- Abstract:
- 1 Introduction▲
- 2 Experimental▲
- 3 Results and discussion▲
- 4 Conclusions▲
- References
- Figure
- Fig.1 Viscosity of aldehyde-treated erythrocyte suspensions. Control, erythrocyte suspension (50% Hct). Values are mean±SD ( n=7 ) and measured at 37 ℃
- Fig.2 Effect of aldehydes on plasma protein fluorescence (ex. 280 nm/em. 350 nm): (a) Measured immediately; (b) Incubated at 37 ℃ for 48 h.
- Fig.3 Fluorescence (ex. 395 nm/em. 460 nm) resulted from aldehydes treated plasma: (a) Measured immediately; (b) Incubated at 37 ℃ for 48 h.
- Fig.4 Erythrocyte plastic viscosity under different conditions (CK=control, ** P<0.01): (a) Induced by 10 mmol/L MDA; (b) Induced by different GSH; (c) Added different GSH firstly and then MDA; (d) Added MDA firstly and then different GSH. All dates were mean and standard deviation of erythrocyte plastic viscosity under corresponding shear rates (mean±SD, n≥5).
J. Cent. South Univ. Technol. (2008) 15(s1): 540-544
DOI: 10.1007/s11771-008-417-9
Influence of carbonyl stress on rheological alterations of blood materials and decarbonylation effect of glutathione
PENG Mi-jun(彭密军)1, 2, 3, CAI Jian-guang(蔡建光)1, 2, HE Hong(贺 洪)1, 2, GONG Ping(龚 萍)1, 2,
LI Guo-lin(李国林)1, 2, TANG Ting(汤 婷)1, 2, ZHU Ze-rui(朱泽瑞)1, 2, YIN Da-zhong(印大中)1, 2
(1. Key laboratory of Protein Chemistry and Developmental Biology of Ministry of Education, Hunan Normal University, Changsha 410081, China;
2. College of Life Science, Hunan Normal University, Changsha 410081, China;
3. Key laboratory of Hunan Forest Products and Chemical Engineering, Jishou University, Zhangjiajie 427000, China)
Abstract:
The effects of various toxic carbonyls such as malondialdehyde (MDA), a secondary product of lipid peroxidation, and other aldehydes on rheological parameters and their relationship with aging-associated alterations were studied. Both MDA and glutaraldehyde (Glu) in different concentrations significantly increase viscosity, plastic viscosity and yield stress of human plasma and erythrocyte suspensions. MDA (20 mmol/L) reduces sharply the typical fluorescence of proteins (excitation 280 nm/emission 350 nm), and produces age pigment-like fluorescence with a strong emission peak at 460 nm when excites at 395 nm by only being incubated for some hours. In contrast, Glu decreases merely the fluorescence of proteins without producing age pigment-like fluorescence. These data suggest interestingly that the MDA-induced gradual protein cross linking seems to form from different mechanisms compared to the fast rheological changes of blood materials which may take place either in acute and chronic diseases or during aging. On the other hand, MDA induces various deleterious alterations of erythrocytes whereas glutathione (GSH) inhibits the MDA-related carbonyl stress in a concentration-dependent manner. The results indicate that carbonyl-amino reaction exists in the blood widely and GSH has the ability to interrupt or reverse this reaction in a certain way. It implies that carbonyl stress may be one of the important factors in blood stasis and suggests a theoretical and practical approach in anti-stresses and anti-aging.
Key words:
1 Introduction
Reactive aldehydes from various sources, especially from lipid peroxidation, are increasingly understood to be cytotoxic and genotoxic in biological systems[1]. Toxic effects of these aldehydes, so called ‘carbonyl stress’, are responsible for the modification of different biological macromolecular structures and functions by reacting with either SH-groups or amino groups of bio-molecules to form seminercaptals or Schiff bases and lead to various aging-related alterations[2]. The reactive aldehydes generated in these processes may act as “secondary” and “common” toxic messengers of a few biological side-reactions[3-4]. Whereas recent studies have shown that diabetes-related glycation and free radical-associated lipid peroxidation may result in enhanced viscosity, reduced erythrocyte deformability and accompanying MDA accumulation in cellular membranes[5], the abnormality of erythrocyte membranes may thus proposed due to the carbonyl stress related biochemical and/or biophysical changes. A direct experimental proof of the proposal might therefore reveal important molecular information of blood viscositic and microcirculational problems. Also, the correlation of rheological and fluorometrical data between carbonyl stress and physiological stress may help to clarify the possible mechanisms of chronic diseases, such as arteriosclerosis, neuropathy, diabetes etc. and their relation with the formation of age pigments and aging.
2 Experimental
2.1 Preparation of MDA and Glu solution
An MDA stock solution was obtained by hydrolizing 1, 1, 3, 3-tetrameth-oxyprorane (TMP) from Fluka, (Switzerland) according to KIKUGAWA et al[6]. It was then diluted to 0.5 mol/L with tris-HCl (pH=7.4) buffer for storage in a refrigerator for no longer than a week. Glu (25%) solution was purchased from Merck (Germany).
2.2 Preparation of plasma and erythrocyte suspension
Venous blood was drawn from healthy donors with sodium citrate as the anticoagulant (1.5 mg/mL). After centrifugation at 1 500 g for 5 min, the plasma was collected for use. A buffer solution[7] was added to the residual erythrocytes for making an erythrocyte suspension , and the hematocrit (Hct) was 50%.
2.3 Measurement of viscosity and calculation of Casson equation parameters
Digital cone-plate Rheometer (Model DV-Ⅲ, Brookfield Inc. USA) was used to determine the viscosity of samples. Estimation temperature (37 ℃) was controlled by an advanced circulating water bath. Viscosity data was recorded automatically by Rheocalc 32 software (Brookfield Inc. USA). Calculations to obtain the Casson Equation parameters, plastic viscosity and yield-stress, were given by the software[8].
2.4 Measurement of plastic viscosity of erythrocyte under different conditions
GSH was bought from Sigma (USA). Fresh erythrocyte was suspended in HEPES (according to routine) and plasma respectively (40% Hct). Both systems were divided into 4 groups: 1) MDA (10 mmol/L) group; 2) GSH (0.5, 1, 5 mmol/L) group; 3) group added different GSH (0.5, 1, 5 mmol/L)firstly, incubated at 37 ℃ for 15 min then added MDA (10 mmol/L); 4) group added MDA (10 mmol/L) firstly, incubated as above, then, added GSH (0.5, 1, 5 mmol/L). After being incubated for 30 min, all of their plastic viscosities were measured (100-400 s-1).
2.5 Fluorescence spectrophotometry
A spectrofluorometer (LS50-B, Perkin-Elmer Corporation, USA) was used for the measurements. Diluted samples were examined. The excitation (ex.) spectra and the emission (em.) spectra were recorded with an excitation slit width of 5.0 nm and emission slit width of 5.0 nm (or 10.0 nm). Scan speed was 1 500 nm/min.
2.6 Statistics
Results were expressed as the means±standard deviation (SD). Statistical analysis of data was done using statistical software SPSS 8.0 (SPSS Inc, Chicago, IL, USA) and Origin 6.0 Professional (Microcal Software Inc, USA). Linear regression analysis was used to test the association between the biorheologic determinants and fluorescent parameters, and a One-Way analysis of variance (One-Way AVOVA) was used to test the differences of multi-groups. All probability values of less than 5% were considered statistically significant.
3 Results and discussion
3.1 Effects of aldehydes on plasma viscosity
Table 1 shows the changes of plasma viscosity affected by MDA and Glu in different concentrations. Viscosity was measured at different shear rates. It can be found the plasma samples, both with or without MDA and/or Glu, belong to Newtonian fluids and show the same viscosity value under any shear rate[9]. Thus the viscosity averages and standard deviations for each sample are calculated (in Table 1). The results show that higher concentrations of MDA or Glu produce higher viscosities, and Glu at 10 mmol/L almost doubles plasma viscosity (from 1.035 mPa?s to 2.02 mPa?s). Pure water, as a Newtonian fluid, at 37 ℃ shows standard viscosity values (0.695 mPa?s) in this study. Plasma with 20 mmol/L Glu is coagulated and its viscosity is impossible to measure. Therefore only 5 mmol/L and 10 mmol/L Glu were used in the system.
Table 1 Effect of different concentrations of MDA and glutaraldehyde on plasma viscosity ( n = 7)
3.2 Effects of aldehydes on erythrocyte suspensions over Casson Equation parameters
Table 2 shows the effects of MDA and Glu on erythrocyte suspensions calculated via the Casson Equation. Both plastic viscosity and yield stress have higher values in the treated groups than in the controls, and the values increase with the increase of the concentrations of aldehydes. Their confidence of fit is over 90%.
Table 2 Effect of MDA and Glu on viscosity parameters of erythrocyte suspensions
Fig.1 shows the viscosity elevations of MDA and Glu treated erythrocyte suspensions at different shear rates. Viscosities of erythrocyte suspensions increase after treatment with MDA and Glu at different shear rates (P<0.05=.
3.3 Fluorescent changes of blood samples induced by aldehyde treatment
Fluorescent emission of most proteins is mainly
Fig.1 Viscosity of aldehyde-treated erythrocyte suspensions. Control, erythrocyte suspension (50% Hct). Values are mean±SD ( n=7 ) and measured at 37 ℃
contributed by tryptophan residues with an absorption maximum near 280 nm and an emission maximum (in water) occurs at 350 nm, such as shown in Fig.2. Comparing Fig.2(a) and Fig.2(b), it is quite evident that both MDA and Glu bring down the intensities of protein
Fig.2 Effect of aldehydes on plasma protein fluorescence (ex. 280 nm/em. 350 nm): (a) Measured immediately; (b) Incubated at 37 ℃ for 48 h.
fluorescence (280 nm/350 nm). When incubated at 37 ℃ for 48 h, the intensities of protein fluorescence reduce from 268 (20 mmol/L MDA), 310 (10 mmol/L MDA), 285 (10 mmol/L Glu) to 39, 81 and 155, respectively.
On the other hand, before incubation, blood samples show no clear fluorescence emission at 460 nm when excited at 395 nm (as shown in Fig.3(a)). When samples are incubated at 37 ℃ for 48 h, a strong fluorescence peak at 460 nm (ex. 395 nm) is produced only with the MDA treated plasma (Fig.3(b)), in contrast to the control and Glu treated samples. The results indicate that after being treated for 48 h the intensity of relative fluorescence increases by 1500% for 20 mmol/L MDA, however, there is no age pigment-like fluorescence in the Glu group[10-11].
Fig.3 Fluorescence (ex. 395 nm/em. 460 nm) resulted from aldehydes treated plasma: (a) Measured immediately; (b) Incubated at 37 ℃ for 48 h.
3.4 Erythrocyte plastic viscosity under different condition and decarbonylation effect of GSH
The results in Fig.4 show that MDA can cause the obvious increase of plastic viscosity, and GSH has the ability to descend it. From Figs.4(a)-(d), we can see both
Fig.4 Erythrocyte plastic viscosity under different conditions (CK=control, ** P<0.01): (a) Induced by 10 mmol/L MDA; (b) Induced by different GSH; (c) Added different GSH firstly and then MDA; (d) Added MDA firstly and then different GSH. All dates were mean and standard deviation of erythrocyte plastic viscosity under corresponding shear rates (mean±SD, n≥5).
add MDA (or GSH, or two of them) or not, the plastic viscosity of erythrocyte-plasma suspension is higher than that of HEPES one. The one treated by MDA is significantly higher than its control in Fig.4(a). Fig.4(b) clearly shows the concentration-dependent manner of GSH on the decrease of plastic viscosity of erythrocyte suspension. Fig.4(c) and (d) indicate that the sequence of adding MDA and GSH in our experiments has no notable influence on the two suspension systems.
4 Conclusions
1) The increase of viscosity of plasma and erythrocyte suspensions treated by MDA in this study suggest strongly that MDA results in alterations of blood materials, probably by causing change of surface charge of biomolecules. And the crosslinking between carbonyls and amino groups may represent a common process during the formation of age pigment-like fluorescence. Due to the absence of conjugated structure in Glu, it can not induce fluorescent pigments as MDA. But Glu has often been used as a binding agent to biological proteins due to its ability to harden the membranes and cause changes of the membrane fluidity.
2) Both plasma and erythrocyte contained the factors to increase the plastic viscosity. The increase of plastic viscosity induced by MDA can be reduced obviously by GSH. It implies that GSH might interrupt or reverse carbonyl-amino reaction in a certain way, for example, deoxidizes carbonyl-amino reaction products, makes the damage of erythrocyte membrane surface repaired in some degree, etc.
As one of the most studied unsaturated carbonyl products of lipid peroxidation, MDA not only results in crosslinking of membrane proteins to form fluorescent pigments and increasing the plastic viscosity of blood materials, but also carries important molecular information in understanding oxidative stress-related rheological changes in acute and chronic diseases. Something with SH-group like GSH has somewhat decarbonylation effect on blood materials.
References[1] MIYATA T. Alterations of non-enzymatic biochemistry in uremia, diabetes, and atherosclerosis (“carbonyl stress”) [J]. Bull Mem Acad R Med Belg, 2002, 157(3/4): 189-198.
[2] ROBERTS M J, WONDRAK G T, LAUREAN D C, JACOBSON M K, JACOBSON E L. DNA damage by carbonyl stress in human skin cells [J]. Mutat Res, 2003, 522(1/2): 45-56.
[3] BAYNES J W, THORP S R. Glycoxidation and lipoxidation in atherogenesis [J]. Free Radic Biol Med, 2000, 28(12): 1708-1716.
[4] HERMANN E, HELMWARD Z. Methods for determination of aldehydic lipid peroxidation products [J]. Free Radic Biol Med, 1989, 7(2): 197-203.
[5] SUSHIL K J, JEAN D R, GARY J L, JOHEN D. The effect of malonyldialdehyde on viscosity of normal and sickle red blood cells [J]. Biochem Med Motab Bio, 1990, 44(1): 37-44.
[6] KIKUGAWA K, TSUKUDA K, KURECHI T. Studies on peroxidized lipids. Interaction of malondialdehyde with secondary amine and its relevance to nitrosamine formation [J]. Chem Pharm Bull, 1980, 28(11): 3323-3331.
[7] LIU X B, QIN W H, YIN D Z. Biochemical relevance between oxidative/carbonyl stress and elevated viscosity of erythrocyte suspensions [J]. Clinical Hemorheology and Microcirculation, 2004, 31(2): 149-156.
[8] SHI Y D. Shortcoming in blood viscosity and how to overcome it by Casson equation [J]. Chinese J Hemorheol, 1998, (8): 4-7. (in Chinese)
[9] CHUNG T W, HO C P. Changes in viscosity of low shear rates and viscoelastic properties of oxidative erythrocyte suspensions [J]. Clin Hemorheol Microcirc, 1999, 21(2): 99-103.
[10] YIN D Z. Biochemical basis of lipofuscin, ceroid, and age pigment -like fluorophores [J]. Free Radic Biol Med, 1996, 21(6): 871-888.
[11] YIN D Z. Lipofuscin-like fluorophores can result from reactions between oxidized ascorbic acid and glutamine. Carbonyl-protein cross-linking may represent a common reaction in oxygen radical and glycosylation-related ageing processes [J]. Mech Ageing Dev, 1992, 62(10): 35-46.
Foundation item: Project(2007AA02Z433) supported by the National High Technology Research and Development Program of China; Project(2007RS4017) supported by the Postdoctoral Science Foundation of Hunan Province, China
Received date: 2008-06-25; Accepted date: 2008-08-05
Corresponding author: YIN Da-zhong, PhD; Tel: +86-731-8872909; E-mail: pengmj163@163.com, dazhongyin@hotmail.com
- Influence of carbonyl stress on rheological alterations of blood materials and decarbonylation effect of glutathione
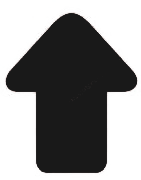