
Electrochemical behaviors of tantalum in anhydrous ethanol containing hydrogen sulfate ions
YANG Hai-ping1, YANG Sheng-hai1, CAI Ya-nan2, HOU Guo-feng1, XIA Jiao-yun1, TANG Mo-tang1
1. School of Metallurgical Science and Engineering, Central South University, Changsha 410083, China;
2. School of Minerals Processing and Bioengineering, Central South University, Changsha 410083, China
Received 1 March 2010; accepted 11 June 2010
Abstract: The electrochemical behaviors of Ta in tetrabutylammonium hydrogen sulfate (TBAHS) ethanol solutions were studied using potentiodynamic polarization, cyclic voltammetry, potentiostatic current time transient and impedance techniques. The results revealed that no active-passive transition is presented in the cyclic voltammogram, and the anodic current density increases with the increase of solution temperature, TBAHS concentration, potential scan rate and water content. The apparent activation energy is about 43.389 kJ/mol and the dissolution process is diffusion-controlled. Potentiostatic measurements showed that the current density gradually decays to a steady value when the potential is low; however, when the potential is higher than a certain value, the current density initially declines to a minimum value and then increases gradually. The resistance of passive film decreases with increasing potential, and inductive loops are presented when the potential is higher than 2.0 V.
Key words: anhydrous ethanol; electrochemical impedance spectra; polarization curve; tantalum; tetrabutylammonium hydrogen sulfate
1 Introduction
Tantalum oxide (Ta2O5) has received considerable attention as capacitor dielectrics in dynamic random access memories (DRAM)[1], ultra large scale integrated circuits (ULSI) gate dielectrics[2], antireflection coatings for solar cells[3], protective coating materials for chemical equipment[4], optical waveguides[5], solid state oxygen sensors[6], filters[7] and barriers to copper diffusion[8]. Ta2O5 films can be deposited by several methods, such as sputtering, anodic oxidation, and chemical vapor deposition (CVD). Among these, CVD is the most promising method because it provides good step coverage, a low rate of defective films, the possibility of depositing films on substrates with large areas, and low damage to substrates[9]. Metal alkoxides are widely used precursors in CVD processes of oxides as they possess many excellent properties. In contrast to metal halide processes, the films deposited with alkoxide processes do not result in harmful halide impurities and the deposition byproduct is less corrosive[10]. Ta2O5 films have been deposited by CVD using several precursor materials such as Ta(OC2H5)5, Ta(OCH3)5, TaCl5 and Ta[N(CH3)2]5. Among theses precursors, tantalum ethoxide (Ta(OC2H5)5) is the most preferred one because of its 100% volatility and superior thermal stability[11].
The most common preparation method of tantalum alkoxides is based on the tantalum chloride and alcohol[12]. This method has the disadvantage that poor yield (less 70%) of tantalum ethoxide is obtained due to the formation of very fine NH4Cl particles adsorbed by a large number of products, which can’t be filtered off. To overcome the difficulties, large amounts of poisonous solvents such as benzene are used. In addition, the necessary reagents are scarce and the reactions involve various kinds of side processes which contaminate the products[13].
For these reasons, the direct electrochemical synthesis of metal alkoxides by anode dissolution of metals in absolute alcohols in the presence of a conductive admixture seems a very promising method. The electrochemical method has great promise for the direct conversion of the less noble electropositive metal to their alkoxides owing to its simplicity and high productivity as well as its continuous and non-polluting character (with hydrogen as the major by-product)[14]. In 1972 the electrochemical synthesis of tantalum ethoxide by anodic dissolution of tantalum in ethanol in the presence of NH4Cl as an electrocondutive additive was patented[15]. Since then, tantalum ethoxide was prepared by SHREIDER et al[16], TUROVA et al[17] and YANG et al[18-19] using the electrochemical method. The electrosynthesis technique has been successfully employed in Russia for the commercial production of alkoxides of Y, Ti, Zr, Nb, Ta, Mo, W, Cu, Ge, Sn, and other metals[14]. Despite the extensive study in the electrochemical synthesis of tantalum ethoxide, to the author’s knowledge, only little literature[20-21] reported the electrochemical behaviors of this process. In the present work, the electrochemical behaviors of tantalum are investigated in anhydrous ethanol containing supporting electrolytes, by using the potentiodynamic polarization, cyclic voltammetry, current time transient and impedance techniques, which are very useful to understand the reaction mechanism.
2 Experimental
A tantalum rod, with size of d2.25 mm×180 mm, was supplied by Zhuzhou Cemented Carbide Group Corp. Ltd, China, with a chemical composition (mass fraction, %): O 0.02, C 0.005, N 0.003, Fe 0.005, Ni 0.005, Cr 0.005, Nb 0.002, W 0.002, Mo 0.001, Si 0.002, Mn 0.001, and Ta balance. The apparent exposed area was 0.04 cm2. The electrode was successively polished with a series of emery papers, from a coarse grade of 400 to fine grade of 1 200, rinsed with acetone, ethanol, and finally dipped in the electrolytic cell.
The experiments were performed in a 300 mL volume Pyrex glass cell using Pt foil and a saturated calomel electrode (SCE) as auxiliary and reference electrodes, respectively. To avoid contamination, the SCE was connected via a bridge filled the solution in test, the tip of which was pressed against the surface of the working electrode to minimize the IR drop. All potential given in this work are referred to this reference electrode. All chemicals used were of analytically pure grade. The electrolyte solutions were prepared from anhydrous ethanol and tetrabutylammonium hydrogen sulfate (TBAHS). Prior to the experiments, the solutions were deaerated with N2 and the electrode was held at -1 V for 300 s to electro-reduce any possible oxidized surface species.
The electrochemical measurements were carried out by using a potentiostat/galvanostat (CHI 660C Electrochemical Workstation provided by Shanghai CH Instrument Company, China) and connected with a computer. The potentiodynamic polarization curves were recorded by changing the electrode potential automatically from -1.0 V to 3.0 V at the desired scanning rate. Cyclic voltammetric measurements were carried out by sweeping linearly the potential from the starting potential into the positive direction at a given scanning rate up to the required potential value and then reversing with the same scanning rate to the starting potential to form one complete cycle. The anodic current transients at a constant anodic potential Ea were recorded for 300 s. Electrochemical impedance spectra (EIS) measurements were carried out using AC signals of amplitude 5 mV at a certain potential in the frequency range from 100 kHz to 0.01 Hz. The EIS results were analyzed by Zview Software. Each experiment was performed with freshly prepared solution and a newly polished set of electrodes. All measurements were carried out at room temperature (25±1 °C).
3 Results and discussion
3.1 Influence of temperature
Figure 1 shows the cyclic voltammogram of Ta in anhydrous ethanol containing 0.1 mol/L TBAHS with a scanning rate of 10 mV/s. On the positive going scan, the cathodic current density decreases gradually, forming a small cathodic current plateau just before reaching a zero value at the corrosion potential (φcorr). Subsequently, the anodic current density rises to a steady value, Jpass, indicating the onset of passivation. The value of Jpass is limited by the chemical dissolution of the film. The chemical film dissolution is counterbalanced by film formation at the steady-state of polarization. It is clear that the curve does not exhibit active-passive transition. The passive region extends over a potential range of about 1 200 mV and the current density begins to increase quickly, leading to a transpassive region. On the reversing sweep, the current density decays gradually without any peak in the curve.
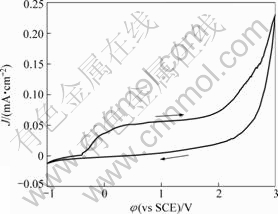
Fig.1 Typical cyclic voltammogram of Ta in anhydrous ethanol containing 0.1 mol/L TBAHS with scanning rate of 10 mV/s
Figure 2 illustrates the solution temperature effect on the cyclic voltammograms of Ta in 0.1 mol/L TBAHS solution of anhydrous ethanol at a scanning rate of 40 mV/s. All the curves shift to the left and to the top direction with the increase of temperature, suggesting the enhanced dissolution effects of temperature. It is likely that the increasing temperature accelerates the rates of migration and diffusion of the reactant and product species. In addition, the increasing temperature can enhance the solubility of the passive film[22].
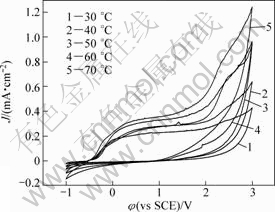
Fig.2 Effect of temperature on cyclic voltammograms of Ta in anhydrous ethanol containing 0.1 mol/L TBAHS with scanning rate of 40 mV/s
In order to obtain the apparent activation energy for the corrosion process, the Tafel curves at different temperature were made, as shown in Fig.3. The shape and evolvement tendency of each curve look similar, indicating dissolution mechanisms don’t change at all. With the increase of solution temperature, the curves shift to the right direction corresponding to a larger current density. It is well known that the corrosion current density is a parameter which represents the corrosion rate of a material. So, the increase in temperature accelerates the corrosion rates of tantalum. The apparent activation energy, Ea, of Ta in 0.1 mol/L TBAHS solution of anhydrous ethanol can be calculated from Arrhenius equation[23]:
(1)
where Jcorr is the corrosion current density calculated by extrapolation of the linear logarithmic sections of the cathodic and anodic Tafel lines to the point of intersection, R is the universal gas constant, T is the absolute temperature and A is the pre-exponential factor. A plot of lg Jcorr vs 1/T gives a straight line, as shown in Fig.4. The value of Ea obtained from the slop of the straight line is 43.389 kJ/mol.
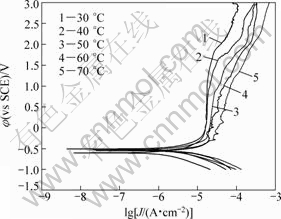
Fig.3 Tafel curves recorded for tantalum in absolute ethanol containing 0.1 mol/L TBAHS at different temperatures with scanning rate of 2 mV/s
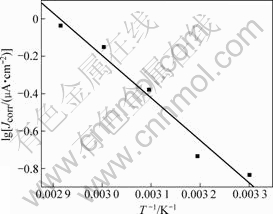
Fig.4 Arrhenius plots of lg(Jcorr) vs 1/T for Ta in 0.1 mol/L TBAHS solution of anhydrous ethanol
3.2 Influence of TBAHS concentration
The influence of TBAHS concentration on anodic potentiodynamic polarization curves of tantalum is given in Fig.5. It is clear that the increase in concentration of
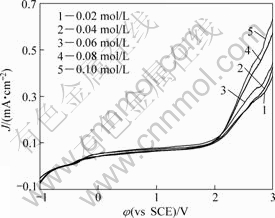
Fig.5 Potentiodynamic polarization curves for Ta in anhydrous ethanol with various TBAHS concentrations with scanning rate of 10 mV/s
the TBAHS enhances the anodic current density. The acceleration effect of the TBAHS may be due to the weakening of the passive film as a result of the competition between its formation and the formation of the soluble product and the increase of the number of aggressive hydrogen sulfate ions that attack the passive layer[24].
3.3 Influence of potential scanning rate
Figure 6 presents the effect of potential scanning rate (v) on the cyclic voltammetry curves of Ta in anhydrous ethanol with 0.1 mol/L TBAHS. It is seen that the current density increases with increasing sweep rate. Figure 7 shows the relationship between the anodic currents and the square root of the scan rate (v1/2) at three potentials. Under these potentials, the current density is proportional to v1/2, which means that the anodic dissolution processes are diffusion-controlled[25].
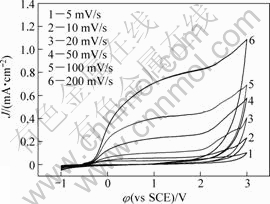
Fig.6 Effect of scanning rate on cyclic voltammograms of tantalum in anhydrous ethanol with 0.1 mol/L TBAHS
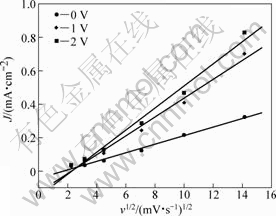
Fig.7 Dependence of anodic current density at various potentials on square root of potential scanning rate
3.4 Influence of water content
The dependence of cyclic voltammograms of Ta on water content is shown in Fig.8. One of the distinctive characteristic is that the anodic passive current density increases gradually with the increase of water content. The other feature is that the passive region enlarges with the increase of water content. The phenomenon may be related to the competition between the enhancement of passivation and the increase of conductance and diffusion coefficient due to the presence of water.
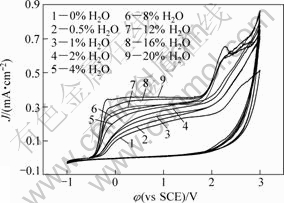
Fig.8 Effect of water content on cyclic voltammograms of Ta in 0.05 mol/L TBAHS ethanol solution with scanning rate of 50 mV/s
3.5 Influence of electrode potential
Figure 9 displays the potentiostatic current-time transients for Ta in 0.1 mol/L TBAHS solution of anhydrous ethanol at various potential (φ). For φ≤1.2 V, the current density decreases with time to attain a steady state current value. The fall of current density may be related to a film growth on the electrode surface. However, at φ≥2.0 V, the current transient initially decreases to a minimum value at a certain time and then rises gradually, indicating that the increasing potential contributes to the dissolution of passive film.
EIS is a very effective technique, which can help for analyzing various steps involved in an electrochemical reaction by measuring the impedance system response to a small AC potential signal in a wide frequency range[26]. The characteristic Nyquist and Bode diagrams of Ta in 0.1 mol/L TBAHS solution of anhydrous ethanol at various electrode potentials are presented in Figs.10 and 11, respectively. It is seen that the impedance decreases slightly when increasing potential from -0.4 V to 1.2 V. However, the impedance declines sharply at 2.0 V. In fact, the impedance of 2.0 V is two decades smaller than that of -0.4 V, as shown in Fig.11. Afterwards, the decline tendency slows down clearly when further increasing potential. The appearance of an inductive loop at φ≥2.0 V is observed, which may represent the commencement of the incubation period for pitting corrosion[27]. Only the inductive loops and no capacitance loop are observed in Nyquist diagram. So, tantalum is in the incubation period for pitting corrosion when the potential is less than 3.6 V.
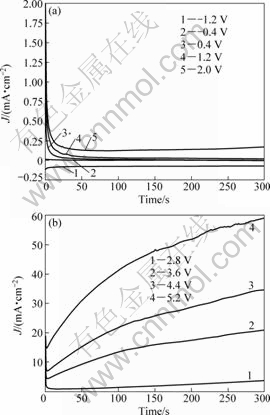
Fig.9 Potentiostic current-time transients for Ta in 0.1 mol/L TBAHS solution of anhydrous ethanol at various potential
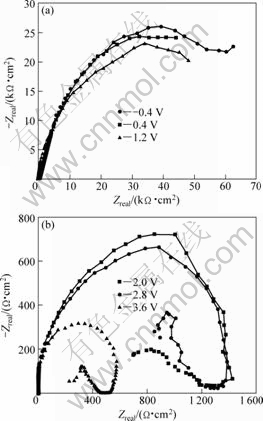
Fig.10 Nyquist plots of Ta in 0.1 mol/L TBAHS solution of anhydrous ethanol at various electrode potentials
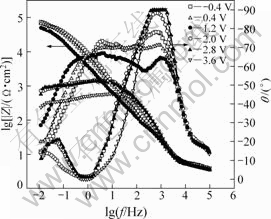
Fig.11 Bode plots of Ta in 0.1 mol/L TBAHS solution of anhydrous ethanol at various electrode potentials
4 Conclusions
1) The dissolution behaviors of tantalum in TBAHS ethanol solutions depend on applied anodic potential, temperature, potential scanning rate, TBAHS and water concentration. Cyclic voltammetry curves exhibit no active-passive transition, and the passivity is due to the presence of thin oxide film on the anode surface. The anodic current density increases with the increase of solution temperature, TBAHS concentration, potential scanning rate and water content. The apparent activation energy in the range of the studied temperature is about 43.389 kJ/mol, and the current density is proportional to the square root of the scanning rate, which suggests that the anodic dissolution processes are diffusion-controlled.
2) The potentiostatic current-time transients reveal that the current density gradually decays to a steady value when the potential is low; however, when the potential is higher than a certain value, the current density initially decreases to a minimum value and then increases gradually.
3) The resistance of passive film decreases with increasing potential, and inductive loops are presented when the potential is higher than 2.0 V. No pitting corrosion is observed under the potentials ranging from -0.4 V to 3.6 V.
References
[1] HE X, WU J, LI X, GAO X, WU L, ZHAO L, GAN X, ZHUGE F. Characterization of high quality tantalum pentoxide film synthesized by oxygen plasma enhanced pulsed laser deposition [J]. Thin Solid Films, 2009, 518: 94-98.
[2] LAN L, PENG J, SUN M, ZHOU J, ZOU J, WANG J, CAO Y. Low-voltage, high-performance n-channel organic thin-film transistors based on tantalum pentoxide insulator modified by polar polymers [J]. Organic Electronics, 2009, 10: 346-351.
[3] HE X, WU J, LI X, GAO X, GAN X, ZHAO L. Effects of the post-annealing ambience on the microstructure and optical properties of tantalum oxide films prepared by pulsed laser deposition [J]. Journal of Alloys and Compounds, 2009, 478: 453-457.
[4] MASUDA Y, WAKAMATSU S, KOUMOTO K. Site-selective deposition and micropatterning of tantalum oxide thin films using a monolayer [J]. Journal of the European Ceramic Society, 2004, 24(2): 301-307.
[5] CHANDRA S V J, UTHANNA S, RAO G M. Effect of substrate temperature on the structural, optical and electrical properties of dc magnetron sputtered tantalum oxide films [J]. Applied Surface Science, 2008, 254(7): 1953-1960.
[6] KRISHNAN R R, GOPCHANDRAN K G, MAHADEVANPILLAI V P, GANESAN V, SATHE V. Microstructural, optical and spectroscopic studies of laser ablated nanostructured tantalum oxide thin films [J]. Applied Surface Science, 2009, 255(16): 7126-7135.
[7] MASSE J P, SZYMANOWSKI H, ZABEIDA O, AMASSIAN A, KLEMBERG-SAPIEHA J E, MARTINU L. Stability and effect of annealing on the optical properties of plasma-deposited Ta2O5 and Nb2O5 films [J]. Thin Solid Films, 2006, 515(4): 1674-1682.
[8] LINTANF-SALAUN A, MANTOUX A, DJURADO E, BLANQUET E. Atomic layer deposition of tantalum oxide thin films for their use as diffusion barriers in microelectronic devices [J]. Microelectronic Engineering, 2010, 87(3): 373-378.
[9] MURALI S, DESHPANDE A, TAKOUDIS C G. Modeling of the metalorganic chemical vapor deposition of tantalum oxide from tantalum ethoxide and oxygen [J]. Industrial and Engineering Chemistry Research, 2005, 44(16): 6387-6392.
[10] ALEN P, VEHKAMAKI M, RITALA M, LESKELA M. Diffusion barrier properties of atomic layer deposited ultrathin Ta2O5 and TiO2 films [J]. Journal of the Electrochemical Society, 2006, 153(4): G304-G308.
[11] KUKLI K, AARIK J, AIDLA A, SIIMON H, RITALA M, LESKEL M. In situ study of atomic layer epitaxy growth of tantalum oxide thin films from Ta(OC2H5)5 and H2O [J]. Applied Surface Science, 1997, 112: 236-242.
[12] REUTER K, ZELL F, EBNER M. Process for the preparation of high-purity zirconium, hafnium, tantalum and niobium alkoxides: US, 7273943 [P]. 2007-09-25.
[13] SHREIDER V A, TUREVSKAYA E P, KOSLOVA N I, TUROVA N Y. Direct electrochemical synthesis of metal alkoxides [J]. Inorganica Chimica Acta, 1981, 53: L73-L76.
[14] BRADLEY D, MEHROTRA R, ROTHWELL I, SINGH A. Alkoxo and aryloxo derivatives of metals [M]. London: Academic Press, 2001: 4-51.
[15] TRIPP T. Production of alkoxides: US, 3730857 [P]. 1973-05-01.
[16] SHREIDER V A, TUREVSKAYA E P, KOZLOVA N I, TUROVA N Y. Direct electrochemical synthesis of metal alcoholates [J]. Russian Chemical Bulletin, 1981, 30(8): 1363-1367.
[17] TUROVA N Y, KOROLEV A V, TCHEBUKOV D E, BELOKON A I, YANOVSKY A I, STRUCHKOV Y T. Tantalum(V) alkoxides: Electrochemical synthesis, mass-spectral investigation and oxoalkoxocomplexes [J]. Polyhedron, 1996, 15(21): 3869-3880.
[18] YANG Sheng-hai, PAN Ze-qiang, LI Zhao-hui, TANG Mo-tang, QIU Guan-zhou. Electrochemical synthesis of tantalum ethoxide using sacrificing anode [J]. Rare Metals Materials and Engineering, 2006, 35(4): 625-628. (in Chinese)
[19] YANG Sheng-hai, CHEN Yong-ming, YANG Hai-ping, LIU Yin-yuan, TANG Mo-tang, QIU Guan-zhou. Preparation of high- purity tantalum ethoxide by vacuum distillation [J]. Transactions of Nonferrous Metals Society of China, 2008, 18(1): 196-201.
[20] YANG Hai-ping, YANG Sheng-hai, CAI Ya-nan, HOU Guo-feng, TANG Mo-tang. Electrochemical behavior of tantalum in anhydrous ethanol [J]. Journal of the Electrochemical Society, 2010, 157(3): D168-D171.
[21] YANG Hai-ping, YANG Sheng-hai, CAI Ya-nan, HOU Guo-feng, TANG Mo-tang. Effect of bromide ions on the corrosion behavior of tantalum in anhydrous ethanol [J]. Electrochimica Acta, 2010, 55: 2829-2834.
[22] AMIN M A, ABD EL-REHIM S S, EL-SHERBINI E E F, MAHMOUD S R, ABBAS M N. Pitting corrosion studies on Al and Al-Zn alloys in SCN-solutions [J]. Electrochimica Acta, 2009, 54(18): 4288-4296.
[23] IBRAHIM M A M, ABD EL-REHIM S S, HAMZA M M. Corrosion behavior of some austenitic stainless steels in chloride environments [J]. Materials Chemistry and Physics, 2009, 115(1): 80-85.
[24] HASSAN H H, FAHMY K. Pitting corrosion of tin by acetate anion in acidic media [J]. International Journal of Electrochemical Science, 2008, 3: 29-43.
[25] BARD A, FAULKNER L. Electrochemical methods, fundamentals and applications [M]. New York: John Wiley& Sons, 2001: 235.
[26] DU T, TAMBOLI D, LUO Y, DESAI V. Electrochemical characterization of copper chemical mechanical planarization in KIO3 slurry [J]. Applied Surface Science, 2004, 229: 167-174.
[27] LEE C P, CHEN Y Y, HSU C Y, YEH J W, SHIH H C. Enhancing pitting corrosion resistance of AlxCrFe1.5MnNi0.5 high-entropy alloys by anodic treatment in sulfuric acid [J]. Thin Solid Films, 2008, 517(3): 1301-1305.
钽在硫酸氢根离子无水乙醇溶液中的电化学行为
杨海平1,杨声海1,蔡亚楠2,侯国锋1,夏娇云1,唐谟堂1
(1. 中南大学 冶金科学与工程学院,长沙 410083;2. 中南大学 资源加工与生物工程学院,长沙 410083)
摘 要:采用动电位极化、循环伏安、电流暂态和阻抗等技术研究钽在四丁基硫酸氢铵(TBAHS)乙醇溶液中的电化学行为。研究结果表明:循环伏安曲线中没有活化-钝化转变,阳极电流密度随溶液温度、TBAHS浓度、电位扫描速率和水含量增加而增加;表观活化能为43.389 kJ/mol,溶解过程为扩散控制。恒电位测试结果表明,当电位较低时,电流密度逐渐降至一稳定值;当电位高于某一值时,电流密度最初下降至一最小值然后逐渐增加。钝化膜电阻随电位升高而减小,当电位高于2.0 V时,出现感抗弧。
关键词:无水乙醇;电化学阻抗谱;极化曲线;钽;四丁基硫酸氢铵
(Edited by LAI Hai-hui)
Foundation item: Project (2007AA03Z425) supported by the Hi-tech Research and Development Program of China; Project (50404011) supported by the National Natural Science Foundation of China
Corresponding author: YANG Sheng-hai; Tel: +86-731-88830470; E-mail: 75894838@163.com
DOI: 10.1016/S1003-6326(11)60696-1