J. Cent. South Univ. (2016) 23: 2475-2482
DOI: 10.1007/s11771-016-3306-7

Electrochemical behavior of Mg-Al-Pb alloy in 3.5% NaCl solution
WEN Li(文利)1, 2, YU Kun(余琨)1, 2, FANG Hong-jie(房洪杰)1, XIONG Han-qing(熊汉青)1, 2, YIN Xiang(尹翔)2,
ZHU Hua-long(朱化龙)1, MA Jia-ji(马家骥)1, JIANG Da-yue(姜大越)1
1. Department of Materials Science and Engineering, Yantai Nanshan University, Yantai 265713, China;
2. School of Materials Science and Engineering, Central South University, Changsha 410083, China
Central South University Press and Springer-Verlag Berlin Heidelberg 2016
Abstract: An investigation on electrochemical behavior of Mg-5%Pb alloy, Mg-6%Al alloy and Mg-6%Al-5%Pb alloy (mass fraction) in 3.5% NaCl (mass fraction) solution was conducted using electrochemical measurements and corroded morphology observation, in which solid solution and the as-aged state of each alloy were compared to discuss the influence mechanism of lead and aluminium on the electrochemical properties of alloys. The X-ray diffraction (XRD) analysis was performed to make microstructure characterization. The electrochemical results indicate that the corrosion of Mg-5%Pb alloy is predominated by homogeneous pitting and dissolution of PbCl2 film due to Cl ions attack, while corrosion crevice propagates along grain boundaries in solid solution of Mg-6%Al alloy and the micro galvanic corrosion also plays vital role in Mg17Al12 phase containing experimental alloys. The co-existence of lead and aluminium in magnesium alloy increases corrosion current density and electrochemical activity as well. The comparison between solid solution and the as-aged state demonstrates that Mg2Pb and Mg17Al12 somewhat increase corrosion resistance but lighten anodic polarization by facilitating corrosion product flaking off.
Key words: Mg-Al-Pb alloy; electrochemical behavior; corrosion; solid solution; as-aged state
1 Introduction
Recently, magnesium alloys are widely used as one of the most important materials for both structural components or anodes applied in automobile industries, sonobuoys, underwater devices and air-sea rescue equipments [1-2]. Although Mg-based materials own the superiority of good mechanical properties such as low density and high specific strength [3-4], their electrochemical performance has evoked a great number of investigations in order to better meet different requirements for various applications. The Mg-Al series alloys, i.e., AZ31, AZ61 and AZ91, have been largely reported in previous studies in terms of their electrochemical behavior in NaCl dilute solutions [5-6]. Among them, the influence of β-Mg17Al12 phase on corrosion of AZ91 was detailedly studied, which is a common existing phase in Mg-Al alloys contributing a lot to corrosion process. Meanwhile, the lead added magnesium alloys were also researched in some literatures to clarify the effect of lead on mechanical and corrosion properties of magnesium alloys. SRINIVASAN et al [7] reported that Pb suppressed the formation and growth of discontinuous precipitation of β-Mg17Al12 and increased its stability as well. It was also found that the addition of Pb in Mg-10%Al-12%Si alloy decreased the Mg2Si phase and consequently lowered the mass hardness of the alloy [8]. Additionally, some investigations were conducted about the microstructure and discharge behavior of Mg-6%Al-5%Pb (AP65) (mass fraction) alloy for it is a potential anode material used in seawater activated battery.
Studies mentioned above suggest that elements Pb and Al both play vital roles in magnesium corrosion. Herein, three alloys named Mg-5%Pb alloy, Mg-6%Al alloy and Mg-6%Al-5%Pb alloy (mass fraction) were designated to investigate the electrochemical behavior of single Pb and single Al added magnesium alloys and the combined effect of these two elements on magnesium corrosion. Also, the solid solution and the as-aged state of each alloy were compared to further investigate the affecting mode of elements Pb and Al.
2 Experimental
Argon atmosphere protection was used in a resistance furnace to prepare Mg-5%Pb alloy, Mg-6%Al alloy and Mg-6%Al-5%Pb alloy by melting pure magnesium (>99.95%), aluminium (>99.99%) and lead (>99.99%) at 760°C. The molten experimental alloys were then cast by pouring into a preheated cylindrical steel mold (217 mm×52 mm ). After removed form mold, the as-cast alloy ingots were homogenized at 400°C for 24 h and extruded at 380°C to obtain the plates of 7.8 mm in thickness. The solid solution (SS) treatment was conducted by keeping specimens in resistance furnace for 4 h at 300°C first and for 44 h at 400°C later, aiming to make the added elements dissolve into and diffuse fully through the matrix Mg. Finally, a part of specimens were subjected to aging treatment at 200°C for 24 h. Additionally, a Quanta-200 scanning electron microscope (SEM) was utilized to characterize the morphology of the corroded surface without discharge products of all experimental alloys and the crystalline phases were identified by an X-ray diffraction meter (XRD, D/Max 2550) using Cu Kα X-ray radiation from 10°to 90°with a scan speed of 8 (°)/min.
All electrochemical measurements were carried out in an Solartron SI 1287 potentiostat/galvanostat system with three-electrode configuration. And the electrolyte was 3.5% NaCl solution. The temperature of the electrolyte was controlled at (25±1) °C with a thermostat water-bath. By selecting a platinum plate as counter electrode and saturated calomel electrode (SCE) as reference electrode, the electrochemical properties of experimental specimens, namely working electrode, were evaluated by exposing a surface of 10 mm×10 mm to the electrolyte. Potentiodynamic polarization tests were conducted with a scan speed of 1 mV/s from 1.9 V (vs SCE) to 1.3 V (vs SCE) after an immersion in 3.5% NaCl solution for 5 min to achieve steady open circuit potentials (OCP). And the potential-time curves were obtained by experimental alloys being polarized at current densities of 150 and 350 mA/cm2 for 1200 s, respectively. The electrochemical impedance spectra (EIS) with perturbation amplitude of 5 mV were recorded after 20 min galvanostatic polarization in electrolyte from 10000 Hz to 0.1 Hz. Three parallel experiments were conducted for each electrochemical test to minimize the error.
3 Results and discussion
3.1 Phase identification of alloys
The XRD results in Fig. 1 display the as-aged states of three experimental alloys, illustrating that the microstructure of Mg-5%Pb alloy comprises of single Mg2Pb phase and the precipitate in Mg-6%Al alloy is identified as Mg17Al12 phase, which is in good agreement with many investigations about Mg-Al alloys [12-14]. For the investigated Mg-6%Al-5%Pb alloy, both of these two precipitated phases are found to co-exist. It can be seen that the peak intensity of Mg17Al12 phase in Mg-6%Al-5%Pb alloy decreases in contrast to Mg-6%Al alloy, which signifies an amount reduction of Mg17Al12 phase. This result is in accordance with the conclusion of CANDAN et al [15] reported in their studies, saying that Pb effectively reduces the diffusion of Al and Mg atoms and consequently decreases the amount of Mg17Al12 phase. However, element Al seems to make little difference in precipitation of Mg2Pb in terms of its amount, as is indicated by the comparison of peak intensity of Mg2Pb phase in Mg-5%Pb alloy and Mg-6%Al-5%Pb alloy. For solid solutions of three kinds of experimental alloys, all alloying elements dissolve into α-Mg matrix and distribute uniformly.
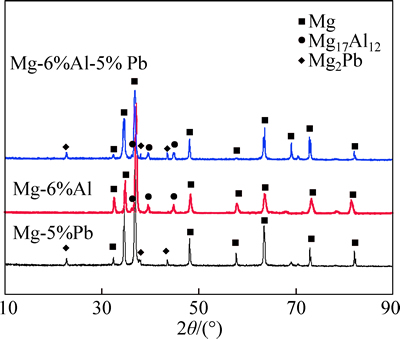
Fig. 1 XRD patterns of experimental alloys in as-aged state
3.2 Electrochemical behavior
The potentiodynamic polarization curves of Mg-5%Pb alloy, Mg-6%Al alloy and Mg-6%Al-5%Pb alloy are shown in Fig. 2. It is found that the solid solution and the as-aged state of each alloy exhibit the similar potentiodynamic polarization behavior but possess different magnitudes of corrosion current density (Jcorr) and corrosion potential (φcorr), which suggests that the corrosion mechanism relies mainly not on the form of alloying elements but on their species. The two curves in Fig. 2(a) all contain three regions, representing anodic activation region (I), passivation region (II) and reactivation region (III), respectively. Each region illustrates distinct corrosion stages of the experimental alloys. The occurrence of passivation region (II) is exactly due to the formation of PbCl2 film in surface of the corroded experimental alloy [16-19]. When it reaches pitting potential φpit, Cl ions react with PbCl2, causing simultaneous chemical dissolution, rupture or breakdown of the passive film [20]. Thus, the Cl ions touch the metal surface directly and the reactivation process (region III) proceeds by dissolution of matrix Mg. For comparison of the solid solution with the as-aged state of Mg-5%Pb alloy, it is found that the former has larger corrosion current density (36.57 μA/cm2) while exhibiting more positive corrosion potential (-1.653 V.), manifesting that the precipitated Mg2Pb phase can increase the corrosion tendency but decrease the rate of corrosion once it starts. This can be interpreted by following reasons: on one hand, the Mg2Pb phase acts as cathode for the micro galvanic corrosion and consequently drives the dissolution of matrix Mg. On the other hand, the corrosion is effectively blocked by the isolated Mg2Pb phase because of its steady property, thus decreasing the corrosion rate.
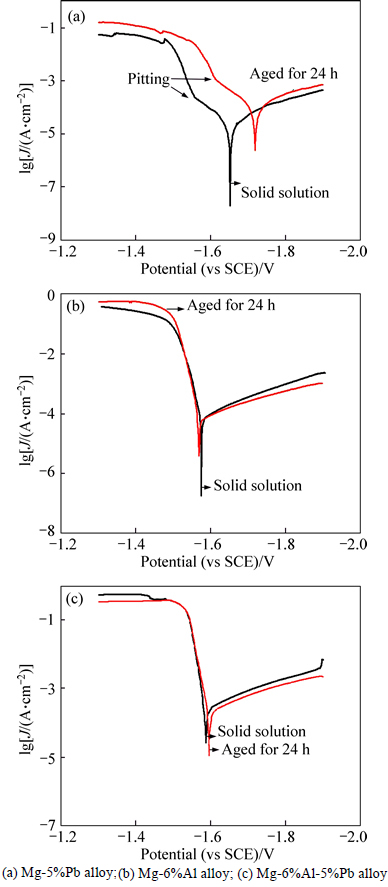
Fig. 2 Potentiodynamic polarization curves:
In Figs. 2(b) and (c), it can be seen that there exits no region III, implying that the addition of aluminium in Mg-6%Al alloy and Mg-6%Al-5% Pb alloy makes some difference in corrosion mechanism. Actually, the pitting also occurs in these two alloys though visible observation can not be made in curves. The phenomenon may be attributed to the reason that the Mg-6%Al alloy and Mg-6%Al-5%Pb alloy belong to materials that are very susceptible to pitting, whose corrosion potential (φcorr) will be above φpit and the materials will spontaneously pit at the corrosion potential [21]. For Mg-6%Al alloy, the solid solution seems to possess slightly more negative corrosion potential (-1.576 V) and larger corrosion current density (82.88 μA/cm2) than the as-aged state (-1.569 V and 73.24 μA/cm2). When adding both aluminium and lead into magnesium, the φcorr of Mg-Al-Pb alloy falls in between that of single aluminium added and single lead added magnesium alloys, more negative than the former and more positive than the latter while the corrosion current density reaches the largest among three kinds of alloys, expressed as Jcorr (Mg-6%Al-5%Pb) > Jcorr (Mg-6%Al) > Jcorr (Mg-5%Pb). The results signify that the Mg-6%Al-5%Pb alloy has the largest corrosion rate, followed by Mg-6%Al alloy and Mg-5%Pb alloy in descending order. Significantly, it is found that the precipitated second phases in three kinds of alloys slightly inhibit the corrosion but increase the corrosion tendency by providing micro galvanic corrosion sites.
Figure 3 displays the galvanostatic polarization curves of Mg-5%Pb alloy, Mg-6%Al alloy and Mg-6% Al-5%Pb alloy in solid solution and as-aged state at current density of 10 mA/cm2. It can be seen that for three alloys, the solid solution exhibits more negative potential than the as-aged state, manifesting that the elecrochemical activity, i.e., the ability of cavity or crevice propagation resulted by corrosion and corrosion product peeling [22], is higher in solid solution due to its homogeneity and absence of second phases as block. The fact that potential of Mg-6%Al-5%Pb alloy is the most negative in both states than single lead and single aluminium added magnesium alloys indicates that co-existence of Pb and Al can facilitate the corrosion propagation and the falling-off of corrosion product. There are frequent fluctuations on galvanostatic polarization curve of Mg-5%Pb alloy in as-aged state, which is correlated with the occurrence of localised corrosion resulted by second phase and the passivation of corrosion products [5]. Noticeably, obvious activation time is observed in both solid solution and as-aged state of Mg-6%Al alloy while it is nearly negligible for Mg-5%Pb alloy. According to previous literatures [22-23], the activation time in galvanostatic polarization corresponds to the pit propagation stage, in which pit resolves as a groove much like a cavity or a crevice until reaching the dynamic balance between advance of corrosion and the deposition of surface products. The images inserted in Fig. 3 show corroded morphology of corresponding alloy in activation stage. It is found that the Mg-5%Pb alloy displays uniform pit points without further resolution to a cavity while Mg-6%Al alloy and Mg-6%Al-5%Pb alloy both present cavities caused by pit propagation. This can well explain the difference of activation time appearing in each alloy. Additionally, the curves in Fig. 3(c) indicate that the addition of Pb to Mg-6%Al alloy can shorten the activation time and make corrosion proceed steadily. This phenomenon occurs no matter Pb exists in form of atom or Mg2Pb phase. Another result obtained in Fig. 3(c) reveals that the solid solution of Mg-6%Al-5%Pb alloy exhibits a slight passivation trend in contrast to its as-aged state while no similar findings are observed in Mg-5%Pb alloy and Mg-6%Al alloy.
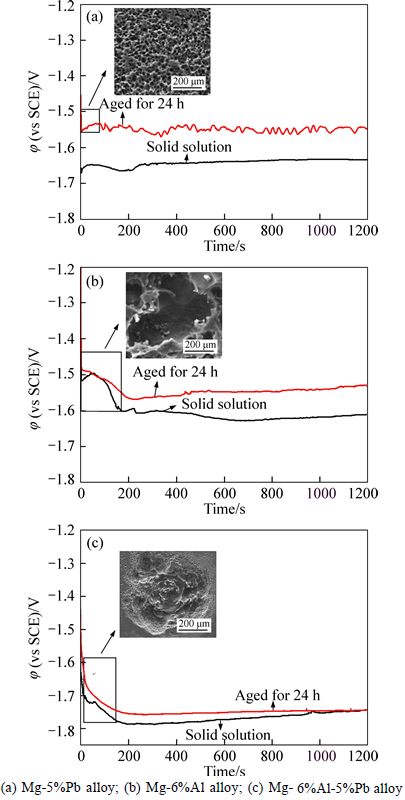
Fig. 3 Galvanostatic polarization curves at current density of 10 mA/cm2:
Figure 4 shows the galvanostatic polarization curves of three experimental alloys at larger current density of 150 mA/cm2. It can be seen that the solid solutions of Mg-5%Pb alloy and Mg-6%Al-5%Pb alloy exhibit obvious passivation trend, which suggests that at large current density, the Cl ions cannot fully react with the PbCl2 film due to high corrosion rate. However, the Mg2Pb phase precipitated in as-aged alloys lightens the degree of passivation, as indicated by Figs. 4(a) and (c). The steady potential states of Mg-6%Al alloy in both solid and as-aged state reveal that the corrosion product 2Mg(OH)2·Al(OH)3 can fake off timely [24], but not passivate the surface of the alloy. At larger current density of 150 mA/cm2, the solid solution of Mg-6%Al alloy shows higher activity than the as-aged state, which is in accordance with that at 10 mA/cm2. For Mg-5%Pb alloy, the as-aged state owns higher activity, opposite to the results at small current density. The reason appears that the localised corrosion resulted by Mg2Pb phase matters more at large current density. Moreover, it is found that the solid solution of each experimental alloy shows more obvious polarization behavior than the as-aged state, which can be interpreted to be due to the facilitation corrosion product flaking off resulted from second phases.
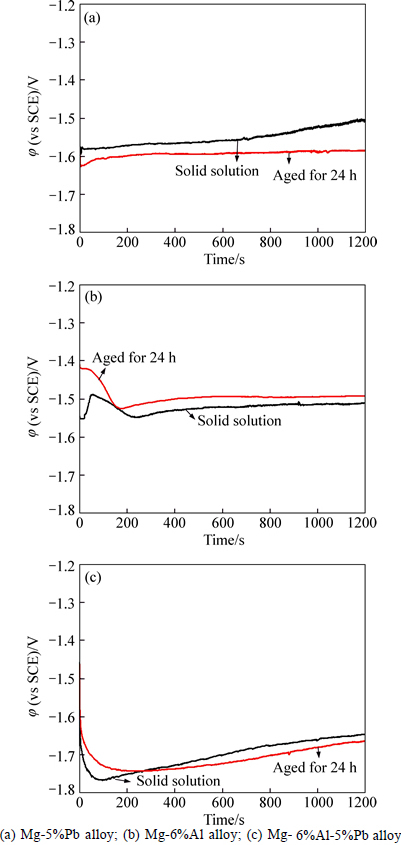
Fig. 4 Galvanostatic polarization curves at current density of 150 mA/cm2:
Figure 5 illustrates the EIS results of Mg-5%Pb alloy, Mg-6%Al alloy and Mg-6%Al-5%Pb alloy measured from 10000 Hz to 0.1 Hz after galvanostatic polarization for 20 min in 3.5% NaCl solution. Moreover, the equivalent circuits are also presented in Fig. 6, signifying the electrochemical process of each experimental alloy more clearly. It can be seen that Mg-5%Pb alloy and Mg-6%Al alloy manifest the same equivalent circuit (Fig. 5(a)) but own different parameter data (Table 1) while the Mg-6%Al-5%Pb alloy exhibits distinctive corrosion model (Fig. 5(b), fitted data in Table 2) compared to the other two experimental alloys. As reported in previous studies [25-26], the high frequency capacitance arc domains the process of electrical double-layer related to charge transfer (Rt and CPEdl), where CPE is a constant phase element substituting for capacitance of electric double layer (C). For Mg-5%Pb alloy and Mg-6%Al alloy, the middle frequency capacitance arc is associated with corrosion product layer, equal to an Cl ion transfer resistance (Rf) and an capacitor (CPEf). Two inductive arcs in low frequency range may be caused by simultaneous process of Cl ions absorption and the pitting relaxation process, i.e., Cl ions gathering in a active hole to proceed corrosion [27], as indicated by equivalent circuit. It is observed that the capacitance arc of Mg-6%Al alloy is larger than that of Mg-5%Pb alloy, while the latter exhibits obvious two inductive arcs in low frequency region, which implies that the corrosion product layer in Mg-5%Pb alloy dissolves more rapidly than Mg-6%Al alloy and the pitting process resulted by Cl ions in this alloy plays prominent role in corrosion. For Mg-6%Al-5%Pb alloy, the middle frequency region depicts an inductive arc related to the transport phenomenon through pores of the film [28] and the low frequency capacitance in middle frequency of arc as well as short inductive arc might be correlated to the desorption of bulk corrosion products, which can be confirmed by the corrosion morphology in Fig. 7.Interestingly, the Nyquist plots and the fitted results demonstrate that solid solutions of each alloy all exhibit smaller parameter values than the as-aged states, meaning that corrosion proceeds more easily in solid solution due to smaller resistance and capacitance.
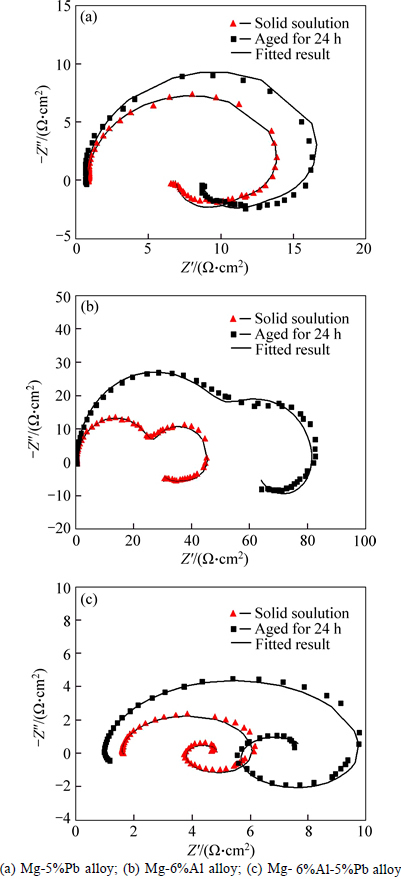
Fig. 5 Electrochemical impedance spectra (EIS):
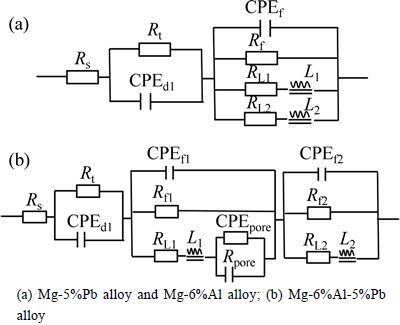
Fig. 6 Equivalent circuits corresponding to EIS results:
Table 1 Electrochemical parameters of Mg-5%Pb alloy and Mg-6%Al alloy obtained by fitting electrochemical impedance spectra
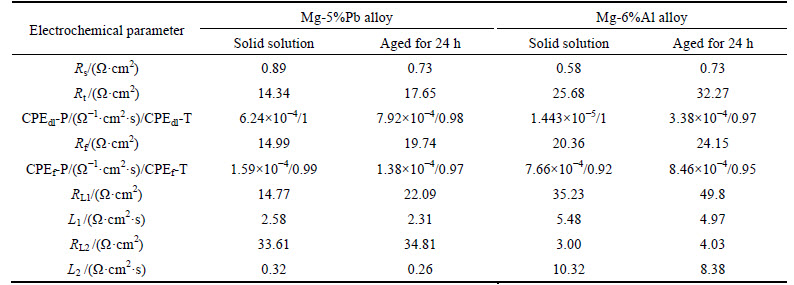
Table 2 Electrochemical parameters of Mg-6%Al-5%Pb alloy obtained by fitting electrochemical impedance spectra
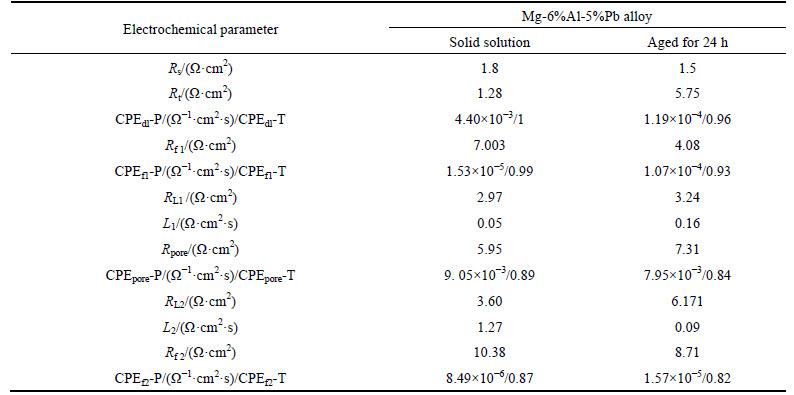
Figure 7 shows the corrosion morphology without corrosion product of Mg-5%Pb alloy, Mg-6%Al alloy and Mg-6%Al-5%Pb alloy after galvanostatic polarization for 20 min at current density of 10 mA/cm2. It is observed obviously that these three experimental alloys exert totally different morphology but the solid solution and the as-aged state of same alloy exhibit similar second electron (SE) images under SEM. For Mg-5%Pb alloy in Figs. 7(a) and (b), porous surface without corrosion product suggests that the advance of corrosion mainly relies on the pitting of Cl ions and the larger holes appearing in the as-aged state are caused by localized corrosion resulted from Mg2Pb phase. It can be seen that corrosion proceeds primarily along the grain boundaries in solid solution of Mg-6%Al alloy while for the as-aged state, corrosion occurs around the precipitated flake-like Mg17Al12 phase and leads to second phase peeling off, which can be verified by small pieces of shallow holes in Fig. 7(e). By comparison, the corroded morphology of Mg-6%Al-5%Pb alloy combines the characteristics of the above two alloys, containing porous structure and shallow holes. The relatively larger shallow holes observed in solid solution might be caused by faking-off of bulk 2Mg(OH)2·Al(OH)3 products and the layered corroded morphology in the as-aged state is correlated to the localized corrosion resulted by lamellar Mg17Al12 phase, which is refined by Pb compared to the flake-like phase in Mg-6%Al alloy.
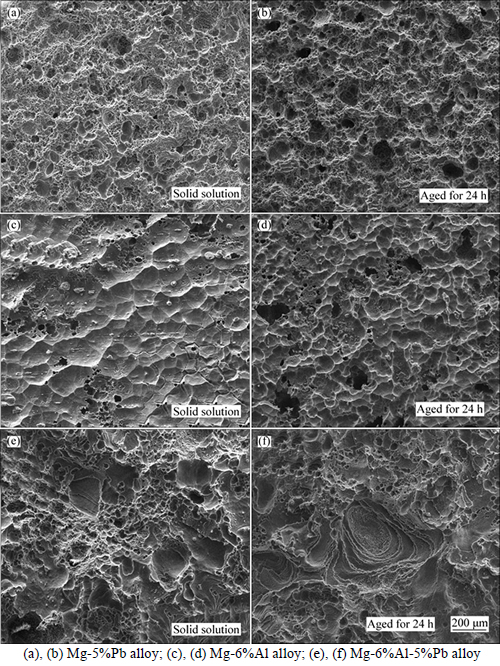
Fig. 7 Secondary electron (SE) images of corroded morphology of experimental alloys after 20 min galvanostatic pilarisation in 3.5% NaCl solution:
4 Conclusions
1) It is identified that Mg2Pb phase exists in Mg-Pb alloy and the addition of Pb to Mg-Al alloy deceases the amount of Mg17Al12 precipitate.
2) The solid solution and the as-aged state of the Mg-5%Pb alloy, Mg-6%Al alloy and Mg-6%Al-5%Pb alloy have the same corrosion mechanism but the solid solution possesses higher electrochemical activity.
3) The Mg-5%Pb alloy exhibits obvious reactivation phenomenon in potentiodynamic polarization test for its corrosion potential (φcorr) is above pitting potential (φpit), while Mg-6%Al alloy and Mg-6%Al-5%Pb alloy display only activation and passivation regions. The corrosion potentials of three alloys in both solid solution and the as-aged state are ranked as φcorr (Mg-5%Pb) > φcorr (Mg-6%Al-5%Pb) > φcorr (Mg-6%Al) and the corrosion currents can be expressed by Jcorr (Mg-6%Al-5%Pb) > Jcorr (Mg-6%Al) > Jcorr (Mg-5%Pb).
4) The Mg-5%Pb alloy shows nearly negligible activation time when it is galvanostatically polarized in current densities of 10 mA/cm2 and 150 mA/cm2, whereas obvious activation stage can be observed in Mg-6%Al alloy. The addition of Pb to Mg-6%Al alloy shortens the activation time.
5) The pitting corrosion is primarily resulted by Cl ions in Mg-5%Pb alloy and corrosion proceeds mainly along the grain boundaries or second phases for Mg-6%Al alloy while it is the combination of both corrosion mechanisms for Mg-6%Al-5%Pb alloy.
References
[1] YANG Ming-bo,LIANG Xiao-feng,YI Zhu, PAN Fu-sheng. Effects of zirconium addition on as-cast microstructure and mechanical properties of Mg-3Sn-2Ca magnesium alloy [J]. Materials and Design, 2011, 32(4): 1967-1973.
[2] SHINOHARA M, ARAKI E, MOCHIZUKI M, KANAZAWA T, SUYEHIRO K. Practical application of a sea-water battery in deep-sea basin and its performance [J]. Journal of Power Sources, 2009,187(1): 253-260.
[3] SONG Guang-ling, ATRENS A, WU Xian-liang, ZHANG Bo. Corrosion behaviour of AZ21, AZ501 and AZ91 in sodium chloride [J]. Corrosion Science, 1998, 40(10): 1769-1791.
[4]
M, DAN P, THIERRY D. Corrosion product formation during NaCl induced atmospheric corrosion of magnesium alloy AZ91D [J]. Corrosion Science, 2007, 49(3): 1540-1558.
[5] WANG Lei, SHINOHARA T, ZHANG Bo-ping. Electrochemical behaviour of AZ61 magnesium alloy in dilute NaCl solutions [J]. Materials and Design, 2012,33: 345-349.
[6] ZHAO Ming-chun, LIU Ming, SONG Guang-ling, ATRENS A. Influence of the b-phase morphology on the corrosion of the Mg alloy AZ91 [J]. Corrosion Science,2008,50(7): 1939-1953.
[7] ARINIVASAN A, PILLAI U, PAI B C. Effect of Pb addition on ageing behavior of AZ91 magnesium alloy [J]. Materials Science & Engineering A, 2007,452(4): 87-92.
[8]
B, SUN Y. A study on the mechanical and corrosion properties of lead added magnesium alloys [J]. Materials and Design,2012,37: 369-372.
[9] WANG Nai-guang, WANG Ri-chu,PENG Chao-qun,FENG Yan, CHEN Bin. Effect of hot rolling and subsequent annealing on electrochemical discharge behavior of AP65 magnesium alloy as anode for seawater activated battery [J]. Corrosion Science, 2012,64(6): 17-27.
[10] WANG Nai-guang, WANG Ri-chu,PENG Chao-qun,FENG Yan. Enhancement of the discharge performance of AP65 magnesium alloy anodes by hot extrusion [J]. Corrosion Science, 2014, 81(12): 85-95.
[11] WANG Nai-guang, WANG Ri-chu, PENG Chao-qun, FENG Yan, ZHANG Xiang-yu. Corrosion behavior of Mg-Al-Pb and Mg-Al-Pb-Zn-Mn alloys in 3.5% NaCl solution [J]. Transaction of Nonferrous Metals Society of China,2010, 20(10): 1936-1943.
[12] SONG Guang-ling. Effect of tin modification on corrosion of AM70 magnesium alloy [J]. Corrosion Science,2009,51(9): 2063-2070.
[13]
K N. Discontinuous and continuous precipitation in magnesium-aluminium type alloys [J]. Journal of Alloys and Compounds, 2009, 447(1/2): 870-876.
[14] LALPOOL M, DZWONCZYK J S, HORT N, OFFERMAN S E. Nucleation mechanism of Mg17Al12-precipitates in binary Mg-7%Al alloy [J]. Journal of Alloys and Compounds, 2013, 557(12): 73-76.
[15] CANDAN S, UNAL M, TURKMEN M, KOC E, TUREN Y, CANDAN E. Improvement of mechanical and corrosion properties of magnesium alloy by lead addition [J]. Materials Science & Engineering A,2009,501(1): 115-118.
[16] CASTRO LUNA A M, MARCHIANO S L, ARVIA A J. Electrochemical formation and cathodic dissolution of a thin cuprous oxide film on copper in alkaline aqueous solution [J]. Journal of Electroanalytical Chemistry and Interfacial Electrochemistry, 1975,59(3): 335-338.
[17] ABD EI REHIM S S, ABD EI HALIM AM, FOAD E E. Potentiodynamic and cyclic voltammetric behaviour of the lead electrode in HCl solutions [J]. Surface Technology. 1983, 18(4): 313-325.
[18] ABD EI AAL E E. Effect of Clanions on zinc passivity in borate solution [J]. Corrosion Science, 2000, 42(1): 1-16.
[19] AAL E, WANEES S. Kinetics of anodic behaviour of Pb in HCl solutions [J]. Corrosion Science,2009,51(3): 458-462.
[20] BARRADAS R G, BELINKO K, GHIBAUDI E. Rotating ring-disc electrode studies of lead in HCl and NaCl Solutions [J]. Canadian Journal of Chemistry, 1975, 53(3): 407-413.
[21] FRANKEL G S. Pitting corrosion of metals [J]. Journal of the Electrochemical Society, 1998, 145(6): 2186-2197.
[22] FRANGINI S, CRISTOFARO N D. Analysis of the galvanostatic polarization method for determining reliable pitting potentials on stainless steels in crevice-free conditions [J]. Corrosion Science, 2003,45(12): 2769-2786.
[23] WILDE B E, WILLIAMS E. The use of current/voltage curves for the study of localized corrosion and passivity breakdown on stainless steels in chloride media [J]. Electrochimica Acta, 1971, 16(11): 1971-1985.
[24] WANG Nai-guang, WANG Ri-chu, PENG Chao-qun, FENG Yan, ZHANG Xiang-yu. Influence of aluminium and lead on activation of magnesium as anode [J]. Transaction of Nonferrous Metals Society of China, 2010, 20(8): 1403-1411.
[25] SONG Ying-wei, SHAN Da-yong, CHEN Rong-shi, HAN En-hou. Corrosion characterization of Mg–8Li alloy in NaCl solution [J]. Corrosion Science, 2009, 51(5): 1087-1094.
[26] HSU C, MANSFELD F. Technical note: concerning the conversion of the constant phase element parameter Y0 into a capacitance [J]. Corrosion, 2001, 57(9): 747-748.
[27] SHAO H B, WANG J M, HE W C, ZHANG J Q, CAO C N. EIS analysis on the anodic dissolution kinetics of pure iron in a highly alkaline solution [J]. Electrochemistry Communications, 2005, 7(12): 1429-1433.
[28] BOISSY C, ALEMANY D, NORMAND B. EIS evaluation of steady-state characteristic of 316L stainless steel passive film grown in acidic solution [J]. Electrochemistry Communications,2013,26(3): 10-12.
(Edited by YANG Bing)
Foundation item: Project supported by 2015 Shandong Provincal Fund for Outstanding Talent Group, China
Received date: 2015-10-27; Accepted date: 2016-03-09
Corresponding author: YU Kun, Professor, PhD; Tel: +86-731-88879341; E-mail: yukun2010@csu.edu.cn