- Abstract:
- 1 Introduction▲
- 2 Materials and expe...▲
- 3 Results and discus...▲
- 4 Conclusions▲
- References
- Figure
- Figure 1 Distributions of precipitates in LMD V-5Cr-5Ti alloys
- Figure 2 Element distributions:
- Figure 3 Distributions of precipitates for samples treated at different solution temperatures:
- Figure 4 Morphologies and distributions of precipitates for samples aged at different temperatures:
- Figure 5 Numbers of precipitates of aged samples treated at different temperatures:(The numbers and densities of precipitates are counted at equal areas for the aged samples)
- Figure 6 Average lengths of precipitates in aged samples
- Figure 7 EDS line scanning result across a precipitate in aged sample treated at 1000 °C
- Figure 8 XRD patterns of LMD sample, the 1560 °C solution sample, and aged samples (a), and aged sample treated at 1000 °C (b)
- Figure 9 Segregation and precipitation behavior of Ti and impurity elements of LMD and heat treated samples:
- Figure 10 Vickers hardness of LMD sample, 1560 °C solution treated sample, and aged samples treated at 800, 1000 and 1200 °C
J. Cent. South Univ. (2021) 28: 1089-1099
DOI: https://doi.org/10.1007/s11771-021-4682-1
Solution and aging behavior of precipitates in laser melting deposited V-5Cr-5Ti alloys
CHAI Peng-tao(柴鹏涛)1, 3, WANG Ye(王烨)1, ZHOU Yu-zhao(周昱昭)1,
YANG Xiao-shan(杨晓珊)1, LI Jin-feng(李晋锋)1, LIU Xue(刘学)1,
LE Guo-min(乐国敏)1, HUANG Xue-fei(黄雪飞)2, YUE Guo-zong(岳国宗)1
1. Institute of Materials, China Academy of Engineering Physics, Mianyang 621908, China;
2. College of Materials Science and Engineering, Sichuan University, Chengdu 610065, China;
3. Shanghai Hangyi Research Institute of High-tech Development, Shanghai 200082, China
Central South University Press and Springer-Verlag GmbH Germany, part of Springer Nature 2021
Abstract:
V-5Cr-5Ti alloys have been fabricated using a laser melting deposition (LMD) additive manufacturing process, showing precipitates aggregated near the grain/dendrite boundaries. Since the mechanical properties of vanadium alloys considerably depend on the precipitates, solution and aging treatments have been applied to eliminating the aggregations of the precipitates. The results show that as the solution temperature increases from 800 to 1560 °C, the densities and the lengths of the precipitates are reduced, while the widths of the precipitates are increased. When the solution temperature reaches 1560 °C, most impurity elements diffuse into the matrix and form into a nearly uniform supersaturated solid solution. Aging treatments have been applied to the 1560 °C solution treated samples. It shows that as the aging temperature increases from 800 to 1200 °C, the precipitate length increases, and the shapes of precipitates change from near-spherical to lath-like. Compared to 800 and 1200 °C, aging at 1000 °C results in the highest precipitate density. Compared to the LMD and solution-treated samples, the aged samples have the highest micro-hardness, due to the precipitation strengthening.
Key words:
vanadium alloy; additive manufacturing; precipitates; solution and aging treatments;
Cite this article as:
CHAI Peng-tao, WANG Ye, ZHOU Yu-zhao, YANG Xiao-shan, LI Jin-feng, LIU Xue, LE Guo-min, HUANG Xue-fei, YUE Guo-zong. Solution and aging behavior of precipitates in laser melting deposited V-5Cr-5Ti alloys [J]. Journal of Central South University, 2021, 28(4): 1089-1099.
DOI:https://dx.doi.org/https://doi.org/10.1007/s11771-021-4682-11 Introduction
V-5Cr-5Ti alloy is considered as one of the most important candidates for structural materials of the future fusion reactor, and has attracted extensive attention due to the excellent properties of neutron irradiation resistance, liquid metal corrosion resistance, mechanical properties at high temperatures, and thermal conductivity [1-6]. The conventional fabrication methods of vanadium alloys include vacuum consumable electrode arc smelting [7, 8], electron beam smelting [8-10], magnetic levitation smelting [11], and powder metallurgy (PM) [12]. However, these methods are often time-consuming, and usually lead to a waste of materials for the fabrications of valuable vanadium alloy components. Therefore, it is necessary to develop new methods to reduce the fabrication cost. Recently, a laser melting deposition (LMD) additive manufacturing technology has been applied to the fabrication of vanadium alloys in our previous work [13], due to the advantages of time saving, single-step fabrication, materials waste-free and etc. [14, 15]. Dense vanadium alloy samples with regular shapes can be prepared successfully using the LMD process. However, the LMD vanadium alloy has rather brittle property, which limits its usage. The brittle property is ascribed to the significant amounts of solute O, C, and N in the vanadium-based matrix preserved during the fast cooling process of LMD, and the segregations of lath-like precipitate clusters near the grain and dendrite boundaries of the vanadium alloys due to the non-equilibrium solidification of the LMD process [13]. Since there is no structural transformation of the BCC structured vanadium-based matrix during the heat treatment processes, vanadium alloys cannot be strengthened by structural transformation of the matrix. Thus the mechanical properties of vanadium alloys considerably depend on the solute elements and the precipitates. A reduction of solute O, C, and N, and a uniform distribution of precipitates can lead to improvements of the mechanical properties [16-22].
The primary precipitates in vanadium alloys are found to be complex Ti-(CNO) compounds, which have a NaCl-type FCC structure, and lath-like or spherical morphologies [18, 20]. The contents of C, N, and O impurity elements of the precipitates are not fixed, which vary with the preparation method and processing environment [23, 24]. The precipitates play roles of precipitation strengthening and purification of matrix impurities for vanadium alloys, which are helpful to improve the tensile strength of the vanadium alloys [25]. However, when the amount of precipitates is too large, it will also greatly reduce the ductility of vanadium alloys [26].
In order to eliminate the aggregations of the precipitate clusters and to reduce the amount of solute elements of O, C, and N in the V-5Cr-5Ti alloys prepared by the LMD process, post solution and aging treatments have been applied to the LMD vanadium alloys. The effects of solution treatment and aging treatment on the size, morphology and distribution of precipitates are studied in this work. Based on the microstructure observations and the micro-hardness tests, the solution and precipitation behavior of the precipitates and strengthening effects at different heat treatment states are analyzed.
2 Materials and experimental process
The V-5Cr-5Ti alloys were prepared using the LMD process with pre-alloyed spherical V-5Cr-5Ti powders. Thin wall plate samples with lengths of 60 mm, and heights of approximately 40 mm were fabricated using a laser power of 1400 W, a scanning speed of 400 mm/min, and a height increment of 0.55-0.6 mm. Details about the powders and the LMD process can be found in Ref. [13]. The chemical composition (in wt %) of the LMD sample is 4.75 Cr, 4.83 Ti, 0.015 C, <0.002 N, 0.925 O, and V balanced. It is noted that the O content is higher than that of the vanadium alloys prepared by the traditional methods, due to the processing of raw powders.
The LMD samples were first isothermally held at different temperatures of 800, 1000, 1200, 1400 and 1560 °C for 1 h and cooled in water to investigate the influence of temperature on the solution of precipitates. Aging treatments were then performed to the 1560 °C solution treated samples at different temperatures of 800, 1000 and 1200 °C for 1 h to investigate the precipitation behavior. The heat treatments were carried out in a high vacuum environment (10-3 Pa). During the heat treatments, samples were wrapped with Zr foils to protect from oxidation.
The specimens for optical microscopy (OM) and scanning electron microscopy (SEM) observations were polished and electrolytic etched with a solution of 5 vol.% sulfuric acid in deionized water at a voltage of 3 V for 10 s. The lengths and the number densities of the precipitates were measured and counted at equal areas of SEM images for aged samples. The number density of the precipitate is calculated as n/A, where n is the number of the precipitates, and A is the area of the SEM image. The micro Vickers hardness tests were conducted on the polished cross-sections of samples under a load of 1000 g and a duration time of 5 s.
3 Results and discussion
3.1 Precipitates in LMD sample
Figure 1 shows the distributions of precipitates in the LMD sample. The precipitates have lath-like shapes. The lengths of precipitates are about a few micrometers. The lath-like precipitates have random orientations, and are distributed in a form of aggregated network near the grain boundaries or the dendrite boundaries. The lath-like precipitates have also been found in vanadium alloys prepared by casting and forging methods, however, showing several parallel sets of lath-like precipitates mainly distributed inside grains, due to a coherent or semi-coherent relationship between the precipitates and the matrix [20, 27, 28]. The coherent/semi-coherent relationships between precipitates and matrix are found to beand
[20]. Compared to the grain boundaries, the precipitates prefer to form inside grains for traditionally prepared vanadium alloys, since it is easier for the precipitates to maintain coherence with matrix if located inside grains [20].
The aggregations of precipitates in the LMD vanadium alloys are formed due to the segregations of Ti, C, N, and O elements near the grain and dendrite boundaries after the non-equilibrium solidification (see Figure 2(a)). Compared to vanadium and chromium elements, the Ti element has a stronger affinity for impurity elements. When the local segregation of elements and compositional fluctuation reach the nucleation conditions of precipitates, the complex Ti-(CNO) compounds are precipitated. However, due to a high atomic chaos near the dendrite and grain boundaries, the precipitates around these boundaries show random orientations.
3.2 Precipitates after solution treatment
Figure 3 shows the distributions of precipitates for samples treated at different solution temperatures. As the solution temperature increases, the densities and the lengths of the precipitates are reduced, while the widths of the precipitates are increased, indicating dissolutions of precipitates into the matrix. When the solution temperature reaches 1560 °C, only a few small sized precipitates are observed near the dendrite/gain boundaries. It is noted that the micro-sized pores are caused by severer corrosions near the precipitates, which wash out the precipitates and round the edges of the voids during the electropolishing process [29].
Figure 1 Distributions of precipitates in LMD V-5Cr-5Ti alloys
Figure 2 Element distributions:
Figure 3 Distributions of precipitates for samples treated at different solution temperatures:
Since there are many supersaturated Ti and impurity elements near the dendrite/grain boundaries in the LMD sample as discussed above, these elements will diffuse to the matrix under the high temperature heating. As the temperature increases from 800 to 1560 °C, the segregated elements diffuse further into the matrix, and form into a uniform supersaturated solid solution after quenching in water (see Figure 2(b)). It is noticed that the supersaturated elements near the dendrite/grain boundaries do not precipitate during the heating process, which may be attributed to two possible reasons. One reason might be the vacancy depletion in the vicinity of dendrite/grain boundaries, which leads to precipitate free zones (PFZ) by the inhibition of precipitation and supersaturation of local elements [30]. Another possible reason might be the lack of coherent/semi-coherent sites near the dendrite/grain boundaries, which provides high energy barriers for the precipitation.
HEO et al [24] have found that the precipitates of vanadium alloy prepared by traditional methods begin to dissolve into the matrix at a solution temperature of 1000 °C. And when the solution temperature reaches about 1100 °C, the precipitates can dissolve completely into the matrix [31]. Compared with the vanadium alloy prepared by traditional methods, the LMD vanadium alloy needs a higher solution temperature. The higher solution temperature of the LMD sample may be related to the higher contents of impurity elements.
3.3 Precipitates after aging treatments
The microstructures of the aged samples at different temperatures are shown in Figure 4. A large number of precipitates are reformed both inside the grains and near the grain boundaries, showing a uniform distribution. Thus, the aggregations of precipitates in the LMD sample are eliminated by proper solution and aging treatments. Precipitates in the 800 °C aged sample (see Figure 4(a)) mainly have near-spherical shapes. However, a few precipitates also have lath-like shapes. After aged at 1000 °C (see Figure 4(b)), more lath-like precipitates are formed, while the near-spherical precipitates almost disappear. After aged at 1200 °C (see Figure 4(c)), all the precipitates have lath-like shapes, and the distribution of the lath-like precipitates is much more organized, showing two sets of paralleled laths. Compared to the LMD and the solution treated samples, more and larger precipitates are formed after the aging treatments. The numbers of precipitates with different lengths were then counted for the aged samples at equal areas, and the results are shown in Figure 5. It shows that the number density of the precipitates is the highest for aging at 1000 °C, compared to aging at 800 °C and 1200 °C (see Figure 5(d)). The lengths of the near-spherical and lath-like precipitates also vary with aging temperatures, showing an increase with the increase of the aging temperature (see Figure 6).
To verify the composition of the precipitates, EDS mapping and line scanning tests were applied to the precipitates in the sample aged at 1000 °C (see Figures 2(c) and 7). It can be clearly seen that the precipitates are Ti-(CNO) compounds, as found in many V-Cr-Ti alloys prepared by other methods [18, 20, 23, 24].
Numerous studies have reported that the Ti-(CNO) compound has a NaCl-type FCC structure [18, 20]. XRD test has been applied to identify the phase of the precipitates, as shown in Figure 8. However, due to the limited volume fractions, the peaks of the precipitates are hard to detect. Only the slower scanning result from 35° to 43° of the 1000 °C aged sample, which has the highest density of precipitates, shows one peak of the FCC structured Ti-(CNO) compound (see Figure 8(b)).
Figure 4 Morphologies and distributions of precipitates for samples aged at different temperatures:
The precipitation behavior can be explained as follows. After the solution treatment at 1560 °C, the alloying elements of Ti and Cr, and the impurity elements of C, N, and O are supersaturated nearly uniformly inside the grains, as indicated in Figure 2(b). During the aging process, three stages including nucleation, growth and coarsening take place during the precipitation of second phase in the supersaturated solid solution [32]. The density of precipitates then depends on the nucleation rate N, which can be calculated using Eq. (1) according to the diffusional transformation theory in solids [33]:
Figure 5 Numbers of precipitates of aged samples treated at different temperatures:(The numbers and densities of precipitates are counted at equal areas for the aged samples)
Figure 6 Average lengths of precipitates in aged samples
Figure 7 EDS line scanning result across a precipitate in aged sample treated at 1000 °C
Figure 8 XRD patterns of LMD sample, the 1560 °C solution sample, and aged samples (a), and aged sample treated at 1000 °C (b)
(1)
where N0 is a factor related to the number of atoms per unit volume in the phase; the vibration frequency of the atoms, and the area of the critical nucleus; k is the Boltzmann constant; T is the temperature; △G* is the activation energy barrier, which strongly depends on T; and △Gm is the activation energy for atomic migration, which is constant. The term exp(-△G*/kT) is essentially the potenial concentration of nuclei, which rises rapidly as T decreases when a critial undercooling is reached. The other term, exp(-△Gm/kT) is essentially the atomic mobility, which decreases rapidly with decreasing temperature. Thus, the combination of these terms leads to a maximum nucleation rate at intermediate undercoolings. As in the present samples, compared to 800 °C and 1200 °C, aging at 1000 °C has the maximum nucleation rate.
After nucleation, the degrees of aggregations of impurity and Ti elements increase as the aging temperature increases, resulting in the growth and coarsening of precipitates into the lath-like shape. It is concluded that the distributions, quantities, sizes and shapes of precipitates can be effectively controlled by the aging treatments.
The segregation and precipitation behavior of impurity elements and Ti element of the LMD and heat-treated samples are then concluded as shown in Figure 9. During the LMD process, the vanadium-based grains nucleate and grow into dendrites. Due to a high temperature gradient and the related constitutional undercooling, elements such as C, N, O and Ti are enriched near the grain/dendrite boundaries. When the molten pool starts to cool down, some impurity elements interact with Ti element, and form into precipitates. Due to a high atomic chaos near the dendrite and grain boundaries, the precipitates show a nearly random distribution. Some Ti and impurities elements are retained as solute atoms near the dendritic boundaries due to the high cooling rate. The LMD samples thus consist of relative-pure vanadium-based dendrites, which have fewer dissolved Ti and impurity elements, and highly alloy-riched dendrite boundaries, which have a large amount of dissolved Ti and impurities elements, and a few precipitates (see Figure 9(a)). The impurity and Ti elements are only able to diffuse in vanadium matrix when the temperature is higher than 300 °C and 500 °C, respectively [32, 33]. When the solution temperature reaches 1560 °C, most Ti and impurity elements are dissolved into vanadium matrix and diffuse from the dendrite boundaries to the grain interior driven by the concentration differences, leading to the disappearance of dendrite boundaries. Thus the segregation of elements is eliminated after solution treatment, and a uniform supersaturated solid solution is formed (see Figure 9(b)). During the aging treatments, the C, N and O interstitial solutes react with Ti, and form into precipitates uniformly distributed inside grains (see Figure 9(c)).
3.4 Micro hardness
The micro hardness of different samples is shown in Figure 10. The micro hardness of the LMD sample first decreases after solution treated at 1560 °C, and then increases dramatically after subsequent aging treatments. However, the micro hardness decreases as the aging temperature increases from 800 °C to 1000 °C. In general, the hardness of the aged samples is higher than that of the LMD sample and the solution treated sample.
Figure 9 Segregation and precipitation behavior of Ti and impurity elements of LMD and heat treated samples:
Figure 10 Vickers hardness of LMD sample, 1560 °C solution treated sample, and aged samples treated at 800, 1000 and 1200 °C
For vanadium alloys, the solution and precipitation behavior of alloying and impurity elements bring two main strengthening effects: solution strengthening and precipitation strengthening. Grain sizes and the dislocation densities can also affect the hardness of the vanadium alloys. The solution heat treatments at high temperatures should cause an increase of grain size, a decrease of dislocation density, and dissolutions of precipitates, which lead to the decrease of hardness. Since the following aging treatments should have minor effects on the grain sizes and dislocation densities, the strengthening of the aged samples are mainly caused by the precipitation strengthening, which agrees with many reported works showing that the precipitation strengthening is stronger than the solution strengthening for vanadium alloys [22, 24, 34]. In this study, the solution sample has the lowest hardness due to the limited precipitation strengthening. The LMD sample has local solution and precipitation strengthening near the dendrite boundaries, thus has the moderate hardness due to the combination of solution and precipitation strengthening. The aged samples have the highest hardness due to the strong precipitation strengthening.
It is noticed that although the 1000 °C aged sample has the highest density of precipitates, it does not have the highest hardness. This abnormal hardness is attributed to the morphologies of precipitates. Compared to the spherical shapes in the 800 °C aged sample, the precipitates in the 1000 °C and 1200 °C aged samples mainly have lath-like shapes. It has been found that the lath-like precipitates separate the grains into isolated domains, only within which dislocations can glide freely, leading to reduced possibilities for the cross gliding and interactions of dislocations (i.e., reduced strengthening effect). And the higher the density of the precipitates, the stronger the effect [19]. Thus for the aged samples, the 1000 °C aged sample with the highest density of lath-like precipitates has the lowest hardness. Although the density of lath-like precipitates decreases for the 1200 °C aged sample, the coarsening of the lath-like precipitates finally results in the comparable hardness with the 1000 °C aged sample.
4 Conclusions
In this study, solution and aging treatments at different temperatures have been applied to the vanadium alloys prepared by LMD process. The solution and aging behavior of the LMD vanadium alloys, as well as the strengthening effects have been studied. The main conclusions are as follows:
1) The aggregations of the precipitates in the LMD samples can be modified by solution and aging treatments. When the solution temperature reaches 1560 °C, most of the precipitates can be dissolved into the matrix. The high solution temperature is probably due to the high contents of impurity elements.
2) The precipitate length increases as the aging temperature increases from 800 °C to 1200 °C. Compared to 800 °C and 1200 °C, aging at 1000 °C results in the highest precipitate density. More lath-like precipitates are formed at high aging temperatures than the near-spherical precipitates.
3) The aged samples have higher micro hardness compared to the LMD and solution samples, due to the precipitation strengthening. For the aged samples, the sample with the highest density of lath-like precipitates has the lowest hardness.
Contributors
The overarching research goals were developed by LE Guo-min, HUANG Xue-fei, and YUE Guo-zong. CHAI Peng-tao, WANG Ye, and ZHOU Yu-zhao provided the measured data, and analyzed the measured data. YANG Xiao-shan, LI Jin-feng, and LIU Xue analyzed the calculated results. The initial draft of the manuscript was written by CHAI Peng-tao. LE Guo-min, HUANG Xue-fei, and YUE Guo-zong edited the draft of manuscript. All authors replied to reviewers’ comments and revised the final version.
Conflict of interest
CHAI Peng-tao, WANG Ye, ZHOU Yu-zhao, YANG Xiao-shan, LI Jin-feng, LIU Xue, LE Guo-min, HUANG Xue-fei, and YUE Guo-zong declare that they have no conflict of interest.
References
[1] SATOU M, ABE K, KAYANO H. High-temperature deformation of modified V-Ti-Cr-Si type alloys [J]. Journal of Nuclear Materials, 1991, 179(1): 757-761. DOI: https://doi. org/10.1016/0022-3115(91)90199-H.
[2] DIERCKS D R, LOOMIS B A. Alloying and impurity effects in vanadium-base alloys [J]. Journal of Nuclear Materials, 1986, 141: 1117-1124. DOI: https://doi.org/10.1016/0022-3115(86)90152-2.
[3] HEO N J, NAGASAKA T, MUROGA T. Recrystallization and precipitation behavior of low-activation V–Cr–Ti alloys after cold rolling [J]. Journal of Nuclear Materials, 2004, 325(1): 53-60. DOI: https://doi.org/10.1016/j.jnucmat. 2003.10.012.
[4] SMITH J P, JOHNSON W R, TRESTER P W. Metallurgical bonding development of V-4Cr-4Ti alloy for the DIII-D radiative divertor program [J]. Journal of Nuclear Materials, 1998, 258: 1420-1424. DOI: https://doi.org/10.1016/S0022-3115(98)00323-7.
[5] BOEV A O, AKSYONOV D A, KARTAMYSHEV A I, MAKSIMENKO V N, NELASOV I V, LIPNITSKII A G. Interaction of Ti and Cr atoms with point defects in bcc vanadium: A DFT study [J]. Journal of Nuclear Materials, 2017, 492(8): 14-21. DOI: https://doi.org/10.1016/j.jnucmat. 2017. 04.046.
[6] BARRON P J, CARRUTHERS A W, FELLOWES J W, JONES N G, PICKERING E J. Towards V-based high-entropy alloys for nuclear fusion applications [J]. Scripta Materialia, 2020, 176: 12-16. DOI: 10.1016/j.scriptamat.2019.09.028.
[7] SHIKOV A K, CHERNOV V M, POTAPENKO M M, GUBKIN I N, DROBYSHEV V A, ZURABOV V S. Development of production process and study of low-activity V-(4–5)%Ti-(4–5)%Cr structural alloys for thermonuclear reactors [J]. Metal Science & Heat Treatment, 2004, 46(11, 12): 497-503. DOI: https://doi.org/10.1007/s11041-005-0009-3.
[8] POTAPENKO M M, DROBISHEV V A, FILKIN V Y, GUBKIN I N, MYASNIKOV V V, NIKULIN A D, SHINGAREV E N, VEDERNIKOV G P, VOTINOV S N, ZURABOV V S, ZOLOTAREV A B. Manufacture of semifinished items of alloys V-4Ti-4Cr and V-10Ti-5Cr for use as a structural material in fusion applications[J]. Journal of Nuclear Materials, 1996, 233-237: 438-441. DOI: https://doi.org/10.1016/S0022-3115(96)00285-1.
[9] MUROGA T, NAGASAKA T, IIYOSHI A, KAWABATA A, SAKATA M. NIFS program for large ingot production of a V–Cr–Ti alloy [J]. Journal of Nuclear Materials, 2000, 283-287: 711-715. DOI: https://doi.org/10.1016/S0022-115(00)00281-6.
[10] NAGASAKA T, MUROGA T, FUKUMOTO K I, WATANABE H, GROSSBECK M L, CHEN Ji-ming. Development of fabrication technology for low activation vanadium alloys as fusion blanket structural materials [J]. Nuclear Fusion, 2006, 46(5): 618-625. DOI: https:// iopscience.iop.org/article/10.1088/0029-5515/46/5/012/meta.
[11] CHUTO T, SATOU M, HASEGAWA A, ABEA K, NAGASAKAB T, MUROGAB T. Fabrication using a levitation melting method of V-4Cr-4Ti-Si-Al-Y alloys and their mechanical properties [J]. Journal of Nuclear Materials, 2002, 307(1): 555-559. DOI: https://doi.org/10.1016/S0022-3115(02)01089-9.
[12] KUWABARA T, KURISHITA H, HASEGAWA M. Development of an ultra-fine grained V–1.7mass% Y alloy dispersed with yttrium compounds having superior ductility and high strength [J]. Materials Science & Engineering A, 2006, 417(1, 2): 16-23. DOI: https://doi.org/10.1016/S0022-3115(02)01089-9.
[13] BAI Lin-rui, LE Guo-min, LIU Xue, LI Jin-feng, XIA Sheng-quan, LI Xiu-yan. Grain morphologies and microstructures of laser melting deposited V-5Cr-5Ti alloys [J]. Journal of Alloys & Compounds, 2018, 745: 716-724. DOI: https://doi.org/ 10.1016/j.jallcom.2018.02.249.
[14] XIA Yang, DONG Zhao-wang, GUO Xue-yi, TIAN Qing-hua, LIU Yong. Towards a circular metal additive manufacturing through recycling of materials: A mini review [J]. Journal of Central South University, 2020, 27(4): 1134-1145. DOI: https://doi.org/10.1007/s11771-020-4354-6.
[15] YANG Li-ning, SHAN Zhong-de, RONG Wen-juan, LIU Feng, WANG Yong-wei. Three-dimensional direct writing technology of low melting point molten metal [J]. Journal of Central South University (Science and Technolgy), 2018, 49(10): 47-54. DOI: http://10.11817/ j.issn.1672-7207. 2018.10.006. (in Chinese)
[16] GELLES D S, STUBBINS J F. Microstructural development in irradiated vanadium alloys [J]. Journal of Nuclear Materials, 1994, 212(part-P1): 778-783. DOI: https://doi.org/10.1016/ 0022-3115(94)90162-7.
[17] GELLES D S, RICE P M, ZINKLE S J, CHUNG H M. Microstructural examination of irradiated V-(4-5%)Cr-(4-5%)Ti [J]. Journal of Nuclear Materials, 1998, 258-263: 1380-1385. DOI: https://doi.org/10.1016/S0022-3115(98)00 206-2.
[18] MUROGA T, HEO N J, NAGASAKA T, WATANABE H, NISHIMURA A, SHINOZAKI K. Heterogeneous precipitation and mechanical property change by heat treatments for the laser weldments of V-4Cr-4Ti alloy [J]. Plasma & Fusion Research, 2015, 10: 1405092. DOI: https://doi.org/10.1585/pfr.10.1405092.
[19] ZHU Bo-ling, YANG Shan-wu, DING Jian-wen, ZHANG Wen-hua, LONG Yi, WAN Fa-rong. Abnormal hardening effect induced by the lath-like precipitates in the V–4Cr–4Ti alloy [J]. Materials Letters, 2015, 161: 609-612. DOI: https://doi.org/10.1016/j.matlet.2015.09.080.
[20] ZHU Bo-ling, YANG Shan-wu, ZHANG Meng-qi, DING Jian-wen, LONG Yi, WAN Fa-rong. Formation and evolution of platelet-like Ti-rich precipitates in the V–4Cr–4Ti alloy [J]. Mater Charact, 2016, 111: 60-66. DOI: https://doi.org/10. 1016/j.matchar.2015.11.012
[21] ZINKLE S J, MATSUI H, SMITH D L, ROWCLIFFE A F, VAN O E, ABE K, KAZAKOV V A. Research and development on vanadium alloys for fusion applications [J]. Journal of Nuclear Materials, 1998, 258-263(4): 205-214. DOI: https://doi.org/10.1016/S0022-3115(98)00269-4.
[22] HOELZER D T, WEST M K, ZINKLE S J, ROWCLIFFE A F. Solute interactions in pure vanadium and V–4Cr–4Ti alloy [J]. Journal of Nuclear Materials, 2000, 283-287: 616-621. DOI: https://doi.org/10.1016/S0022-3115(00)00344-5.
[23] LI Zeng-de, LIN Chen-guang, CUI Shun. Behavior of secondary phase of As-cast V-5Cr-5Ti alloy [J]. Rare Metal Mat Eng, 2017, 46(1): 104-110. DOI: http://www.cqvip. com/QK/92850X/201701/671192524.html. (in Chinese)
[24] HEO N J, NAGASAKA T, MUROGA T. Recrystallization and precipitation behavior of low-activation V–Cr–Ti alloys after cold rolling [J]. Journal of Nuclear Materials, 2004, 325(1): 53-60. DOI: https://doi.org/10.1016/j.jnucmat.2003. 10.012.
[25] MUROGAA T, CHEN J M, CHERNOV V M, KURTZ R J, FLEM M L. Present status of vanadium alloys for fusion applications [J]. Journal of Nuclear Materials: Materials Aspects of Fission and Fusion, 2014, 455(1-3): 263-268. DOI: https://doi.org/10.1016/j.jnucmat.2014.06.025.
[26] NAGASAKA T, HEO N J, MUROGA T, NISHIMURA A, WATANABE H, NARUI M, SHINOZAKI K. Impact properties of NIFS-HEAT-2 (V–4Cr–4Ti) after YAG laser welding and neutron irradiation at 563 K [J]. Journal of Nuclear Materials, 2004, 329-333: 1539-1543. DOI: https://doi.org/10.1016/j.jnucmat.2004.04.171.
[27] NAGASAKA T, HEO N J, MUROGA T, IMAMURA M. Examination of fabrication process parameters for improvement of low-activation vanadium alloys [J]. Fusion Engineering and Design, 2002, 61-62: 757-762. DOI: https://doi.org/10.1016/S0920-3796(02)00258-2.
[28] DING Jian-wen, YANG Shan-wu, LIU Guo-liang, LI Qiu-fan, ZHU Bo-ling, ZHANG Meng-qi, ZHOU Lu-jun, SHANG Cheng-jia, ZHAN Qian, WAN Fa-rong. Recrystallization nucleation in V-4Cr-4Ti alloy [J]. Journal of Alloys and Compounds, 2018, 777: 663-672. DOI: https://doi.org/10. 1016/j.jallcom.2018.11.014.
[29] BUEHLER. Buehler SumMet-A guide to materials preparation & analysis [M]. 2nd edition. Germany: Buehler, 2013.
[30] HIROSAWA S, OGURI Y, OGURA T, SATO T. Formation mechanisms of precipitate free zones in age-hardenable Al alloys [J]. Material Forum, 2004: 666-671. DOI: http://www. icaa-conference.net/ICAA9/data/papers/GP%2091.pdf.
[31] NISHIMURA A, IWAHORI A, HEO N J, NAGASAKA T,MUROGA T, TANAKA S I. Effect of precipitation and solution behavior of impurities on mechanical properties of low activation vanadium alloy [J]. Journal of Nuclear Materials, 2004, 329: 438-441. DOI: https://doi.org/ 10.1016/j.jnucmat.2004.04.072.
[32] GRONG O, SHERCLIFF H R. Microstructural modelling in metals processing [J]. Progress in Materials Science, 2002, 47(2): 163-282. DOI: https://doi.org/10.1016/S0079-6425(00) 00004-9.
[33] PORTER D A, EASTERLING K E, SHERIF M. Phase transformations in metals and alloys [M]. Boca Raton: CRC Press, 2009.
[34] CHEN J M, MUROGA T, NAGASAKA T, QIU S Y, LI C, CHEN Y, LIANG B, XU Z Y. The mechanical properties of V–4Cr–4Ti in various thermo-mechanical states [J]. Fusion Engineering & Design, 2006, 81(23-24): 2899-2905. DOI: https://doi.org/10.1016/j.fusengdes.2006.07.051.
(Edited by HE Yun-bin)
中文导读
激光熔化沉积V-5Cr-5Ti合金第二相的固溶和时效行为
摘要:使用激光熔化沉积(LMD)技术制备的V-5Cr-5Ti合金中存在大量偏析分布在晶界/枝晶界的第二相。由于钒合金基体无法通过热处理进行相变强化,其力学性能主要取决于第二相,因此十分有必要研究如何采用固溶和时效热处理来消除LMD钒合金中的第二相偏析。研究结果表明,随着固溶温度从800 °C升至1560 °C,LMD钒合金中的第二相的长度和密度减小而其宽度增加。当固溶温度达到1560 °C,大部分杂质元素可扩散进基体中并形成近乎均匀分布的过饱和固溶体。对1560 °C固溶样品进行时效处理,结果表明,随着时效温度从800 °C升至1200 °C,第二相的长度增加,且第二相的形状由近球形逐渐向条状转变。与800 °C和1200 °C相比,1000 °C时效处理形成的第二相密度最高。由于存在第二相的析出强化效果,与LMD样品和固溶状态样品相比,时效样品具有最高的硬度。
关键词:钒合金;增材制造;第二相;固溶时效
Foundation item: Project(51871203) supported by the National Natural Science Foundation of China; Project(TZ2018006-0303-02) supported by the Science Challenge Project, China; Projects(2020ZDZX0017, 2019YFG0217) supported by the Sichuan Science and Technology Program, China
Received date: 2020-11-23; Accepted date: 2021-03-09
Corresponding author: YUE Guo-zong, PhD, Associate Researcher; Tel: +86-28-65726375; E-mail: yueguozong@163.com; ORCID: https://orcid.org/0000-0001-9046-157X
Abstract: V-5Cr-5Ti alloys have been fabricated using a laser melting deposition (LMD) additive manufacturing process, showing precipitates aggregated near the grain/dendrite boundaries. Since the mechanical properties of vanadium alloys considerably depend on the precipitates, solution and aging treatments have been applied to eliminating the aggregations of the precipitates. The results show that as the solution temperature increases from 800 to 1560 °C, the densities and the lengths of the precipitates are reduced, while the widths of the precipitates are increased. When the solution temperature reaches 1560 °C, most impurity elements diffuse into the matrix and form into a nearly uniform supersaturated solid solution. Aging treatments have been applied to the 1560 °C solution treated samples. It shows that as the aging temperature increases from 800 to 1200 °C, the precipitate length increases, and the shapes of precipitates change from near-spherical to lath-like. Compared to 800 and 1200 °C, aging at 1000 °C results in the highest precipitate density. Compared to the LMD and solution-treated samples, the aged samples have the highest micro-hardness, due to the precipitation strengthening.
- Solution and aging behavior of precipitates in laser melting deposited V-5Cr-5Ti alloys
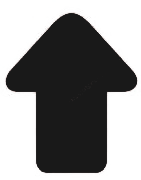