- Abstract:
- 1 Introduction▲
- 2 Experimental▲
- 3 Results and discussion▲
- 4 Conclusions▲
- References
- Figure
- Fig.1 Specimen geometry used in tests (unit: mm)
- Fig.2 Stress–strain tensile curves of EPS at 10 mm/min with different densities
- Fig.3 Creep strain vs time for different stresses: (a) ρ*=12 kg/m3; (b) ρ*= 20 kg/m3; (c) ρ*=25 kg/m3
- Fig.4 Creep strain vs time for different densities at fixed stress σ=50 kPa
- Fig.5 Creep compliance vs time for different stresses (ρ*=12 kg/m3)
- Fig.6 Standard solid model
- Fig.7 Fitting creep curves of EPS with different densities at stress level of 50 kPa
J. Cent. South Univ. Technol. (2008) 15(s1): 202-205
DOI: 10.1007/s11771-008-346-7
Uniaxial tension and tensile creep behaviors of EPS
KANG Ying-an(康颖安)1, 2, LI Xian-fang(李显方)2, TAN Jia-cai(谭加才)1
(1. Department of Mechanics Engineering, Hunan Institute of Engineering,
Xiangtan 411101, China;
2. Institute of Mechanics and Sensor Technology, Central South University, Changsha 410083, China)
Abstract:
The mechanical behavior of EPS (Expanded polystyrene) with three densities at room temperature and under tension loading was studied. The results show that EPS material is characterized by brittle behavior in the tension tests, and tensile properties of EPS increase with the increase of density. Volume fraction has no a significant effect on the modulus of these foams. The tensile creep strain increases with stress for EPS with same density, indicating that the creep behavior is of the stress dependency. And the creep behavior of EPS exhibits density dependency, which the creep strain decreases with densities for a fixed stress value. Moreover the creep behavior under the constant tension load is well in coincidence with the three-parameter solid model.
Key words:
EPS; mechanical behavior; tensile creep; density;
1 Introduction
EPS (Expanded Polystyrene) is a lightweight, rigid, plastic foam insulation material produced from solid beads of polystyrene. Expansion is achieved by virtue of small amounts of pentane gas dissolved into the polystyrene base material during production. The gas expands under the action of heat, applied as steam, to form perfectly closed cells of EPS. These cells occupy approximately 40 times the volume of the original polystyrene bead. The EPS beads are then moulded into appropriate forms suited to their application. EPS has many useful properties. It can be used as an insulator against heat and the cold. It is good at absorbing shocks and protecting delicate items. New applications using EPS have exploded in the recent years. Due to its advantages of super light, self-supporting and simple construction, EPS is an innovative building material that lends to the design and structural integrity of many building projects, and it is used to solve some problems such as excessive settlement of filling embankment in the road engineering in foreign countries[1-4]. In these applications, the foam is subjected to compressive and tensile stresses. Therefore, the mechanics behavior of EPS is of great interest[5-6]. However, lots of works on the metallic foams have been done, related study on EPS under tension and tensile creep has been relativelylimited[7-9]. Creep is the term used to describe the tendency of a solid material to slowly move or deform permanently under the influence of stresses. The rate of creep deformation is a function of the material properties, exposure time, exposure temperature and the applied load (stress). Depending on the magnitude of the applied stress and its duration, the deformation may become so large that a component can no longer perform its function. In this paper, the uniaxial tension and tensile creep tests of EPS were carried out.
2 Experimental
Three densities of EPS were investigated in this study, as the density is known to have a significant effect on mechanical response. The specimens used were cut from EPS blocks which supplied by Beipao Light Steel Building Material Company Ltd., Beijing. The average densities of foam blocks were 12, 20 and 25 kg/m3, respectively. Uniaxial tension and tensile creep tests were conducted on flat specimens with 130 mm in length, 22 mm in width, and 6 mm in thickness (see Fig.1).
Fig.1 Specimen geometry used in tests (unit: mm)
All tests were carried out on a displacement- controlled universal mechanic testing machine at room temperature of 21 ℃ and relative air humidity of 50%. By means of an extensiometer, the displacement for the specimens was measured.
3 Results and discussion
3.1 Tension tests
Stress–strain tensile curves of EPS with deferent densities at a cross-head displacement rate of 10 mm/min are shown in Fig.2. Tensile tests on EPS show that the material is characterized by brittle behavior: little or no plastic deformation before fracture; And in the tests failure occurs perpendicularly to load axis, with no detectable shrinkage, the strain to failure is less than 5%; tensile strength depends on foam density. According to the experiment data, Table 1 shows tensile elastic modulus and strength of EPS with different densities. It is seen that density is the most important structural characteristic of a cellular solid, which affects elastic modulus and tensile strength for EPS. As density increases, the tensile properties of EPS increase.
Fig.2 Stress–strain tensile curves of EPS at 10 mm/min with different densities
Table 1 Tensile property of EPS with different densities
The mechanical properties of foams under stress can be modeled by considering the mechanisms by which the cells deform and fail. The elastic modulus of a foam can be described by the following equation, derived using dimensional analysis[7].
(1)
where ρ* and E* are the density and elastic modulus of the foam, ρs and Es are those of the solid cell wall material, and φ is the volume fraction of solid contained in the cell edges. We know that ρs=1 053 kg/m3 and Es = 7 660 MPa. The volume fraction of solid contained in the cell edges is shown in Table 2. The results suggest that φ is nearly the same, and the material distribution probably has not a significant effect on the modulus of these foams. The poor mechanical properties of EPS are more likely attributable to the morphological defects in the cell faces[8-9].
Table 2 Volume fraction of solid contained in cell edges
3.2 Tensile creep tests
Creep curves of EPS with densities: 12 kg/m3, 20 kg/m3 and 25 kg/m3 at different stress levels are shown in Fig.3. It can be seen that the creep curves show the typical shape that may be divided into three stages, that is namely instantaneous elasticity stage, transient creep stage and steady state creep stage. In the initial stage, the strain rate is relatively high, but slows with increasing strain. The strain rate eventually reaches a minimum and becomes near constant. Unlike brittle fracture, creep deformation does not occur suddenly upon the application of stress. Instead, strain accumulates as a result of long-term stress. Creep deformation is time-dependent deformation. And the creep strain increases with stress for EPS with same density, which higher strain gets at higher stress level, indicating that the creep behavior is of the stress dependency.
Fig.4 shows the creep curves of EPS with different densities at the stress level of 50 kPa. It can be seen that the creep behavior of EPS exhibits density dependency, which the creep strain decreases with densities for a fixed stress value.
The corresponding apparent creep compliance for EPS with the density of 12 kg/m3 is shown in Fig.5. It can be seen that the creep compliance curves are deviated from each other after certain times, indicating that the creep behavior is nonlinear for stresses beyond 31 kPa.
Testing of EPS under suddenly-applied constant
Fig.3 Creep strain vs time for different stresses: (a) ρ*=12 kg/m3; (b) ρ*= 20 kg/m3; (c) ρ*=25 kg/m3
Fig.4 Creep strain vs time for different densities at fixed stress σ=50 kPa
Fig.5 Creep compliance vs time for different stresses (ρ*=12 kg/m3)
stress can be used to extract a time-dependent creep function, J(t). According to viscoelasticity theory, the creep strain observed can be expressed as follows[10]:
(2)
where J(t) is the apparent creep compliance function of the damaged material under stress, σ0 is constant tensile stress at any moment of time t, and t is the time of taking the reading.
The standard solid model can be represented by the Kelvin model and a Hookean spring connected in series, as shown in Fig.6. In Fig.6, E1 and E2 are the elastic moduli of the material, and η is the viscosity of the material. For this model, the creep compliance is calculated:
(3)
where q0, q1 and p1 are constants which related to E1, E2 and η. Fitting Eqns.(2) and (3) to the experimental data of Fig.4 yields three values in Table 3. And the model fitting creep curves of EPS with different densities at the stress level of 50 kPa are plotted in Fig.7. It is shown that the curves are well in coincidence with the three- parameter solid model; while in steady-state creep the prediction doesn’t agree well with the experimental value because of some nonlinear mechanisms.
Fig.6 Standard solid model
Table 3 Values of three parameters
Fig.7 Fitting creep curves of EPS with different densities at stress level of 50 kPa
4 Conclusions
EPS material is characterized by brittle behavior in the tension tests, and tensile properties of EPS increase with the increase of density. Volume fraction has no significant effect on the modulus of these foams.
The creep strain increases with stress for EPS with same density, indicating that the creep behavior is of the stress-dependent. And the creep behavior of EPS exhibits density dependency, which the creep strain decreases with densities for a fixed stress value. Moreover the creep behavior under the constant tension load is well in coincidence with the three-parameter solid model.
References
[1] DUSKOV M. Materials research on EPS20 and EPS15 under representative conditions in pavement structures [J]. Geotextiles and Geomembrances, 1997, 15: 147-181.
[2] THOMPSETT D J. Design and construction of expanded polystyrene embankments [J]. Construction and Building Materials, 1995, 9(6): 403-411.
[3] HORVATH J S. Expanded polystyrene (EPS) geofoam: An intro- duction to material behavior [J]. Geotextiles and Geomembrances, 1994, 13: 263-280.
[4] BEINBRECH G, HILLMANN R. EPS in road construction—current situation in Germany [J]. Geotextiles and Geomembrances, 1997, 15: 39-57.
[5] GNIP I J, KERSULIS V J, VAITKUS S J. Predicting the deformability of expanded polystyrene in long-term compression [J]. Mech Compos Mater, 2005, 41(5): 407-414.
[6] GNIP I Y, VAITKUS S, KERSULIS V, VEJELIS S. Long-term prediction of compressive creep development in expanded polystyrene [J]. Polymer Testing, 2008, 27: 378-391.
[7] GIBSON L J, ASHBY M F. Cellular solids: Structures and properties [M]. Cambridge: Cambridge University Press, 1997.
[8] KANG Y A, ZHANG J Y, TAN J C. Compressive behavior of aluminum foams at low and high strain rates [J]. Journal of Central South University of Technology, 2007, 14(s1): 301-305.
[9] SIMONE A E, GIBSON L J. The effects of cell face curvature and corrugations on the stiffness and strength of metallic foams [J]. Acta Materialia, 1998, 46(11): 3929-3935.
[10] ZHANG C Y. Viscoelastic fracture mechanics [M]. Beijing: Science Press, 2006.
Foundation item: Project(06C243) supported by Scientific Research Fund of Hunan Provincial Education Department, China
Received date: 2008-06-25; Accepted date: 2008-08-05
Corresponding author: KANG Ying-an, Doctor candidate, Associate professor; Tel: +86-732-8680106; E-mail:kangya2000@sina.com
- Uniaxial tension and tensile creep behaviors of EPS
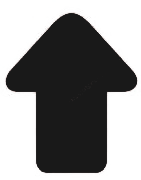