTTEN P H L. Relationship between equilibrium hydrogen pressure and exchange current for the hydrogen electrode reaction at MnNi3.9-xMn0.4AlxCo0.7 alloy electrodes [J]. Electrochem Soc 2000, 147: 2451-2455.
[4] SAKAI T, OGURO K, MIYAMURA H, KURIYAMA N, KURIYAMA N, KATO A, ISHIKAKA H. Some facts affecting the cycle lives of LaNi5-based alloy electrodes of hydrogen battery [J]. J Less-Common Met, 1990, 161: 193-202.
[5] WANG X Y,LIU B,YAN J, et al. Effect of state of charge on impedance spectrum of sealed Ni-MH cells [J]. Journal of Alloys and Compounds, 1999, 293-295.
[6] GAMBOA S A, SEBASTIAN P J, et al. Temperature, cycling, discharge current and self-discharge electrochemical studies to evaluate the performance of a pellet metal-hydride electrode [J]. International Journal of Hydrogen Energy, 2001, 26: 1315-1318.
[7] WANG Zhi-xing LI Xin-hai, CHEN Qi-yuan, et al. Structure of Sn doped AB5 stoichiometric hydrogen [J]. Journal of Rare Earths, 2000, 18: 225-228.
[8] WILLEMS J J G, BUSCHOW K H J. From permanent magnets to rechargeable hydride electrodes [J]. J of the Less-Common Metals, 1987, 129: 13-30.
[9] LEI Yong-quan, JIANG Jian-jun, SUN Da-lin, et al. Effect of rare earth composition on the electrochemical properties of multicomponent RENi5-xMx (M=Co, Mn, Ti) alloys [J]. 1995, 231: 553-557.
[10] YUAN Xian-xia. Study on electrochemical behavior of AB5-type hydrogen storage alloys for Ni-MH battery [D]. Shanghai: Shanghai Institute of Microsystem and Information Technology, Chinese Academy of Sciences, 2002: 5-7.
(Edited by LAI Hai-hui)
Corresponding author: JIAO Yong-han; Tel: +86-10-82241241; E-mail: yonghan504682@163.com