- Abstract:
- 1 Introduction▲
- 2 Experimental▲
- 3 Results and discus...▲
- 3.1 Diffuse reflectance spectra (DRS) of eosin/TiO2
- 3.2 Effect of mass ratio of eosin to TiO2 on photocatalytic activity of eosin/TiO2 under visible light irradiation
- 3.3 Effect of oxygen on photodegradation of phenol under visible light irradiation
- 3.4 Formation of singlet oxygen (1O2) in eosin/TiO2 system under visible light irradiation
- 3.5 Formation of hydroxyl radical (·OH) in eosin/TiO2 system under visible light irradiation
- 3.6 Formation of hydrogen peroxide (H2O2) in eosin/TiO2 system under visible light irradiation
- 3.7 mechanism of photocatalytic degradation of phenol process using eosin sensitized TiO2 under visible light irradiation
- 4 conclusions▲
- References
- Figure
- Fig. 1 Diffuse reflectance spectra of TiO2 and eosin/TiO2
- Fig. 2 Effect of mass ratio of eosin to TiO2 on photocatalytic activity of eosin/TiO2
- Fig. 3 effect of O2 on photodegradation of phenol under visible light irradiation
- Fig. 4 effect of NaN3 on photodegradation of phenol under visible light irradiation
- Fig. 5 absorbancy in eosin/TiO2 system under different conditions
- Fig. 6 effect of isopropanol on photodegradation of phenol under visible light irradiation
- Fig.7 absorbancy in eosin/TiO2 system under different conditions
J. Cent. South Univ. (2013) 20: 495–500
DOI: 10.1007/s11771-013-1511-1
Formation of active radicals and mechanism of photocatalytic degradation of phenol process using eosin sensitized TiO2 under visible light irradiation
SONG Lin(宋琳)1, 2, ZHANG Xin(张鑫)1, 2, MA Yun-kun(马云坤)1, 2,
LI Ming-yu(李明玉)1, 2, ZENG Xiao-long(曾小龙)1, 2
1. Department of Environmental Engineering, Jinan University, Guangzhou 510632, China;
2. Key Laboratory of Water/Soil Toxic Pollutants Control and Bioremediation,
Department of Education of Guangdong Province, Guangzhou 510632, China
Central South University Press and Springer-Verlag Berlin Heidelberg 2013
Abstract:
The role of oxygen and the generation of active radicals in the photocatalitic degradation of phenol were investigated using the eosin sensitized TiO2 as photocatalyst under visible light irradiation. Diffuse reflectance spectra show that the absorbancy range of eosin/TiO2 is expanded from 378 nm (TiO2) to about 600 nm. The photocatalitic degradation of phenol is almost stopped when the eosin/TiO2 system is saturated with N2, which indicates the significance of O2. The addition of NaN3 (a quencher of single oxygen) causes about a 62% decrease in the phenol degradation. The phenol degradation ratio is dropped from 92% to 75% when the isopropanol (a quencher of hydroxyl radical) is present in the system. The experimental results show that there are singlet oxygen and hydroxyl radical generated in the eosin/TiO2 system under visible light irradiation. The changes of absorbancy indicate that the hydrogen peroxide might be produced. Through the analysis and comparison, it is found that the singlet oxygen is the predominant active radical for the degradation of phenol.
Key words:
eosin sensitized TiO2; visible light; photocatalytic degradation; reaction mechanism;
1 Introduction
In recent years, it is of great interest that TiO2 is used as photocatalyst to eliminate environmental pollutants [1–3]. However, pure TiO2 has a bandgap of 3.0–3.2 eV, which is too wide to absorb visible light and limits its application on sunlight[4–7]. Therefore, many ways to modify TiO2 for visible light activity have been reported [8–11]. The surface sensitization as an important method has attracted considerable attention. Surface sensitization means the sensitizer which could absorb visible light was absorbed on the surface of photocatalyst through chemical absorption or physical absorption. The photocatalyst sensitized would be of visible light activity. The sensitizers usually are organic dyes, chlorophyll, humic acid, fulvic acid, unsaturated fatty acid, etc. [8]. The eosin is an excellent sensitizer and the photocatalytic reaction can be carried out smoothly under visible light using eosin sensitized TiO2. However, most researches of eosin/TiO2 photocatalyst mainly focused on the photocatalytic effects. The formation of active radicals and which radicals play the most significant role in the photocatalytic process of eosin/TiO2 system have hardly been reported. Because the degradation of pollutants is mainly dependent on strong oxidation of various active radicals, the research on the formation of active radicals will be significant for TiO2 photocatalytic technology. In addition to that, the details of the mechanisms of photocatalytic reaction processes at the TiO2 surface remain elusive [12]. The major uncertain issue is the reaction pathway. Some researchers suggested that oxidation processes of pollutants were attributed to positive holes, while others have suggested a hydroxyl radical-mediated pathway [13]. Therefore, it is necessary to discuss the mechanism of photocatalytic reaction processes in eosin/TiO2 system. In order to find out the formation of active radicals and the mechanism of eosin/TiO2 for the degradation of pollutants, the photocatalitic degradation of phenol using the eosin modified TiO2 was studied under visible light irradiation.
2 Experimental
2.1 Materials
TiO2, with a crystallographic mode of 80% anatase and 20% rutile, a BET surface area of 50 m2/g and an average particle size of 30 nm, was of supplied by Degussa Co. All other chemicals were of analytical grade and used without further purification. Distilled water was used throughout the experiment.
2.2 Eosin/TiO2 preparation
A mixture composed of eosin (10 mg) and absolute ethanol (10 mL) was stirred for 4 h to form a clear solution in darkness. TiO2 (1 g) powder was added to 100 mL absolute ethanol and stirred for 4 h in darkness to obtain a uniform suspension. The eosin solution was added dropwise into the stirring TiO2 suspension and stirred for another 10 min. The solvent was removed under vacuum when yielding red powder in darkness. Finally, the powder was dried at room temperature. The eosin/TiO2 photocatalyst was obtained and the mass ratio of eosin to TiO2 was 1:100. In the same way, the eosin/TiO2 photocatalysts whose mass ratios of eosin to TiO2 were 1:50, 1:200, 1:400 and 1:800 respectively were obtained by changing the proportion of eosin.
2.3 Photocatalytic reaction
The photocatalytic reduction was carried out in a cylindrical glass reactor. A 3 W light-emitting diode (Shenzhen Lanbaoli Photo-electricity Technology Co. Ltd., China, lights with a radiant wavelength of 525–531 nm) was used as the source of visible light, which was positioned alongside the reactor. The reactor was open to air to ensure enough oxygen in the reaction solution.0.04 g photocatalyst was suspended into 40 mL aqueous solution of phenol (10 mg/L). Prior to irradiation, the suspension was magnetically stirred by using a magnetic stirrer (JB-2 Shanghai Xinjing Co. Ltd.) in darkness for 30 min to ensure the establishment of adsorption-desorption equilibrium of phenol on photocatalyst surface. After equilibration, the concentration of phenol was measured and taken as the initial concentration (C0). Then, the light-emitting diode was opened, 5 mL solutions were sampled at appropriate time intervals. The samples were centrifuged immediately at 6000 r/min for 10 min by a high speed freeze centrifuge (KDC-160HR, Keda Innovation Co. Ltd., China) and filtered through 0.45 μm PTFE syringe filter. The filtrates were collected and analyzed. The concentration of phenol was determination by Spectrumlab 54 UV-Visible spectrophotometer (Shanghai Lengguang Lighting Technology Co. Ltd., China) at a wavelength of 510 nm through 4-aminoantipyrine spectrophotometric method [14]. It was found that the removing of 5 mL reaction mixture did not significantly affect the concentration of phenol in the remaining solution. The reaction mixture was maintained in suspension throughout the experiment. The reaction was carried out at room temperature. Duplicate were carried out for each test, and the relative standard deviation was generally less than 5%. The diffuse reflectance spectra (DRS) of eosin/TiO2 complex and TiO2 powders were examined by a UV-Visible Spectrophotometer (UV-2501PC, SHIMADZU).
3 Results and discussion
3.1 Diffuse reflectance spectra (DRS) of eosin/TiO2
Figure 1 shows the DRS of TiO2 and eosin/TiO2 powders, which are similar to the polymer/TiO2 [15–16]. As shown in Fig. 1, there is no absorption above 400 nm for pure TiO2, while eosin/TiO2 shows light absorption from 378 to about 600 nm. It is a vital prerequisite for photosensitization that the photosensitizer has been adsorbed onto the surface of semiconductor. Eosin/TiO2 seems capable of responding to visible light. The absorbency of eosin/TiO2 is maximum at about 530 nm. So, the green light-emitting diode whose radiant wavelength is 525–531 nm is used as photosource.
Fig. 1 Diffuse reflectance spectra of TiO2 and eosin/TiO2
3.2 Effect of mass ratio of eosin to TiO2 on photocatalytic activity of eosin/TiO2 under visible light irradiation
As shown in Fig. 2, the eosin/TiO2 powers with different mass ratios of eosin to TiO2 all are of visible light catalytic activities. The phenol in different eosin/TiO2 systems can be degraded in varying degrees under visible irradiation. When the mass ratio of eosin to TiO2 is 1:800, the photodegradation of phenol is only 55.9%. The photocatalytic activities of eosin/TiO2 powers are enhanced obviously as the proportion of eosin increases. In the eosin/TiO2 solutions (the mass ratios of eosin to TiO2 are 1:400 and 1: 200), the photodegradation of phenol rises to 61% and 72.7%, respectively. The photodegradation of phenol is as high as 92% using the eosin/TiO2 (m(eosin): m(TiO2)= 1:100) as photocatalyst. However, the photocatalytic activities of eosin/TiO2 powders decrease when the proportion of eosin is more than 1%. Only 77.3% phenol is degraded when the mass ratio of eosin to TiO2 is 1: 50 and the reaction solution becomes red. On one hand, the excessive proportion of eosin leads to the eosin which absorbed on the surface of TiO2 become easy to fall off. On the other hand, the TiO2 particles will gather and the partical size of TiO2 will increase when too much eosin is absorbed on the surface of TiO2, and the photocatalytic activities of eosin/TiO2 powders decrease. So, the eosin sensitized TiO2 (m(eosin): m(TiO2)= 1:100) is used as photocatalyst in subsequent experiments
Fig. 2 Effect of mass ratio of eosin to TiO2 on photocatalytic activity of eosin/TiO2
3.3 Effect of oxygen on photodegradation of phenol under visible light irradiation
As shown in Fig. 3, the degradation of phenol is 92% in air-saturated eosin/TiO2 system under visible light irradiation while in N2-saturated photocatalytic reaction is almost stopped. It indicated that O2 plays an important role in the photocatalytic degradation of phenol. It is well known that O2 is an efficient electron scavenger [17–22], which can prevent the recombination of the electron and radical cation that is produced by eosin in excited state. Cr2O72–is also able to trap the photogenerated electron [23]. As shown in Fig. 3, when O2 is replaced by Cr2O72– as electron scavenger, the photodegradation of phenol in N2-saturated system is 36.43%, which is about one-third of that in O2 as electron scavenger system. It should be pointed out that only a small proportion of the 36.43% degradative phenol is oxidized by Cr2O72– directly, because the degradation of phenol is only 8.58% under the same experimental conditions without photocatalyst. The reason for the difference between O2 and Cr2O72– is that the Cr2O72– can not generate strong oxidizing radicals which could contribute to the degradation of phenol, but O2 can. In conclusion, the role of O2 in the photocatalytic reaction is not only as electron scavenger, but more significantly, it can generate a series of active radicals.
Fig. 3 effect of O2 on photodegradation of phenol under visible light irradiation
3.4 Formation of singlet oxygen (1O2) in eosin/TiO2 system under visible light irradiation
The formation of singlet oxygen (1O2) under visible light irradiation and its role in the phenol degradation process is investigated indirectly. In these experiments, a comparison is made between the original degradation curves of phenol and those obtained after addition of sodium azide (NaN3), which is a quencher of 1O2 [24–25]. As shown in Fig. 4, the degradation ratio of phenol is 92% in the absence of NaN3 after 2 h under visible light irradiation. The ratio is reduced significantly when NaN3 is added into the system. It is reduced to 53.89% when the concentration of NaN3 is 2.5 mmol/L in the solution and decreases gradually with the concentration of NaN3 increasing. The experimental results suggest that the 1O2 which is a low-lying single excited state of molecular oxygen [25–26] might be produced in eosin/TiO2 system under visible light irradiation. The 1O2 may be produced by quenching of the excited state of the photosensitizer by oxygen [8]:
eosin + hv → 1eosin (1)
1eosin→ 3eosin (2)
3eosin + O2 → eosin + 1O2 (3)
The oxidation potential of 1O2 is about 1 V higher than its ground state, therefore, it is significantly more electrophilic [27]. As a result, it can oxidate phenol. It is also seen in Fig. 4 that when the concentration of NaN3 is more than 200 mmol/L, the degradation of phenol is almost unchanged, about 30%. Since the quantity of 1O2 which is produced in the photocatalytic process is finite in certain condition, nearly all 1O2 are quenched by NaN3 at this moment. In the presence of NaN3, the degradation of phenol drops from 92% to 30%, which indicates that the degradation of about 62% phenol is caused by singlet oxygen (1O2) and the rest of phenol is oxidated by other active species.
Fig. 4 effect of NaN3 on photodegradation of phenol under visible light irradiation
3.5 Formation of hydroxyl radical (·OH) in eosin/TiO2 system under visible light irradiation
The methylene blue can be oxidated to form colorless methyleneblue-hydroxylation (MB-OH) by hydroxyl radical (·OH) while can not by general strong oxidizer, such as K2Cr2O6, H2O2, etc. Although strong reducing agent can fade methyleneblue, the fade is reversible. In contrast, the MB-OH is stable, which could not be colored through oxidation or reduction reaction. The reason is that the sulfur atoms with intermediate valence of methyleneblue molecular have a high affinity for ·OH [28]. Therefore, ·OH is captured by methyleneblue easily and the formation of ·OH could be demonstrated by spectrophotometry indirectly. As shown in Fig. 5, the absorbancy drops from 0.185 to 0.0018 when the system is irradiated under visible light in the presence of methyleneblue of 10 μmol/L. It suggests that ·OH is formed in eosin/TiO2 system.
Fig. 5 absorbancy in eosin/TiO2 system under different conditions
In order to further confirmed the generation of ·OH, control experiments are carried out in the presence of isopropanol, which is a quencher of ·OH [29–30] in eosin/TiO2 system. As shown in Fig. 6, the degradation of phenol reduces from 92% to 89.46% in the presence of 0.01 mmol/L isopropanol. It decreases gradually with the concentration of isopropanol increasing. The experimental results suggest that the ·OH is likely to be produced in eosin/TiO2 system under visible light irradiation and it is one of free radicals for the degradation of phenol. It is also seen in Fig. 6 that when the concentration of isopropanol is more than 1 mmol/L, the degradation of phenol is almost unchanged, about 72%. Since the quantity of ·OH which is produced in the photocatalytic process is finite in certain condition, nearly all ·OH is quenched by isopropanol at this moment. In the presence of isopropanol, the degradation of phenol drops from 92% to 72%, which indicates that the degradation of about 20% phenol is caused by ·OH and the degradation of rest phenol is contributed to other active species. It is well known that oxidation potential of ·OH is 2.8 V [8] and its oxidizing is stronger than other active species. However, most of phenol was not oxidated by ·OH. It may be ascribed that ·OH that is produced on the surface of eosin/TiO2 can not be transformed to solution of phenol well.
Fig. 6 effect of isopropanol on photodegradation of phenol under visible light irradiation
3.6 Formation of hydrogen peroxide (H2O2) in eosin/TiO2 system under visible light irradiation
Because potassium iodide (KI) can be oxidated by oxidizing substance in the presence of hydrochloric acid to form iodine (I2), which is able to react with starch to form blue iodide [31]. Therefore, the determination of oxidizing substance can be carried out indirectly through the change of absorbancy in eosin/TiO2 system. As shown in Fig. 7, the absorbancy of eosin/TiO2 system which is added into KI and starch after being stirred 30 min in darkness is only 0.0075. It indicates that the oxidizing substance can not be generated in eosin/TiO2 system without visible light irradiation. When the system is irradiated by visible light, the absorbancy increases significantly amounted to 1.327. It indicates that the oxidizing substance is generated in eosin/TiO2 system under visible light irradiation. The absorbancy drops to 0.758 in the presence of catalase, which can eliminate H2O2 through hydrolysis. It suggests that the H2O2 may be generated in eosin/TiO2 system under visible light irradiation.
Fig.7 absorbancy in eosin/TiO2 system under different conditions
3.7 mechanism of photocatalytic degradation of phenol process using eosin sensitized TiO2 under visible light irradiation
As mentioned above, the 1O2, ·OH and H2O2 might be generated in eosin/TiO2 system under visible light irradiation. Because the equilibrium of photocatalytic reaction is destructed when different kinds of quenchers of the active radicals are added into system, the proportion of each active radical can not be concluded through the photocatalytic reactions under different conditions. However, according to contrast and analysis, the quantity of phenol that is degraded by 1O2 is the most, follows by other active radicals. Therefore, the 1O2 is the predominant active radical.
The mechanism of eosin/TiO2 can be concluded as: the eosin molecule absorbed on the TiO2 surface might be excited by visible light to produce excited state of eosin (1eosin and 3eosin) [8]:
eosin + hv → 1eosin (1)
1eosin→ 3eosin (2)
The oxidation potential of the excited state of eosin is more negative than the potential of the conduction of TiO2 under neutral condition. Thus, from a thermodynamical point of view, an electron can be injected from the excited state of eosin to the conduction of TiO2, with 3eosin being converted to its cation radical (eosin·+).
3eosin + TiO2 →eosin·+ + TiO2(e–) (4)
In this reaction, TiO2 as electron carrier plays an important role in the separation of injected electron and eosin cation radical (eosin·+). The injected electron can be scavenged by molecule oxygen and generate the superoxide radical (O2·-).
TiO2(e–)+ O2→TiO2 + O2·- (5)
The O2·-can convert to the H2O2, hydroperoxy (HO2·) and ·OH [19].
O2·- + H+ →HO2· (6)
HO2· + O2·- + H+ → H2O2+ O2 (7)
HO2· + H+ + TiO2(e–) →H2O2+ TiO2 (8)
H2O2 + O2·-→ ·OH + OH- + O2 (9)
H2O2 + TiO2 (e–)→ ·OH + OH- + TiO2 (10)
In addition to the above oxidative radicals (O2·-, HO2·, ·OH), 1O2 might be also formed under the present conditions. At the same time, the photosensitizer could be regenerated.
3eosin + O2 → eosin + 1O2 (3)
The degradation of phenol is contributed to all the oxidative radicals above.
4 conclusions
1) The eosin sensitized TiO2 is of photocatalytic activity. It can absorb the visible light of wavelength from 378 nm to about 600 nm.
2) The proportion of eosin has a marked effect on the photocatalytic activity of eosin/TiO2. The eosin/TiO2 (m(eosin):m(TiO2)=1:100) is the best for the photodegradation of phenol.
3) The singlet oxygen, ·OH and H2O2 might be generated in eosin/TiO2 system under visible light irradiation. The singlet oxygen is the predominant active radical for the degradation of phenol.
References
[1] PAPADAM T, XEKOUKOULOTAKIS N P, POULIOS I, Mantzavinos D. Photocatalytic transformation of acid orange 20 and Cr (VI) in aqueous TiO2 suspensions [J]. Journal of Photochemistry and Photobiology A-Chemistry, 2007, 186(2/3): 308–315.
[2] GAYA U I, ABDULLAH A H. Heterogeneous photocatalytic degradation of organic contaminants over titanium dioxide: A review of fundamentals, progress and problems[J]. Journal of Photochemistry and Photobiology C-Photochemistry Reviews, 2008, 9(1): 1–12.
[3] KIM G, CHOI W. Charge-transfer surface complex of EDTA-TiO2 and its effect on photocatalysis under visible light [J]. Applied Catalysis B: Environmental, 2010, 100(1/2): 77–83.
[4] Wang Nan, Zhu Li-hua, Huang Ying-ping, She Yuan-bin, Yu Yan-min, Tang He-qing. Drastically enhanced visible-light photocatalytic degradation of colorless aromatic pollutants over TiO2 via a charge-transfer-complex path: A correlation between chemical structure and degradation rate of the pollutants [J]. Journal of Catalysis, 2009, 266(2): 199–206.
[5] YANG C F, HUANG H H, CHEN C Y, HUANG P C, DIAO C C. The development of the physical and electrical characteristics of multi-layer TiO(2)-W-TiO(2) thin films[J]. Applied Physics A-Materials Science & Processing, 2009, 94(1): 117–122.
[6] HONDA K, YOSHIKAWA T. Characterization of TiO2 ultrafine particle prepared by low-temperature hydrolysis of TiCl4 in high-concentration hydrochloric acid aqueous solution [J]. Bunseki Kagaku, 2007, 56(1):51–54.
[7] SHABAN Y A, KHAN S U M. Visible light active carbon modified n-TiO2 for efficient hydrogen production by photoelectrochemical splitting of water [J]. International Journal of Hydrogen energy, 2008, 33(4): 1118–1126.
[8] DENG Sheng-nan, WU Feng. Environment Photochemical[M]. Beijing: Chemical Industry Press, 2003: 308–353. (in Chinese)
[9] SHANKAR K, BASHAM J I, ALLAM N K, VARGHESE O K, MOR G K, FENG X J, PAULOSE M, SEABOLD J A, CHOI K S, GRIMES C A. Recent advances in the use of TiO2 nanotube and nanowire arrays for oxidative photoelectrochemistry [J]. Journal of Physical Chemistry C, 2009, 113(16): 6327–6359.
[10] HAMAL D B, KLABUNDE K J. Synthesis, characterization, and visible light activity of new nanoparticle photocatalysts based on silver, carbon, and sulfur-doped TiO2[J]. Journal of Colloid and Interface Science, 2007, 311(2): 514–522.
[11] Chen Xiao-bo, Mao S S. Titanium dioxide nanomaterials: synthesis, properties, modifications, and applications [J]. Chemical Reviews, 2007, 107(7): 2891–2959.
[12] HUFSCHMIDT D, LIU L, SELZER V, BAHNEMANN D. Photocatalytic water treatment: fundamental knowledge required for its practical application [J]. Water Science and Technology, 2004, 49(4): 135–140.
[13] Zhang Dong-dong, Qiu Rong-liang, Song Lin, ERIC B, Mo Yue-qi, Huang Xiong-fei. Role of oxygen active species in the photocatalytic degradation of phenol using polymer sensitized TiO2 under visible light irradiation [J]. Journal of Hazardous Materials, 2009, 163(2/3): 843–847.
[14] Environmental Protection Department of China. Environment protection standard of the People’s Republic of China( HJ 503–2009) [S]. 2009.
[15] Song Lin, Qiu Rong-liang, Mo Yue-qi, Zhang Dong-dong, Wei Hui, Xiong Ya. Photodegradation of phenol in a polymer-modified TiO2 semiconductor particulate system under the irradiation of visible light [J]. Catalysis Communications, 2007, 8(3): 429–433.
[16] Song Lin, Zhang Xin, Zeng Xiao-long. Application of poly(fluorene-co- bithiophene)s as a novel sensitizer for TiO2 in the photodegradation of phenol under irradiation of GaN LED clusters [J]. Reaction Kinetics, Mechanisms and Catalysis, 2010, 102(2): 295–302.
[17] TAKEUCHI M, MARTRA G, COLUCCIA S, ANPO M.Verification of the photoadsorption of H2O molecules on TiO2 semiconductor surfaces by vibrational absorption spectroscopy [J]. Journal of Physical Chemistry C, 2007, 111(27): 9811–9817.
[18] NAKAMURA R, NAKATO Y. Primary intermediates of oxygen photoevolution reaction on TiO2(Rutile) particles, revealed by in situ FTIR absorption and photoluminescence measurements [J]. Journal of the American Chemical Society, 2004, 126(4): 1290–1298.
[19] DI VALENTIN C, FINAZZI E, PACCHIONI G, SELLONI A, LIVRAGHI S, PAGANINI M C, GIAMELLO E. N-doped TiO2: Theory and experiment[J]. Chemical Physics, 2007, 339(1/2/3): 44–56.
[20] CHO Y M, CHOI W Y, LEE C H, HYEON T, LEE H I. Visible light-induced degradation of carbon tetrachloride on dye-sensitized TiO2[J]. Environmental Science and Technology, 2001, 35(5): 966–970.
[21] SHARMA V K, GRAHAM N J D, LI X Z, YUAN B L. Ferrate(VI) enhanced photocatalytic oxidation of pollutants in aqueous TiO2 suspensions [J]. Environmental Science and Pollution Research, 2010, 17(2): 453–461.
[22] LIU Guang-ming, ZHAO Jin-cai, HIDAKA H. ESR spin-trapping detection of radical intermediates in the TiO2-assisted photo-oxidation of sulforhodamine B under visible irradiation [J]. Journal of Photochemistry and Photobiology A: Chemistry, 2000, 133(1/2): 83–88.
[23] FU Hong-xiang, LU Gong-xuan, LI Shu-ben. Photocatalytic reduction of Cr6+ ion in the presence of organics [J]. Acta physico-chimica sinci, 1997, 13(2): 106–112. (in Chinese)
[24] HAN S K, HWANG T, YOON Y, KANG J W. Evidence of singlet oxygen and hydroxyl radical formation in aqueous goethite suspension using spin-trapping electron paramagnetic resonance (EPR)[J]. Chemosphere, 2011, 84(8): 1095–1101.
[25] SCHWEITZER C, SCHMIDT R. Physical mechanisms of generation and deactivation of singlet oxygen [J]. Chemical Reviews, 2003, 103(5): 1685–1758.
[26] DEROSA M C, CRUTCHLEY R J. Photosensitized singlet oxygen and its applications [J]. Coordination Chemistry Reviews, 2002, 233–234(1): 351–371.
[27] STYLIDI M, KONDARIDES D I, VERYKIOS X E. Visible light-induced photocatalytic degradation of acid orange 7 in aqueous TiO2 suspensions [J]. Applied Catalysis B: Environmental, 2004, 47(3): 189–201.
[28] LIAO Li-fu, HE Yu-yuan, YUAN Ya-li. Determination of hydroxyl radicalin fenton reaction by visible spcetrophotometry [J]. Journal of Hengyang Medieal College, 1996(2): 130–132. (in Chinese)
[29] KIM S, CHOI W. Dual photocatalytic pathways of trichloroacetate degradation on TiO2: effects of nanosized platinum deposits on kinetics and mechanism [J]. The Journal of Physical Chemistry B, 2002, 106(51): 13311–13317.
[30] CHIA L, TANG X, WEAVERS L K. Kinetics and mechanism of photoactivated periodate reaction with 4-chlorophenol in acidic solution [J]. Environmental Science and Technology, 2004, 38(24): 6875–6880. (in Chinese)
[31] ZHANG Yao-ting, Bai Shi-ji, Zhang Wei. An improved method for determination of trace hydrogen peroxide in water [J]. Journal of Environment and health, 2006, 23(3): 258–261.
(Edited by HE Yun-bin)
Foundation item: Project(8451063201001261) supported by the Guangdong Natural Science Fund Committee, China; project(LYM08022) supported by the Foundation for Distinguished Young Talents in Higher Education of Guangdong, China; project (2007A032400001, 2008A030202010) supported by the Scientific and Technological Planning of Guangdong Province, China; project(216113132) supported by the Scientific Research Cultivation and Innovation Fund, Jinan University, China
Received date: 2011–12–15; Accepted date: 2012–05–28
Corresponding author: LI Ming-yu, PhD, Professor; Tel.: +86–13302283608; E-mail: tlimy@jnu.edu.cn
Abstract: The role of oxygen and the generation of active radicals in the photocatalitic degradation of phenol were investigated using the eosin sensitized TiO2 as photocatalyst under visible light irradiation. Diffuse reflectance spectra show that the absorbancy range of eosin/TiO2 is expanded from 378 nm (TiO2) to about 600 nm. The photocatalitic degradation of phenol is almost stopped when the eosin/TiO2 system is saturated with N2, which indicates the significance of O2. The addition of NaN3 (a quencher of single oxygen) causes about a 62% decrease in the phenol degradation. The phenol degradation ratio is dropped from 92% to 75% when the isopropanol (a quencher of hydroxyl radical) is present in the system. The experimental results show that there are singlet oxygen and hydroxyl radical generated in the eosin/TiO2 system under visible light irradiation. The changes of absorbancy indicate that the hydrogen peroxide might be produced. Through the analysis and comparison, it is found that the singlet oxygen is the predominant active radical for the degradation of phenol.
- Formation of active radicals and mechanism of photocatalytic degradation of phenol process using eosin sensitized TiO2 under visible light irradiation
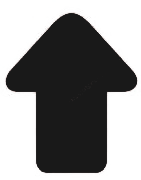