网络首发时间: 2017-04-06 17:37
稀有金属2017年第5期
锂离子电池富锂锰基正极材料的研究进展
刘祥欢 庄卫东 彭敏 王振尧 卢世刚
北京有色金属研究总院国联汽车动力电池研究院有限责任公司
摘 要:
富锂锰基正极材料x Li2Mn O3- (1-x) Li MO2 (M=Ni, Co, Mn, 0<x<1) 具有放电比容量高 (>250 m Ah·g-1) 、成本低、对环境友好等特点, 是有潜力的下一代锂离子电池用正极材料。但是该材料的首次不可逆容量高、循环和倍率性能较差, 尤其是充放电循环过程中放电中压不断降低, 阻碍了其实际应用。研究者通过采用表面包覆、表面处理、元素掺杂以及制备特殊的形貌等方法, 极大地提升了富锂锰基正极材料的电化学性能。然而, 对该材料是固溶体还是两相纳米复合的结构存在争议。另外, 对材料中的O元素和Mn元素是否可逆地参与电化学反应尚无定论。本文主要从材料结构、脱嵌锂机制和材料改性 (表面包覆、表面处理、掺杂以及特殊形貌的材料制备) 等几个方面综述了锂离子电池富锂锰基正极材料的研究进展, 并提出了下一步的研究方向。
关键词:
富锂锰基正极材料;结构;脱嵌锂机制;改性研究;锂离子电池;
中图分类号: TM912
作者简介:刘祥欢 (1990-) , 男, 云南人, 博士研究生, 研究方向:锂离子电池正极材料;;庄卫东, 教授;电话:010-82241193-8169;E-mail:wdzhuang@126.com;
收稿日期:2017-02-09
基金:国家自然科学基金项目 (51302017);科技部国家重点研发计划项目 (2016YFB0100401) 资助;
Research Progress in Li-Rich Manganese-Based Cathode Material for Li-Ion Battery
Liu Xianghuan Zhuang Weidong Peng Min Wang Zhenyao Lu Shigang
China Automotive Battery Research Institute Co.Ltd., General Research Institute for Nonferrous Metals
Abstract:
Li-rich manganese-based layered oxide ( LLO) _xLi2MnO3- ( 1-x) Li MO2 ( M = Ni, Co, Mn, 0 < x < 1) has the potential to be the cathode material for the next generation Li-ion battery, due to its high discharge specific capacity ( > 250 m Ah·g-1) , low cost and environment-friendly characteristics. However, it suffers from some problems, such as low initial coulombic efficiency, poor cycle performance, unsatisfactory rate performance and voltage fade during cycling, which hinder its practical application. Until now, the electrochemical performance of LLO have been greatly improved by means of coating, surface treatment, doping and preparation of special structures. The structure of LLO is either a solid solution or a two-phase nanocomposite, which is still controversial. Moreover, it is unclear whether the oxygen and manganese in LLO can be reversibly involved in the electrochemical reaction during its charge-discharge cycling. In this paper, the research progress in the structure, charge-discharge mechanism and modification of Li-rich manganese-based cathode material was reviewed, and the further research direction was proposed.
Keyword:
Li-rich manganese-based cathode material; structure; charge-discharge mechanism; modification; Li-ion battery;
Received: 2017-02-09
富锂锰基正极材料因其具有高放电比容量、成本低、对环境友好等特点, 近十几年来, 受到研究者们的广泛关注。相对于常用的锂离子电池正极材料Li Co O2, Li Fe PO4, Li Mn2O4, Li (Ni1/3Co1/3Mn1/3) O2以及Li (Ni0.5Co0.2Mn0.3) O2等, 富锂锰基正极材料具有更高的放电比容量 (>250 m Ah·g-1, 2.0~4.8 V, 0.1C) 。富锂锰基正极材料主要有3种不同的表达形式, 可以写成x Li2Mn O3- (1-x) LiMO2 (M=Ni, Co, Mn, 0<x<1) , 还可以写成Li1+ (x/ (2+x) ) M1- (x/ (2+x) ) O2 (M=Ni, Co, Mn, 0<x<1) , 又由于Li2Mn O3可以写成Li[Li1/3Mn2/3]O2, 所以也可以写成y Li[Li1/3Mn2/3]O2- (1-y) Li MO2 (M=Ni, Co, Mn, 0<y<1) 。通过一些简单的计算, 这3个不同的表达式之间可以相互转换, 比如0.5Li2Mn O3-0.5Li Ni0.5Mn0.5O2可以转换为Li1.2Ni0.2Mn0.6O2, 也可以转换为0.6Li[Li1/3Mn2/3]O2-0.4Li Ni0.5Mn0.5O2。
富锂锰基正极材料的研究可以追溯到20世纪90年代。早在1997年, Numata等[1]首次提出将Li2Mn O3与Li Co O2结合, 成功地制备出了富锂锰基正极材料Li (Lix/3Mn2x/3Co1-x) O2 (0<x<1) , 研究发现Li2Mn O3引入有利于提高Li Co O2在4.1~4.3 V下的结构稳定性。2001年, Lu等[2]成功合成出富锂锰基正极材料, 在2.0~4.8 V电压区间充放电, 材料的首次放电比容量超过250m Ah·g-1。十几年来, 国内外许多课题组对富锂锰基正极材料进行了大量的研究。
近年来, 为了满足纯电动汽车的长续航里程的要求, 到2020年, 我国生产的锂离子电池单体的比能量需达到350 Wh·kg-1。显然, 传统的正极材料明显达不到要求, 采用商业化Li Fe PO4为正极的锂离子电池单体的能量密度只有140 Wh·kg-1左右, 远远达不到要求。富锂锰基正极材料具有较高的放电比容量, 几乎是Li Fe PO4正极材料的两倍, 也远远高于三元正极材料, 如果与硅碳复合材料负极匹配, 电池单体的能量密度可以达到350 Wh·kg-1。因此, 富锂锰基正极材料极有可能成为下一代锂离子电池正极材料的主流材料。
富锂锰基正极材料表现出良好的应用前景。但是该材料的首次不可逆容量高、循环和倍率性能较差, 尤其是充放电循环过程中放电中压不断降低, 阻碍了其实际应用。对材料结构的认识存在争议, 是固溶体结构还是两相纳米复合结构尚无定论。材料的脱嵌锂机制也存在争议, 主要的争议是材料中的O元素和Mn元素是否可逆地参与电化学反应。为了大规模的工业化生产以及实际应用, 研究者们对材料的结构、脱嵌锂机制以及材料改性等方面做了大量的研究。
1 富锂锰基正极材料的结构研究
富锂锰基正极材料的高放电比容量与其独特的结构密切相关, 然而目前对其结构的认识依然存在争议。主要包括两种观点:一种观点认为富锂锰基正极材料是层状单斜相的Li2Mn O3 (空间群为C/m) 和层状六方相的Li MO2 (空间群为R3m) 的固溶体;而另一种观点认为富锂锰基正极材料是由层状单斜相的Li2Mn O3和层状六方相的Li MO2组成的纳米复合材料。
从组成上看, 富锂锰基正极材料可以认为是由Li2Mn O3与Li MO2 (M=Ni, Co, Mn) 两种组分构成。Li2Mn O3 (也可以写成Li[Li1/3Mn2/3]O2的形式) 与Li MO2的结构具有相似之处, 其中氧都是立方紧密堆积, 锂离子和过渡金属离子占据氧八面体的间隙[3,4,5,6], 如图1所示[6]。
Li2Mn O3中锂层和锂/锰混合层交替排列, LiMO2中锂层和过渡金属层交替排列, 它们的主要区别在于Li2Mn O3的锂/锰混合层和Li MO2的过渡金属层的不同。Li2Mn O3的锂/锰混合层中Li+/Mn4+呈有序排列, 一个Li+与六个Mn4+以花瓣状排列, 对应X射线衍射 (XRD) 图谱中20°~25°处微弱的衍射峰[7,8], 而Li MO2的过渡金属层中的过渡金属离子随机排列[9]。富锂锰基正极材料典型的XRD图[10], 如图2所示[10], 除了图谱中20°~25°处所对应的微弱的衍射峰外, 其余的峰都与LiMO2的XRD图中的衍射峰对应, 而与Li2Mn O3的XRD图中的衍射峰则全部对应, 很多研究者用Li2Mn O3相和Li MO2相的结构模型也都可以很好地拟合富锂锰基正极材料的XRD数据[11]。Lu和Dahn[12]和Numata等[13]从富锂锰基正极材料的XRD数据分析得到, 晶胞参数的变化与成分的比例呈线性关系, 服从Vegard定律, 认为材料为固溶体。
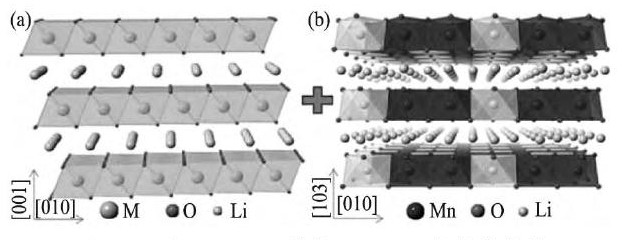
图1 六方Li MO2和单斜Li2Mn O3的晶体结构Fig.1 Crystal structure of rhombohedral Li MO2 (a) and mono-clinic Li2Mn O3 (b)
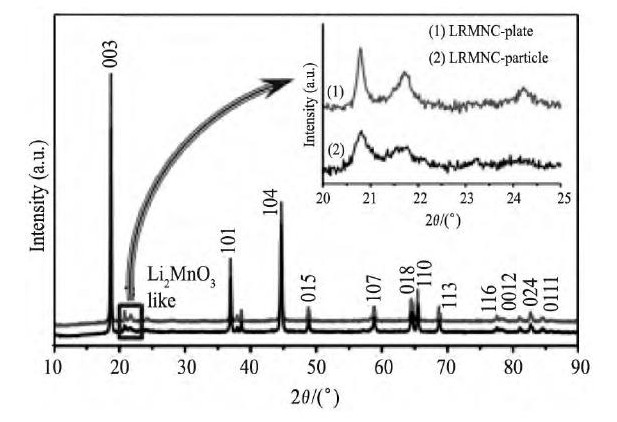
图2 Li1.2Mn0.54Ni0.13Co0.13O2的XRD图谱 (内嵌图为在20°~25°的XRD图谱) Fig.2 XRD patterns of Li1.2Mn0.54Ni0.13Co0.13O2 (insert being XRD patterns at 20°~25°)
一方面, 单斜晶系Li2Mn O3的 (001) 晶面与六方晶系Li MO2的 (003) 晶面位置刚好重合, 且晶面距离都接近0.47 nm[14,15,16], 材料的局部结构变得非常复杂;另一方面, XRD数据也只能反映材料的平均晶体结构, 因此对材料的局部结构的表征显得非常必要。高分辨的透射电子显微镜 (HR-TEM) 、明场扫描透射电镜 (annular bright-field scanning transmission electron microscopy, ABF-STEM) 以及高角度环形暗场扫描透射电镜 (high-angle annular dark-field scanning transmission electron microscopy, HAADF-STEM) 能够在原子级别直接观察材料的结构, 是对材料的局部结构观察最有效的手段。Boulineau等[17]采用HAADF-STEM观察到在Li1.2Mn0.61Ni0.18Mg0.01O2 (即0.6Li2Mn O3-0.4Li Ni0.45Mn0.525Mg0.025O2) 中形成了Li2Mn O3和Li MO2共存的微区。Baren1o等[18]采用选区电子衍射 (SAED) 和延伸X射线吸收精细结构 (EXAFS) 对Li1.2Co0.4Mn0.4O2进行深入分析, 发现材料含有六方Li MO2相和单斜Li2Mn O3相。然而, Jarvis等[19]采用HRTEM对富锂锰基正极材料的原子排列进行观察, 提供了Li[Li0.2Ni0.2Mn0.6]O2是固溶体的证据。
北京工业大学的Yu等[20]对富锂锰基正极材料的结构做了大量深入的研究, 2013年, 他们结合ABF-STEM和HAADF-STEM, 在原子级别直接观察到在Li1.2Mn0.567Ni0.166Co0.067O2中有两相的存在, 即单斜Li2Mn O3相和六方Li MO2相, 另外SAED的结果也表明材料中有两相的存在。尽管能够直接观察到两相的存在, 但仅仅研究了材料的表面区域, 所以还不能判断在材料的内部也有两相的存在。经过多年的努力, 2016年, Yu等[21]采用Ar离子减薄技术, 成功获得厚度为20~50 nm, 面积为105 nm2的薄区, 采用ABF-STEM和HAADF-STEM不仅直接观察到材料表面原子的排列信息, 还观察了材料内部原子的排列信息, 并且在Li1.2Mn0.567Ni0.166Co0.067O2颗粒的每个微区中都观察到Li MO2相和Li2Mn O3相实则是交互生长的“双晶畴”, 图3[21]为材料颗粒某个区域的HAADF-STEM。结合明场透射电镜 (BF-TEM) 和电子衍射 (ED) 测试, 作者还提出了富锂锰基正极材料中Li2Mn O3相的Li Mn2有序结构层堆叠的方向不同。
综上所述, 对富锂锰基正极材料结构的认识还存在争议, 尽管研究发现材料中存在Li MO2和Li2Mn O3两相, 但是仍然存在一些问题, 比如, 材料中Li MO2相和Li2Mn O3相是如何分布以及材料是否可以按设计的比例形成, 这些问题都尚未说明。目前富锂锰基正极材料的结构仍不是十分清楚, 其是否与合成条件、锂含量以及过渡金属组分等因素密切关联, 还需作进一步深入研究。
2 富锂锰基正极材料的脱嵌锂机制研究
富锂锰基正极材料在2.0~4.8 V的电压区间, 可以放出大于250 m Ah·g-1的比容量。但是, 传统的层状正极材料的脱嵌锂机制无法解释富锂锰基正极材料具有高容量的原因, 针对这种情况, 近十几年来, 相关的科研工作者进行了大量深入的研究, 然而, 到目前为止对富锂锰基正极材料的脱嵌锂机制依然没有达成共识。
富锂锰基材料典型的充电曲线如图4所示[22], 首次充电曲线与第二次充电曲线存在较大的差异。在材料的首次充电过程中, 充电曲线存在两个明显的区域:在小于4.5 V (vs.Li+/Li) 的充电区域, 该材料中的Li MO2组分的过渡金属元素Ni氧化提供容量, 随着Li+的脱出, Ni2+氧化成Ni4+ (含Co元素的Co3+氧化到Co4+) [23], 该过程的反应机制可以用传统层状材料的嵌脱锂机制来解释, 即Li MO2→Li++MO2+e-;在电压超过4.5V (vs.Li+/Li) 的区域, 出现一个明显的放电平台, 至于出现这个平台的原因, 研究者们大都认为是与富锂材料中另一组分Li2Mn O3相关[24,25,26,27]。
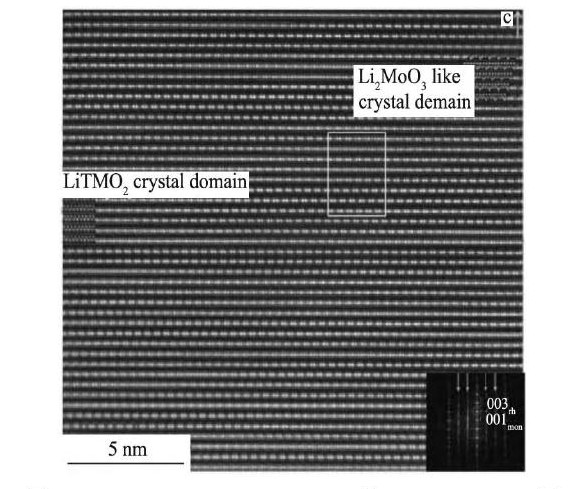
图3 Li1.2Mn0.567Ni0.166Co0.067O2的HAADF-STEM图Fig.3 HAADF-STEM image of Li1.2Mn0.567Ni0.166Co0.067O2
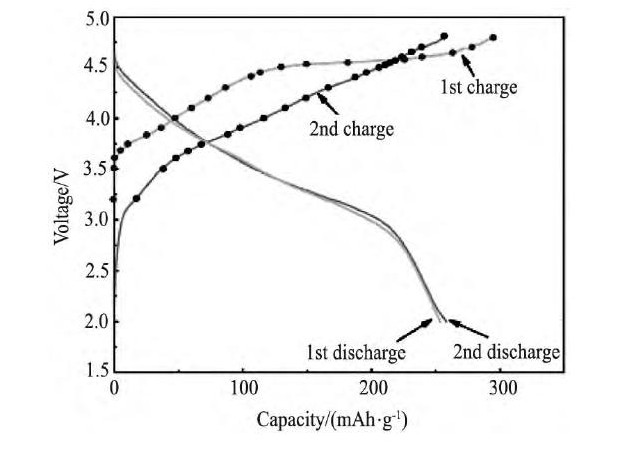
图4 0.5 Li2MnO3-0.5 LiN i0.5Mn0.5O2的首周以及第二周的充放电曲线Fig.4 First and second charge-discharge profiles of0.5 Li2MnO3-0.5 LiN i0.5Mn0.5O2
早在2003年, Robertson和Bruce[28]对Li2Mn O3材料的脱嵌锂机制进行研究, 发现在脱锂后, 材料中的Mn仍然保持+4价。他们认为, 电极材料在较高温度 (55℃) 充电时, 扣式电池中的有机电解液会在较高电压下发生氧化反应生成H+并提供电子, H+与材料中的Li+发生离子交换形成Li2-xHxMn O3。而电极材料在较低温度 (30℃) 充电时, Li+的脱出伴随着材料中晶格氧的流失以及与H+的离子交换。然而, Dogan等[29]和Croy等[30]采用核磁共振 (nuclearmagnetic resonance, NMR) 和X射线吸收光谱 (X-ray absorption spectroscopy, XAS) 对材料进行研究, 结果表明, 在首次充电过程中并没有发现H+与材料中的Li+发生离子交换。
Lu等[2]认为富锂锰基正极材料在首次充电 (>4.5 V) 时, Li+与O以“Li2O”的形式同时从富锂材料中脱出, 这就是所谓的“氧流失”机制。Armstrong等[31]与La Mantia等[32]分别采用原位电化学质谱和原位微分电化学质谱直接检测到在材料首次充电至4.5 V时有O2析出。Park等[33]通过原位的电池内压测试证实在电压高于4.5 V时有气体析出。Konishi等[34]对脱锂态的Li1.2Ni0.2-xMn0.6-xCo2xO2 (x=0, 0.033和0.067) 进行研究, 热脱附光谱-质谱 (thermal desorption spectroscopymass spectroscopy, TDS-MS) 测试结果表明, 在测试中脱锂态的富锂材料伴有氧的释放。Koyama等[35]用第一性原理计算也证明了4.5 V左右平台为O2p的氧化。Seo等[36]通过第一性原理计算也表明富锂正极材料中的氧能发生氧化, 他们认为, 这与富锂材料中存在独特的Li-O-Li结构有关。这些研究都为“氧流失”机制提供依据。富锂锰基正极材料中的氧流失后 (伴随着Li+从过渡金属层中脱出) , 导致首次充放电后, 材料的结构发生重排[37,38,39]。另外, 从富锂锰基正极材料的首次充电曲线与第二周充电曲线的差异, 也可以间接推论出材料在首次循环后结构发生重排[40]。Genevois等[41]利用HAADF-STEM以及ED对Li1.20Mn0.54Co0.13Ni0.13O2进行深入研究, 发现在脱嵌锂过程中, 材料的颗粒表面会发生过渡金属离子的迁移, 使材料的表面结构发生变化, 这说明首次充放电后材料的结构发生重排。Wang等[42]采用ABF-STEM直接从原子级别观察Li2Mn O3材料的结构, 观察到在电化学脱锂后, 锂离子从Li2Mn O3的Li Mn2层中脱出, 并且一些Mn离子从过渡金属层迁移到锂层, 另外完全化学脱锂之后, Li2Mn O3能够转变为Mn O2。
富锂锰基正极材料在氧流失后, 其结构不稳定会转变为传统的层状材料脱锂后的结构即MO2·Mn O2[2,42], “氧流失”机制虽然可以解释材料后续放电的高比容量, 但是却需要几乎材料中全部的Mn参与电化学反应。然而, 高度脱锂后的MO2·Mn O2是怎样保持结构稳定性的, 从这一角度来说, “氧流失”机制也很难解释。
由于“氧流失”机制存在一定的缺陷, 并不能很好地解释富锂锰基正极材料具有高可逆放电比容量的原因, 所以许多研究者继续对富锂锰基正极材料的脱嵌锂机制进行深入的研究。
Muhammad等[43]采用XAS对0.4Li2Mn O3-0.6Li Mn0.5Ni0.5O2进行表征, 研究发现在充放电过程中, 材料中的Mn始终以Mn4+存在, 不参与电化学反应。同时, 通过对材料充放电过程中的高分辨同步XRD数据进行结构精修, 计算出材料中氧含量的变化, 如图5所示[43], 作者认为在充放电过程中, 材料中的O能够可逆脱嵌。
Hy等[44]采用原位表面增强拉曼光谱 (SERS) 对Li1.2Ni0.2Mn0.6O2进行研究, 结果表明, 在首次充电到电压大于4.4 V, 材料中有Li2O相生成 (如表1中反应式 (3) 所示) , 部分氧从O2-氧化到O- (如表1中反应式 (4) 所示) , 在充电末端有少量O2的生成 (如表1中反应式 (5) 所示) 。
Oishi等[45]对Li1.16Ni0.15Co0.19Mn0.50O2中O的K边XAS光谱进行分析表明, 材料充放电过程中 (O2) 2-可逆的生成与消耗, 能够直接说明脱嵌锂过程中, O参与电化学反应, 提供电荷补偿。2015年, Han等[46]采用X射线光电子能谱 (XPS) 对Li1.14Ni0.136Co0.136Mn0.544O2和Li2Mn O3的表面进行测试, 分析认为, 在充放电过程中, 这两种材料中的部分O发生了可逆的氧化还原反应 (作者认为可能是O2- (O2) 2-) 。
Luo等[47]采用18O标记Li1.2[Ni0.13Co0.13Mn0.54]O2, 对材料进行原位质谱测试, 结果表明, 在首次充电过程中, 尽管没有检测到18O2生成, 但检测到C18O2生成, 这也说明了O从材料的晶格中脱出。此外, 软X射线吸收光谱 (soft X-ray absorption spectroscopy) 、X射线吸收近边结构光谱 (X-ray absorption near edge structure spectroscopy, XANES) 以及拉曼光谱 (Raman spectroscopy) 测试表明, 材料中的氧除了少量流失外, 大部分的氧会参与电荷补偿。作者认为, 氧发生电荷补偿的机制是:在脱锂时, 与Mn4+和Li+配位的O原子会形成局部的电子空穴以提供电荷补偿, 而不是形成真正的 (O2) 2-。
2016年, Shimoda等[48]采用探测深度约为50 nm的硬X射线光电子能谱 (HAX-PES) 对Li[Li0.25Ni0.20Mn0.55]O1.93进行深入研究, 结果表明充放电过程中, 材料中有O-生成, 通过解析O1S的光电子能谱, 得到材料首次充放电过程中[O-]/[O-+O2-]的比值。作者认为, 充电过程中:在2.0~4.5V的电压区间, 材料中的Ni和少量的O发生氧化, Ni从Ni2+氧化到Ni4+, 而O从O2-氧化到O-;在4.5~4.8 V的电压区间, 材料中的O从O2-氧化到O-提供电荷补偿。放电过程中:在4.8~3.5 V的电压区间, 材料中的Ni4+和O-发生还原;在3.5~2.0 V的电压区间, Mn4+还原为Mn3+和Mn2+。
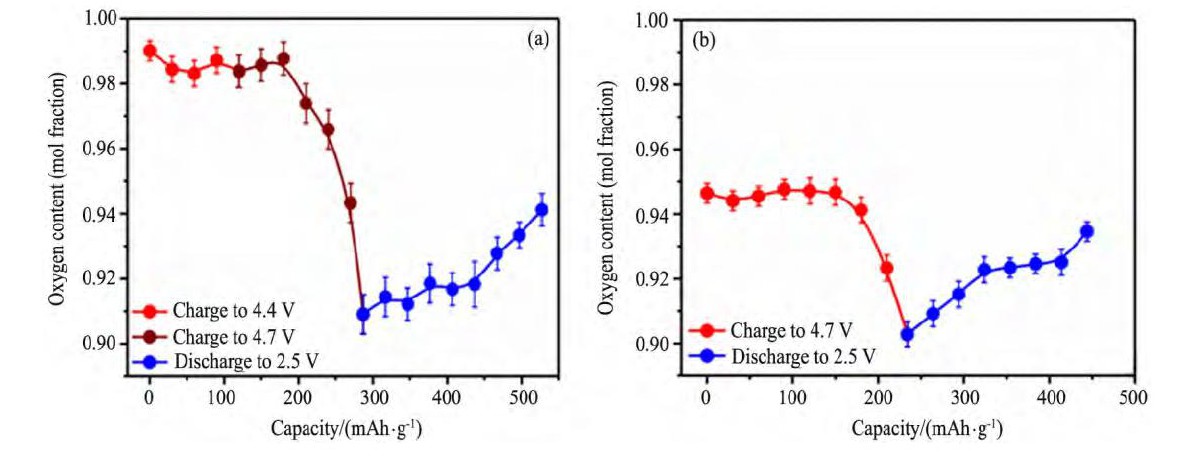
图5 首周充放电循环和第二周充放电循环0.4Li2Mn O3-0.6Li Mn0.5Ni0.5O2氧含量变化Fig.5 Variation of oxygen content in 0.4Li2Mn O3-0.6Li Mn0.5Ni0.5O2during first cycle (a) and during second cycle (b)
表1 Li1.2Ni0.2Mn0.6O2首次充电到4.8 V对应的反应方程式Table 1 Different reactions occurring during charging for Li1.2Ni0.2Mn0.6O2up to 4.8 V 下载原图
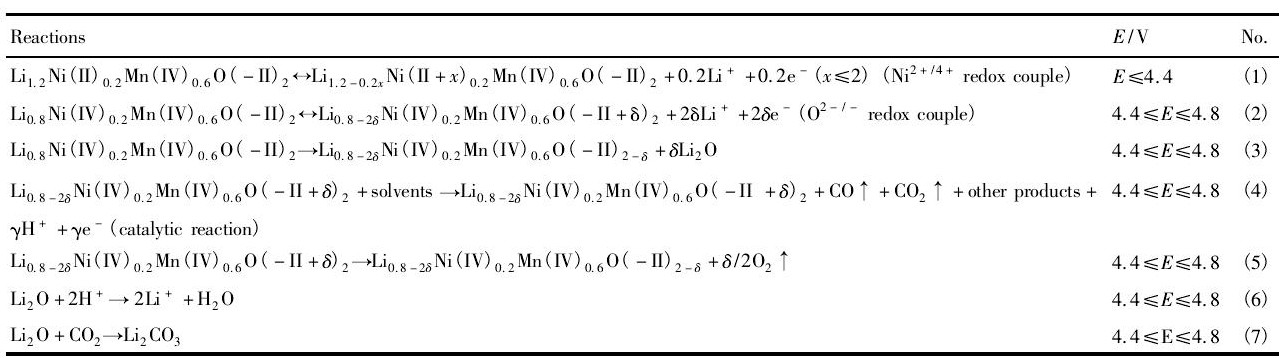
表1 Li1.2Ni0.2Mn0.6O2首次充电到4.8 V对应的反应方程式Table 1 Different reactions occurring during charging for Li1.2Ni0.2Mn0.6O2up to 4.8 V
综上所述, 随着研究的深入, 近年来研究者对富锂锰基正极材料的首次锂脱出机制的认识基本一致, 认为电压<4.5 V的区域, Ni (和Co) 参与电荷补偿;电压≥4.5 V的区域, 氧发生氧化 (O2-氧化为O-) 以及少量氧流失 (以“Li2O”的形式) ;充电过程中Mn不参与电化学反应。而对材料的首次嵌锂机制存比较大的争议, 最早的观点认为, 只有Ni (和Co) 、Mn参与电荷补偿而O不参与电荷补偿;近年来的观点认为, 除了Ni (和Co) 参与电荷补偿外, O参与电荷补偿或Mn和O都参与电荷补偿。由于O参与电荷补偿的直接证据尚不完善, 所以对于O是否参与电荷补偿还需要做更深入的研究。
另外, 首次充电后, 材料的结构变化尚不清楚, 电极材料后续充放电的脱嵌锂机制以及在高温下的脱嵌锂机制也尚不清楚, 均需作进一步研究。
3 富锂锰基正极材料的制备方法研究
现阶段文献报道的富锂锰基正极材料的制备方法主要有共沉淀法[49,50,51,52,53,54]、溶胶-凝胶法[55,56,57]以及高温固相法[58,59]。另外也有一些关于水热法[60,61,62]、喷雾干燥法[63]、燃烧法[64,65]、离子交换法[66,67]和熔盐法[68]合成材料的文献报道。
Miao等[69]采用共沉淀法合成含锂前驱体 (其中Li OH作为锂源和沉淀剂) , 然后将共沉淀后的溶液转移到容器中, 在180℃下微波处理60 min, 将处理后的物质洗涤、干燥, 并在800℃焙烧3 h后, 得到目标产物x Li2Mn O3- (1-x) Li Ni1/3Co1/3Mn1/3O2 (x=0.2, 0.4, 0.6, 0.8) ;电化学测试表明x=0.4的样品具有最佳的电化学性能, 在0.1C, 2.5~4.8 V充放电条件条件下, 材料的首次放电比容量高达325 m Ah·g-1。Yuan等[70]采用8-羟基喹啉作为沉淀剂成功合成Li[Li0.2Mn0.54Ni0.13Co0.13]O2, 电化学测试表明, 在0.2C, 2.0~4.8 V条件下, 材料的首次放电比容量高达287.2m Ah·g-1;采用8-羟基喹啉作为沉淀剂合成的材料在2.0~4.8 V, 2.0C倍率下循环100周后, 材料的放电比容量高达207.3 m Ah·g-1, 而采用Na OH作为沉淀剂合成的样品, 在相同条件下循环100周后, 材料的放电比容量仅为156.3 m Ah·g-1, 如图6所示[70]。
Wang等[68]采用熔盐法制备了Li[Li0.26Ni0.11Mn0.63]O2 (以KCl-Na Cl为熔盐) , 电化学测试表明, 在相同的充放电条件下, 相对于共沉淀法合成的样品, 熔盐法制备的材料具有更好的倍率性能和循环性能, 如图7所示[68]。
4 富锂锰基正极材料的改性研究
富锂锰基正极材料本身存在诸多问题:首次不可逆容量高;容量衰减严重;由于材料本身的锂离子以及电子电导性较差[71], 导致倍率性能不佳;在后续的循环中存在电压降。以上问题的存在, 严重影响了富锂锰基正极材料的应用, 因此国内外的许多研究者对富锂锰基正极材料的改性进行了大量的研究, 常用的改性方法有表面包覆、表面处理, 元素掺杂以及制备特殊形貌的电极材料等。
表面包覆可避免电极材料与电解液的直接接触, 抑制循环过程中HF对电极材料的侵蚀, 减少材料中过渡金属元素的溶解, 减少电极材料与电解液发生的副反应, 抑制材料在后续循环过程中SEI膜的生长, 降低电池在充放电过程中的电荷转移电阻, 可进一步提高材料的电化学性能;表面处理可以改善电极材料的表面状态或结构, 有助于锂离子的脱嵌, 从而改善材料的电化学性能;引入掺杂元素能够提高材料晶体结构的稳定性, 减少材料过渡金属溶解以及氧的损失, 改善材料的电化学性能;通过调控合成条件从而控制材料的晶面取向, 制备具有特殊形貌的富锂锰基正极材料, 有助于材料电化学性能的改善。
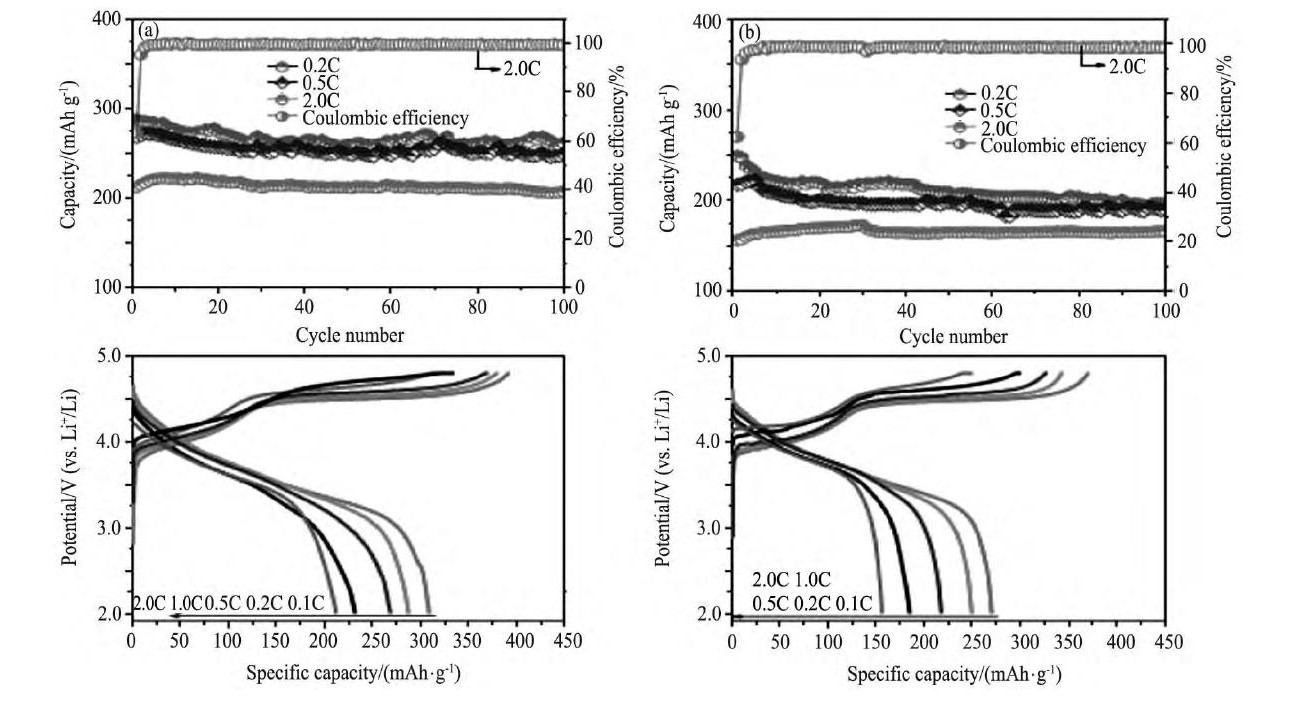
图6 不同沉淀剂合成的Li[Li0.2Mn0.54Ni0.13Co0.13]O2在0.2C, 0.5C, 2.0C倍率下的循环性能8-羟基喹啉 (沉淀剂) , Na OH以及在0.1C, 0.2C, 0.5, 1.0C, 2.0C倍率下的首次充放电曲线, 8-羟基喹啉 (沉淀剂) , Na OH Fig.6 Cycle performance of Li[Li0.2Mn0.54Ni0.13Co0.13]O2prepared with precipitants of (a) 8-hydroxyquinoline and (b) Na OH at0.2C, 0.5C, 2.0C, respectively;the first charge-discharge curves of materials at 0.1C, 0.2C, 0.5C, 1.0C and 2.0C, re-spectively, (c) 8-hydroxyquinoline and (d) Na OH
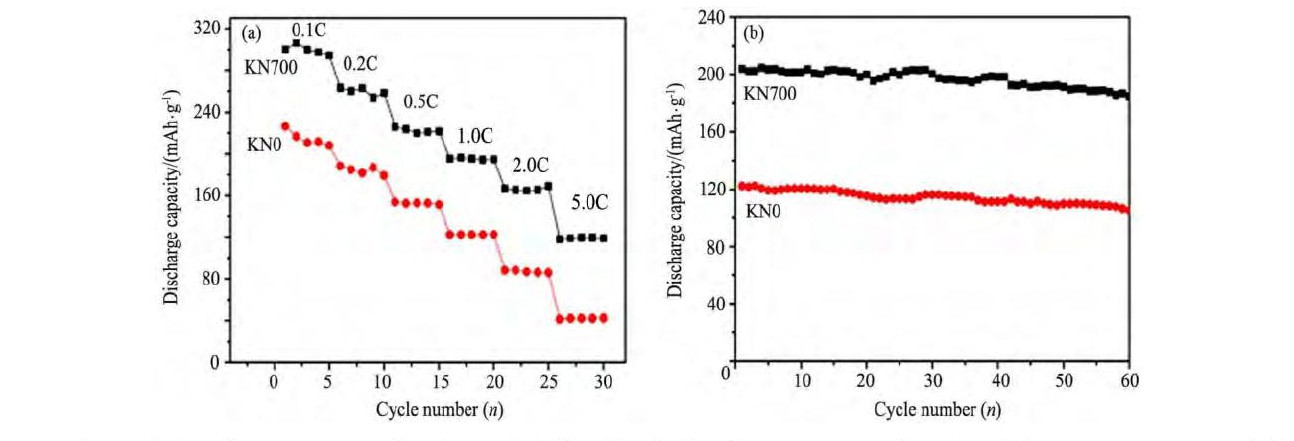
图7 熔盐法合成Li[Li0.26Ni0.11Mn0.63]O2 (KN700) 与共沉淀法合成Li[Li0.26Ni0.11Mn0.63]O2 (KN0) 在2.0~4.8 V, 1.0C条件下的倍率性能和循环性能Fig.7 Rate (a) and cyclic (b) performance of Li[Li0.26Ni0.11Mn0.63]O2at a 1.0C rate between 2.0 and 4.8 V
4.1 表面包覆改性
富锂锰基材料具有较高的容量, 但是材料在后续的循环中, 材料表面出现Ni/Mn元素分离的现象, 材料颗粒表面形成微裂纹、颗粒粉化、过渡金属溶解, 以及材料表面结构发生不可逆的相变等问题[72,73,74], 因此稳定材料颗粒表面结构显得尤为重要。
研究发现, 对富锂锰基正极材料进行表面包覆, 能够在一定程度上稳定材料的表面结构, 达到改善材料电化学性能的作用。常用的包覆材料主要有简单氧化物Ti O2[75], Al2O3[76,77], Zr O2[78], Mn O2[79,80], Mo O3[81], Ce O2[82,83]等, 磷酸盐FePO4, Co PO4[84], Ni PO4[85]等, 氟化物Ca F2[86], Al F3[87,88], Sm F3[89]等, 有机高分子聚合物[90,91]、锂离子良导体Li Al O2[92], Li2Zr O3[93], Li2Ti O3[94], Li4Ti5O12[95], Li3VO4[96], Li Ni PO4[97]等, 电子良导体单质Al[98]、碳材料[99,100]等。这些包覆层或多或少都可以避免电极材料中的活性物质与电解液的直接接触, 降低材料的溶解, 减少电极/电极液界面副反应的发生, 从而改善了材料的电化学性能。
Yu等[101]采用溶胶-凝胶法合成Li1.2Ni0.13Co0.13Mn0.54O2, 并在材料二次颗粒表面包覆了5%的Zn O, 相关测试表明Zn O包覆层能够抑制电解液的分解, 减小电极/电解液界面副反应的发生, 有效地稳定了电极/电解液界面结构。在后续的充放电循环中, 与未包覆样品相比, 包覆后的材料具有更小的电荷转移阻抗 (Rct) 及更高的锂离子扩散速度。Yuan等[82]在Li[Li0.17Ni0.2Co0.05Mn0.58]O2表面包覆1%Ce O2, 循环80周后, 包覆的样品放电容量为258.1 m Ah·g-1 (2.0~4.8 V, 0.1C) , 而未包覆的样品放电容量仅有229.8 m Ah·g-1 (2.0~4.8 V, 0.1C) ;在1.0C倍率下, 充放电循环80周后包覆样品的容量保持率为90.8%。Wu等[81]采用一定量的Mo O3对Li[Li0.2Mn0.54Ni0.13Co0.13]O2进行包覆, 电化学测试结果表明, 5%Mo O3包覆的样品具有最佳的电化学性能;由于Mo O3能够提供多余的锂位, 包覆后材料的首次不可逆容量损失减小。Wang等[102]以2%Al PO4或2%Co PO4为内包覆层, 2%~3.5%Al2O3为外包覆层对Li[Li0.2Mn0.54Ni0.13Co0.13]O2进行双层包覆, 材料的首次不可逆容量减少至26 m Ah·g-1, 首次放电比容量接近300 m Ah·g-1;2%Al PO4+3%Al2O3和2%CoPO4+3%Al2O3双层包覆的样品, 在2C倍率下的放电比容量分别为215和204 m Ah·g-1, 较只包覆Al PO4, Co PO4和Al2O3时有很大提高。
在充放电循环中, 电解液的溶质Li PF6容易与痕量的水反应生成HF, HF能够与活性物质发生反应, 导致材料溶解, 使材料的电化学性能变差, 采用氟化物包覆能够有效抑制HF对材料表面的侵蚀。Liu等[86]结合Na2S2O8预处理与Ca F2包覆对Li1.2Mn0.54Ni0.13Co0.13O2进行改性研究, 相关测试表明, Na2S2O8预处理能够有效抑制材料在长循环过程中的电压降, 从而减缓材料能量密度的衰减;Ca F2包覆层能够减轻HF对材料的腐蚀, 减少Mn元素的溶解, 从而改善了材料的循环性能;40%Na2S2O8-2%Ca F2改性后的样品, 材料的首次库伦效率高达99.2%, 循环200周后, 容量保持率高达89.2%, 如图8所示[86]。Lu等[89]采用无定型态的Sm F3对Li1.2Mn0.52Co0.08Ni0.2O2进行包覆, Sm F3包覆层的厚度约为20 nm, 在2C倍率下循环150周, 包覆样品的容量保持率为84.5%, 而未包覆样品的容量保持率只有68.9%。Zheng等[87]和Sun等[103]对Al F3包覆富锂锰基正极材料的改性机制进行深入研究, 相关研究表明, Al F3包覆层减少电解液在高电压 (>4.5 V) 下的氧化分解, 抑制了SEI膜的快速生长;Al F3包覆层还能显著提高材料表面结构的稳定性, 减少HF对电极的腐蚀以及活性材料的溶解;表面包覆层还能减缓材料颗粒内部结构从层状向尖晶石相转变, 从而减缓了材料的电压降。
由于富锂锰基正极材料本身的离子/电子电导率较低, 使得材料的倍率性能较差, 为了改善其离子/电子导电性, 国内外的研究者们通过在材料表面包覆具有良好离子导电性的离子导体以及良好电子导电性的电子导体。Zhang等[93]采用Li2Zr O3对富锂锰基正极材料进行包覆, 电化学测试表明, 1%Li2Zr O3包覆的样品具有最优的电化学性能;Zhao等[94]采用3%Li2Ti O3对Li1.13Ni0.30Mn0.57O2进行包覆改性, 研究结果表明, 在5C倍率下循环100周后, 材料的放电容量从未包覆的78 m Ah·g-1提升到105 m Ah·g-1;Guo等[99]通过静电纺丝和热处理显著改善了Li1.2Co0.13Ni0.13Mn0.54O2的倍率性能和循环性能, 这是由于在材料表面形成导电性良好的多壁碳纳米管 (MCNTs) 网络, 如图9所示[99]。
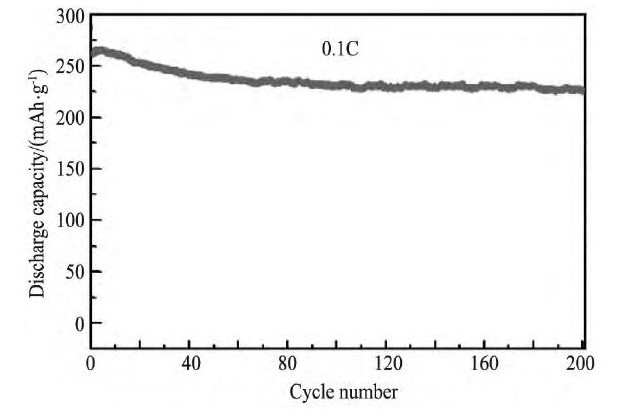
图8 0.1C倍率下, 40%Na2S2O8-2%Ca F2处理后的Li1.2Mn0.54Ni0.13Co0.13O2的循环性能Fig.8Cyclic performance at 0.1C for 40%Na2S2O8treated and 2%Ca F2-coated Li1.2Mn0.54Ni0.13Co0.13O2
最近, Qiao等[104]将一定量的四水氯化锰和2, 5-二羟基对苯二甲酸溶解于体积比为15∶1∶1的二甲基甲酰胺-乙醇-去离子水的混合溶液中, 然后将Li (Li0.17Ni0.20Co0.05Mn0.58) O2材料倒入上述溶液中, 经过一系列的处理过程, 得到具有Mn-有机物结合物 (MOF) 包覆的目标产物。作者认为, 这种Mn-有机物结合物具有高的比表面积、较大的孔、稳定的框架结构而且具有一定的电化学活性 (由于Mn的存在) , 一方面其能够抑制基体材料在首次充电过程中晶格氧的流失, 另一方面有机配位体与基体材料中的金属离子之间的相互作用, 使得基体材料的结构更加稳定。包覆改性后样品在0.1C倍率下的首次放电比容量高达323.8 m Ah·g-1, 首次库伦效率高达91.1%。图10[104]为材料的循环性能图。
综上所述, 对富锂锰基正极材料进行包覆, 可以有效改善材料的电化学性能。材料包覆改性的效果, 除了与包覆物的选择有关外, 还需要严格控制好包覆物的量。
4.2 表面处理改性
为了减小材料的首次不可逆容量, 早在2004, Johnson等[105]采用酸处理的方式对材料的表面进行改性, 他们分析认为, 酸处理后富锂锰基正极材料的Li2Mn O3相中的Li+和O会以“Li2O”的形式脱出, 减少了材料的首次不可逆容量。但是更多的研究表明, 酸处理会对材料的结构造成破坏, 使得材料的循环和倍率性能变差。Zheng等[106]采用Na2S2O8对Li[Li0.2Mn0.54Ni0.13Co0.13]O2材料进行处理, 研究表明处理后样品的首次库伦效率接近100%, 倍率性能也有改善, 作者认为, 这是因为在材料的表面形成了锂离子更容易扩散的尖晶石相。
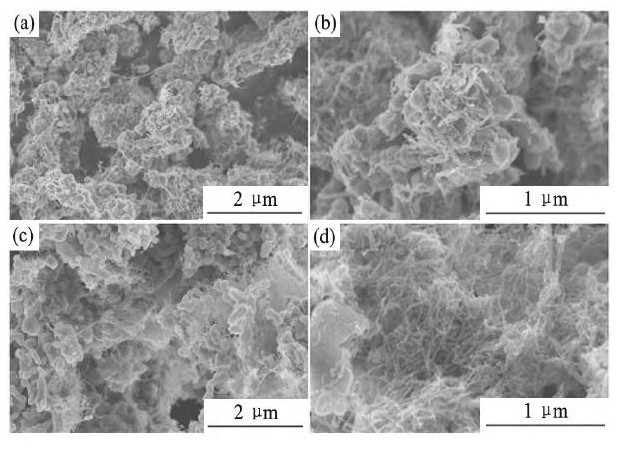
图9 15%MCNTs-Li1.2Co0.13Ni0.13Mn0.54O2和20%MCNTs-Li1.2Co0.13Ni0.13Mn0.54O2的SEM图Fig.9 SEM images of 15%MCNTs-Li1.2Co0.13Ni0.13Mn0.54O2 (a, b) and 20%MCNTs-Li1.2Co0.13Ni0.13Mn0.54O2 (c, d)
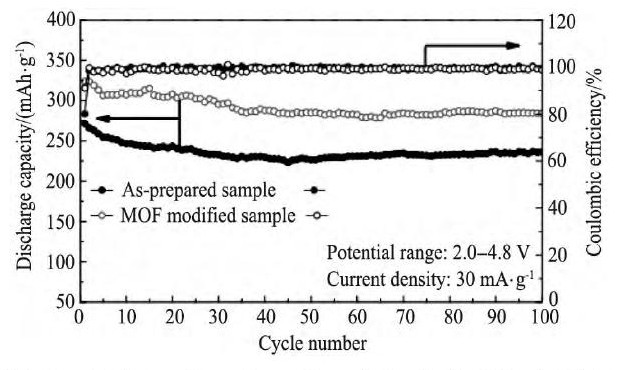
图1 0 Li (Li0.17Ni0.20Co0.05Mn0.58) O2以及MOF包覆Li (Li0.17Ni0.20Co0.05Mn0.58) O2的循环性能 (2.0~4.8V, 30 m A·g-1) Fig.10 Cycle performance of as-prepared and MOF-modified Li (Li0.17Ni0.20Co0.05Mn0.58) O2samples at 30 m A·g-1
2012年, Abouimrane等[107]将Li1.12Mn0.55Ni0.145Co0.1O2置于含有3.5%氢气的氩气中, 250℃煅烧3 h, 使材料表面少量的Mn4+还原成Mn3+, 通过测试发现表面处理样品的电导率为 (4.7±0.4) ×10-7S·cm-1, 而未处理样品的电导率为 (3.2±0.3) ×10-7S·cm-1, 从而提高材料的倍率性能, 该材料在320 m A·g-1的电流下可释放196 m Ah·g-1的容量。2013年, Song等[108]采用超声辅助的方法将Li (Li0.2Mn0.54Ni0.13Co0.13) O2与Super p分散在N-甲基吡咯烷酮 (NMP) 中, 继续搅拌12 h, 130℃干燥12 h, 将所得干燥粉末350℃保温2 h, 得到目标产物。结合XRD, XPS, HRTEM以及相应的电化学测试, 作者认为, 在350℃保温2 h, Super p的存在会使材料的表面形成类尖晶石结构, 这有助于材料电化学性能的发挥。在充电电流密度为2500 m A·g-1、放电电流密度为250 m A·g-1条件下, 质量分数为5%的Super p表面处理后样品的放电比容量高达200 m Ah·g-1, 而对比样的放电比容量仅有75 m Ah·g-1。
最近, Qiu等[109]通过设计气-固界面反应使富锂锰基正极材料的表面形成均匀的氧空位, 并且材料的内部结构保持不变。理论计算和实验表明, 材料表面形成的氧空位使得材料内部的锂离子更加容易扩散并且极大地抑制了材料表面氧的流失。表面处理后样品的首次放电比容量和首次库伦效率高达301 m Ah·g-1和93.2%, 并且在循环100周后, 材料的放电比容量仍高达301 m Ah·g-1, 容量几乎没有衰减。
4.3 元素掺杂改性
研究发现, 对材料进行元素掺杂, 可以提升材料晶体结构的稳定性, 提高材料的电子电导率或离子导电率, 降低材料的电化学阻抗, 改善材料的循环稳定性和倍率性能。国内外研究者们对富锂锰基正极材料的掺杂改性进行了大量的研究, 主要包括以下4个途径: (1) Li位掺杂Na[110], K[111]等; (2) 过渡金属位掺杂Fe[112,113], Zr, Ti, Zn[114], Sn, Mo[115], Al[116], Cr[117]等; (3) O位掺杂F[118,119]; (4) 将B等元素掺杂在过渡金属层的四面体位形成聚阴离子 (BO4) [120]等。
Lim等[110]采用Na+对Li1.167Ni0.18Mn0.548Co0.105O2中的Li+进行部分取代, 相关测试表明Na+的掺杂, 能够有效改善材料的倍率性能。Li等[111]采用K+对Li1.20Mn0.54Co0.13Ni0.13O2中的Li+进行部分取代, 研究表明, K+掺杂能够抑制材料循环过程中由层状向尖晶石相转变, 其作用机制是掺杂的K+固定在Li位, 阻碍部分Mn迁移的路径, 抑制尖晶石相的形成, 如图11[111] (b) 所示。K+掺杂改性后的样品, 在放电电流密度为20 m A·g-1下循环110周, 材料的放电比容量高达267 m Ah·g-1, 容量保持率为85%。
Yamamoto等[121]采用Ti对0.5Li2Mn O3-0.5Li Ni0.5Mn0.5O2材料中的Mn进行部分取代, 能谱 (EDS) 测试结果表明, Ti原子均匀取代部分Mn原子;Ti掺杂的样品在全电池中循环100周后具有较高的容量保持率, 而未掺杂的样品容量显著衰减, 这是由于Ti掺杂样品能够抑制过渡金属离子的溶解以及材料在隔膜和负极上的沉积, 从而改善了材料在全电池中的性能。Xiao等[122]通过计算表明, Ti掺杂后形成的Ti-O键较强, 能够抑制O的流失, 使材料的结构更加稳定。最近, Feng等[123]认为Ti4+的掺杂, 部分取代了Li1.2Mn0.54Co0.13Ni0.13O2中的Li+, 根据电中性原理, 一个Ti4+离子占据一个Li+离子的位置, 留下3个锂空位。
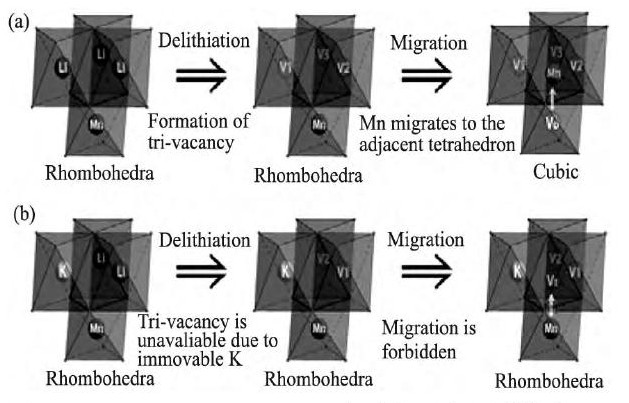
图1 1 Li1.20Mn0.54Co0.13Ni0.13O2相变的示意图 (其中V1, V2, V3代表锂层的空位;Vt代表四面体空位, Vo代表八面体空位) Fig.11Schematic diagrams of phase evolution routes for po-tassium-free (a) and potassium-doped (b) Li1.20Mn0.54Co0.13Ni0.13O2 (V1, V2and V3standing for va-cancies in lithium layer;Vtstanding for tetrahedral vacancy and Vofor octahedral vacancy)
Zhao等[124]用Sn4+对Li1.2Ni0.2Mn0.8O2进行掺杂改性, 充放电测试表明, 2.0%Sn掺杂的样品具有优异的循环稳定性, 在0.20C充放电倍率下循环160周, 材料的放电容量保持率高达98.5%, 图12[124]为不同Sn4+掺杂量样品的循环性能图。
He等[125]采用Zr对Li[Li0.2Mn0.54Ni0.13Co0.13]O2进行掺杂, 相关研究表明, Zr掺杂的样品在充放电循环过程中结构变化小, 并且锂离子扩散速率变化不大, 而未掺杂的样品, 在电流密度为250m A·g-1下循环100周后, 材料的锂离子扩散速率减小了一个数量级, 材料结构变化大。另外, Ma等[126]采用Mo5+对Li2Mn O3进行掺杂, XRD和X射线吸收谱测试表明, Mo掺杂使Li2Mn O3相的晶胞增大, 减少了Li-O和Mn-O的共键价成分, 从而抑制了材料氧的流失。
Kim等[127]通过共沉淀法成功合成F掺杂的0.3Li2Mn O3-0.7Li Mn0.60Ni0.25Co0.15O1.975F0.025, 电化学测试表明, F掺杂后材料的电化学性能得到改进, 这是因为F掺杂后材料的结构更加稳定, 表面能够形成更加致密的固态电解质 (SEI) 膜, 减少活性物质的溶解, 降低材料的内部阻抗。Zhang等[128]采用 (PO4) 3-对富锂锰基正极材料进行掺杂, 研究表明 (PO4) 3-掺杂的样品在循环过程中结构稳定, 作者认为, 这是因为 (PO4) 3-与过渡金属离子之间键的作用力更强, 掺杂后的样品的循环性能得到了极大的改善。Li等[120]成功合成Li[Li0.2Ni0.13Co0.13Mn0.54] (BO4) 0.75x (BO3) 0.25xO2-3.75x聚阴离子掺杂材料, 他们认为B掺杂在材料过渡金属层的四面体位, 通过第一性原理计算表明, 硼酸根阴离子掺杂后能够降低O2p轨道能级, 从而减少M-O键的共价键成分, 抑制了富锂材料中氧的析出, 减少材料在充放电循环中结构的破坏。掺杂后的材料具有优异的循环性能, 300周循环后材料的容量保持率高达89%, 如图13[120]所示。
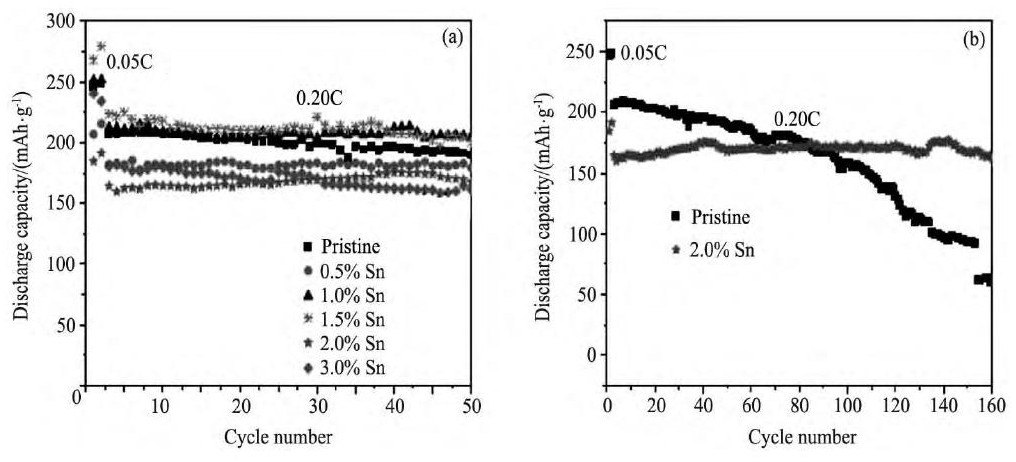
图1 2 Sn4+掺杂的Li1.2Ni0.2Mn0.8O2的循环性能Fig.12 Cycling performance of Sn4+doped-Li1.2Ni0.2Mn0.8O2 (0.20C, T=25℃)
最近, Hou等[129]将 (PO4) 3-以全浓度梯度的方式掺杂在富锂锰基正极材料中。通过控制 (PO4) 3-/ (Ni-Co-Mn) 的比例先制备出 (PO4) 3-以全浓度梯度分布的碳酸盐前驱体, 并成功制备出从材料颗粒内部到表面Ni, Co含量逐渐降低, Mn, P含量逐渐增加的目标产物, 如图14[129]所示。经过分析, 他们认为一部分P (2.0%) 进入材料的晶胞中占据锂层的锂位, 另一部分P (1.5%) 与材料中Li结合生成Li3PO4。 (PO4) 3-全浓度梯度掺杂的样品表现出优异的电化学性能, 在放电电流密度为100 m A·g-1的条件下循环400周后, 材料的容量保持率高达88.2%, 这是因为材料表面富Mn与 (PO4) 3-, 能够提升材料的结构稳定性。
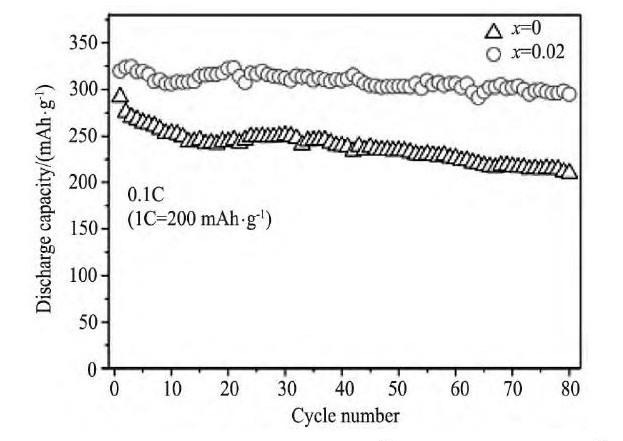
图1 3 硼含量为x=0和0.02的Li[Li0.2Ni0.13Co0.13Mn0.54] (BO4) 0.75x (BO3) 0.25xO2-3.75x, 在电流密度为20m A·g-1条件下的循环性能Fig.13Cycle performance of Li[Li0.2Ni0.13Co0.13Mn0.54] (BO4) 0.75x (BO3) 0.25xO2-3.75xwith x=0 and 0.02 at a current density of 20 m A·g-1
综上所述, 对富锂锰基正极材料进行掺杂可以有效改善材料的电化学性能。其改性机制可以作如下总结:Li位进行部分Na+, K+等掺杂, 使锂层间距增大, 有利于Li+的脱嵌;O位进行部分F-掺杂, F-掺杂后减少过渡金属的流失, 稳定材料结构;过渡金属八面体位进行阳离子掺杂, 掺杂元素与O形成的键较强, 减少了O的流失, 稳定材料结构;过渡金属的四面体位进行非金属元素 (比如B等) 掺杂, 形成聚阴离子, 可以降低O2p电子轨道的能级, 使过渡金属与O形成的键的共价键成分减少, 减少了O的流失, 稳定材料结构。
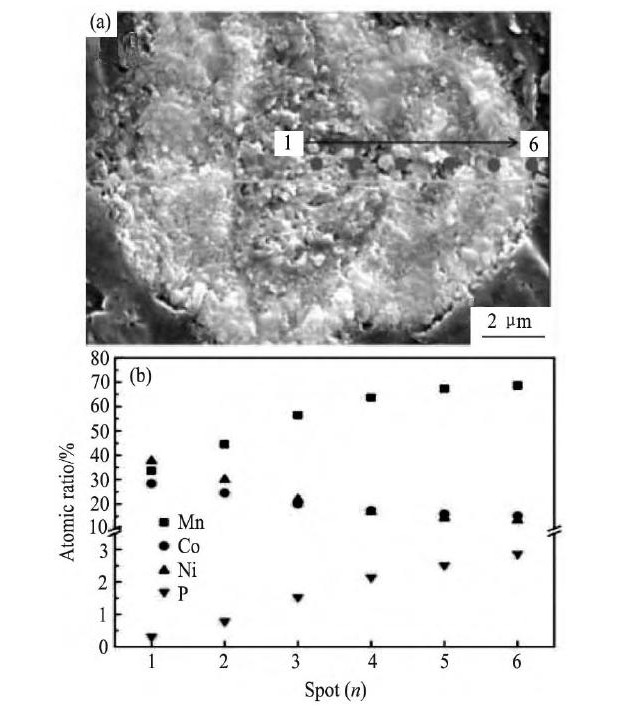
图1 4 (PO4) 3-全浓度梯度掺杂的样品横截面的SEM图和材料颗粒横截面的中心到边缘不同元素的原子比Fig.14SEM image of cross-section of a single CG-PO43-doped Li-rich layered oxide particle (a) and spot scanning EDS results on cross-section (b)
4.4 制备特殊形貌的材料
Wu课题组在制备具有特殊形貌的富锂锰基正极材料方面做了大量的工作。2014年, 该课题组[130]采用氢氧化物共沉淀法获得由纳米片组成的类球形的前驱体, 将该前驱体在500℃预烧 (低温预烧可以很好的保留前驱体的纳米片) , 之后配锂, 并在900℃焙烧, 得到目标产物。结合HR-TEM以及SAED测试, 作者认为Li1.2Ni0.2Mn0.6O2材料中纳米片的表面的晶面属于{010}晶面族, 这有利于锂离子的快速脱嵌, 而材料良好的倍率性能也证明了这一点。为了提高材料的结构稳定性, 2016年, 该课题组[131]设计出了一种梭型的纳米/微米富锂锰基正极材料, 并采用以尿素为螯合剂、聚乙烯吡咯烷酮 (PVP) 为形貌诱导剂的水热法成功制备出了形貌为梭型的正极材料Li1.2Ni0.2Mn0.6O2, 图15[131]为不同水热反应时间所合成的前驱体以及该前驱体焙烧成品的场发射扫描电镜 (FESEM) 图。该材料在0.1C倍率下循环100周, 容量保持率高到94%, 并且在5C的高倍率下放电容量也达到166.8 m Ah·g-1。作者把该材料的优异性能归功于其合成方法以及特别的梭型形貌, 他们认为, 该材料不但具有分布均匀的元素、密实的二次颗粒, 还具有稳定的几何外形。
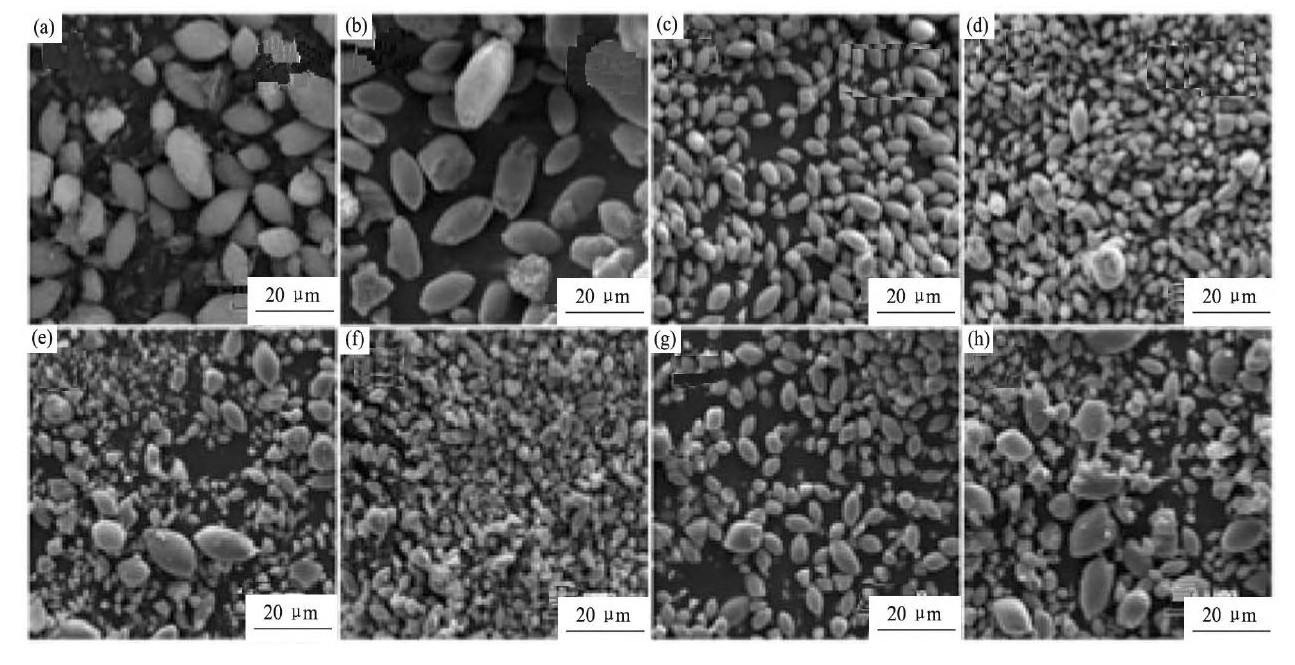
图1 5 不同水热反应时间2, 6, 10, 14 h所合成的碳酸盐前驱体以及该前驱体焙烧成品Li1.2Ni0.2Mn0.6O2的FESEM图Fig.15 FESEM images of carbonate precursors (a~d) and Li1.2Ni0.2Mn0.6O2powders (e~h) for different hydrothermal reaction time 2, 6, 10 and 14 h
5 结语
目前, 富锂锰基正极材料在结构研究、脱嵌锂机制研究、性能改进研究等方面取得了一定的进展。但是, 对富锂锰基正极材料的结构认识仍然存在争议, 是固溶体结构还是两相纳米复合结构尚无定论, 需进一步研究。材料首次充电后结构的变化以及后续循环过程中的脱嵌锂机制也尚不清楚, 均需作进一步研究。通过表面包覆、表面改性、掺杂以及特殊形貌的材料制备等手段, 材料的电化学性能得到很大的改进, 但是这些方法都是在实验室中进行的, 怎样应用于大规模的生产, 还需要付出巨大的努力。另外, 在循环过程中存在电压降, 目前还没有较有效的方法去抑制, 材料出现电压降的本质原因也不太清楚, 这些都需要进一步深入研究。
除了上述问题外, 富锂锰基正极材料对温度比较敏感, 尤其是超低温下材料的放电比容量下降严重, 对材料在低温下的充放电机制研究和性能改进, 也是未来的一个重要研究方向;充电至较高电压, O从材料晶格中脱出, 对材料的热稳定性产生影响, 因此需作进一步的研究;富锂锰基正极材料在半电池中的研究较多, 而在全电池中的研究较少, 材料最终要应用于全电池, 所以对全电池的研究非常重要;富锂锰基正极材料要真正应用于锂离子电池, 还需要新型高电压电解液与稳定的硅碳复合负极材料的协同开发。
参考文献
[1] Numata K, Sakaki C, Yamanaka S.Synthesis of solid solutions in a system of Li Co O2-Li2Mn O3for cathode materials of secondary lithium batteries[J].Chemistry Letters, 1997, 1997 (8) :725.
[2] Lu Z, Beaulieu L Y, Donaberger R A, Thomas C L, Dahn J R.Synthesis, structure, and electrochemical behavior of Li[NixLi1/3-2x/3Mn2/3-x/3]O2[J].Journal of the Electrochemical Society, 2002, 149 (6) :A778.
[3] Paulsen J, Mueller N J, Dahn J.Layered Li Co O2with a different oxygen stacking (O2structure) as a cathode material for rechargeable lithium batteries[J].Journal of the Electrochemical Society, 2000, 147 (2) :508.
[4] Reed J, Ceder G.Charge, potential, and phase stability of layered Li (Ni0.5Mn0.5) O2[J].Electrochemical and Solid-State Letters, 2002, 5 (7) :A145.
[5] Yu H, Wang Y, Asakura D, Hosono E, Zhang T, Zhou H.Electrochemical kinetics of the 0.5Li2Mn O3·0.5Li Mn0.42Ni0.42Co0.16O2‘composite’layered cathode material for lithium-ion batteries[J].RSC Advances, 2012, 2 (23) :8797.
[6] Yu H J, Zhou H S.High-energy cathode materials (Li2Mn O3-Li MO2) for lithium-ion batteries[J].The Journal of Physical Chemistry Letters, 2013, 4 (8) :1268
[7] Riou A, Lecerf A, Gerault Y, Cudennec Y.Etude structurale Li2Mn O3[J].Materials Research Bulletin, 1992, 27 (3) :269.
[8] Ito A, Li D, Sato Y.Cyclic deterioration and its improvement for Li-rich layered cathode material Li[Ni0.17Li0.2Co0.07Mn0.56]O2[J].Journal of Power Sources, 2010, 195:567.
[9] Koyama Y, Tanaka I, Adachi H.Crystal and electronic structures of superstructural Li1-x[Co1/3Ni1/3Mn1/3]O2 (0≤x≤1) [J].Journal of Power Sources, 2003, 119:644.
[10] Dai D, Li B, Tang H.Simultaneously improved capacity and initial coulombic efficiency of Li-rich cathode Li[Li0.2Mn0.54Co0.13Ni0.13]O2by enlarging crystal cell from a nanoplate precursor[J].Journal of Power Sources, 2016, 307:665.
[11] Chen C J, Pang W K, Mori T.The origin of capacity fade in the Li2Mn O3-Li MO2 (M=Li, Ni, Co, Mn) microsphere positive electrode:an operando neutron diffraction and transmission X-ray microscopy study[J].Journal of the American Chemical Society, 2016, 138 (28) :8824.
[12] Lu Z, Dahn J R.Structure and electrochemistry of layered Li[CrxLi (1/3-x/3) Mn (2/3-2x/3) ]O2[J].Journal of the Electrochemical Society, 2002, 149 (11) :A1454.
[13] Numata K, Sakaki C, Yamanaka S.Synthesis and characterization of layer structured solid solutions in the system of Li Co O2-Li2Mn O3[J].Solid State Ionics, 1999, 117 (3) :257.
[14] Wu F, Li N, Su Y, Shou H, Bao L, Yang W.Spinel/layered heterostructured cathode material for high-capacity and high-rate Li-ion batteries[J].Advanced Materials, 2013, 25 (27) :3722.
[15] Shi S J, Lou Z R, Xia T F, Wang X L, Gu C D, Tu JP.Hollow Li1.2Mn0.5Co0.25Ni0.05O2microcube prepared by binary template as a cathode material for lithium ion batteries[J].Journal of Power Sources, 2014, 257:198.
[16] Thackeray M M, Kang S H, Johnson C S, Vaughey J T, Benedek R, Hackney S A.Li2Mn O3-stabilized Li MO2 (M=Mn, Ni, Co) electrodes for lithium-ion batteries[J].Journal of Materials Chemistry, 2007, 17 (30) :3112.
[17] Boulineau A, Simonin L, Colin J F.Evolutions of Li1.2Mn0.61Ni0.18Mg0.01O2during the initial charge/discharge cycle studied byadvanced electron microscopy[J].Chemistry of Materials, 2012, 24 (18) :3558.
[18] Bare1o J, Balasubramanian M, Kang S H, Wen J G, Lei C H, Pol S V.Long-range and local structure in the layered oxide Li1.2Co0.4Mn0.4O2[J].Chemistry of Materials, 2011, 23 (8) :2039.
[19] Jarvis K A, Deng Z, Allard L F, Manthiram A, Ferreira P J.Atomic structure of a lithium-rich layered oxide material for lithium-ion batteries:evidence of a solid solution[J].Chemistry of Materials, 2011, 23 (16) :3614.
[20] Yu H J, Ishikawa R, So Y G, Shibata N, Kudo T, Zhou H.Direct atomic-resolution observation of two phases in the Li1.2Mn0.567Ni0.166Co0.067O2cathode material for lithium-ion batteries[J].Angewandte Chemie.2013, 52 (23) :5969.
[21] Yu H J, So YG, Kuwabara A, Tochigi E, Shibata N, Kudo T.Crystalline grain interior configuration affects lithium migration kinetics in Li-rich layered oxide[J].Nano Letters, 2016, 16 (5) :2907.
[22] Chen C, Chen S, Shui M, Xu X, Zheng W, Feng L.Comparative studies on potential dependent electrochemical impedance spectroscopy of cathode material0.5Li2Mn O3-0.5Li Ni0.5Mn0.5O2for the initial two charging cycles[J].Current Applied Physics, 2015, 15 (2) :149.
[23] Buchholz D, Li J, Passerini S, Aquilanti G, Wang D, Giorgetti M.X-ray absorption spectroscopy investigation of lithium-rich, cobalt-poor layered-oxide cathode material with high capacity[J].Chem Electro Chem, 2015, 2 (1) :85.
[24] Jarvis K A, Wang C C, Manthiram A, Ferreira P J.The role of composition in the atomic structure, oxygen loss, and capacity of layered Li-Mn-Ni oxide cathodes[J].J.Mater.Chem.A, 2014, 2 (5) :1353.
[25] DupréN, Cuisinier M, Legall E, War D, Guyomard D.Contribution of the oxygen extracted from overlithiated layered oxides at high potential to the formation of the interphase[J].Journal of Power Sources, 2015, 299:231.
[26] Mohanty D, Sefat A S, Payzant E A, Li J, Wood D L, Daniel C.Unconventional irreversible structural changes in a high-voltage Li-Mn-rich oxide for lithium-ion battery cathodes[J].Journal of Power Sources, 2015, 283:423.
[27] Xu G, Li J, Li X, Zhou H, Ding X, Wang X.Understanding the electrochemical superiority of 0.6Li[Li1/3Mn2/3]O2-0.4Li[Ni1/3Co1/3Mn1/3]O2nanofibers as cathode material for lithium ion batteries[J].Electrochimica Acta, 2015, 173:672.
[28] Robertson A D, Bruce P G.Mechanism of electrochemical activity in Li2Mn O3[J].Chemistry of Materials, 2003, 15 (10) :1984.
[29] Dogan F, Croy J R, Balasubramanian M, Slater M D, Iddir H, Johnson C S, Key B.Solid state NMR studies of Li2Mn O3and Li-rich cathode materials:proton insertion, local structure, and voltage fade[J].Journal of the Electrochemical Society, 2015, 162 (1) :A235.
[30] Croy J R, Park J S, Dogan F, Johnson C S, Key B, Balasubramanian M.First-cycle evolution of local structure in electrochemically activated Li2Mn O3[J].Chemistry of Materials, 2014, 26 (24) :7091.
[31] Armstrong A R, Holzapfel M, Bruce P G.Demonstrating oxygen loss and associated structural reorganization in the lithium battery cathode Li[Ni0.2Li0.2Mn0.6]O2[J].J.Am.Chem.Soc., 2006, 128 (5) :8694.
[32] La Mantia F, Rosciano F, Tran N.Direct evidence of oxygen evolution from Li1+x (Ni1/3Mn1/3Co1/3) 1-xO2at high potentials[J].J.Appl.Electrochem., 2008, 38 (2) :893.
[33] Park M S, Lee J W, Park K S, Im D, Doo S G, Park K S.On the surface modifications of high-voltage oxide cathodes for lithium-ion batteries:new insight and significant safety improvement[J].J.Mater.Chem., 2010, 20 (3) :7208.
[34] Konishi H, Hirano T, Takamatsu D, Gunji A, Feng X, Furutsuki S.Effect of composition of transition metals on stability of charged Li-rich layer-structured cathodes Li1.2Ni0.2-xMn0.6-xCo2xO2 (x=0, 0.033, and 0.067) at high temperatures[J].Electrochimica Acta, 2015, 186:591.
[35] Koyama Y, Tanaka I, Nagao M, Kanno R.First-principles study on lithium removal from Li2Mn O3[J].J.Power Sources, 2009, 189:798.
[36] Seo DH, Lee J, Urban A, Malik R, Kang S, Ceder G.The structural and chemical origin of the oxygen redox activity in layered and cation-disordered Li-excess cathode materials[J].Nature Chemistry, 2016, 8 (7) :692.
[37] Yabuuchi N, Yoshii K, Myung S T, Nakai I, Komaba S.Detailed studies of a high-capacity electrode material for rechargeable batteries, Li2Mn O3-Li Co1/3Ni1/3Mn1/3O2[J].Journal of the American Chemical Society, 2011, 133 (12) :4404.
[38] Idemoto Y, Yamamoto R, Ishida N, Kitamura N.Change in local structure of 0.4Li2Mn O3-0.6Li Mn1/3Ni1/3Co1/3O2during first discharge process[J].Electrochimica Acta, 2015, 153:399.
[39] Zhang L, Jin K, Wang L, Zhang Y, Li X, Song Y.High capacity Li1.2Mn0.54Ni0.13Co0.13O2cathode materials synthesized using mesocrystal precursors for lithium-ion batteries[J].Journal of Alloys and Compounds, 2015, 638 (3) :298.
[40] Gu R M, Yan S Y, Sun S, Wang C Y, Li M W.Electrochemical behavior of lithium-rich layered oxide Li[Li0.23Ni0.15Mn0.62]O2cathode material for lithium-ion battery[J].Journal of Solid State Electrochemistry, 2015, 19 (6) :1659.
[41] Genevois C, Koga H, Croguennec L, Ménétrier M, Delmas C, Weill F.Insight into the atomic structure of cycled lithium-rich layered oxide Li1.20Mn0.54Co0.13Ni0.13O2using HAADF STEM and electron nanodiffraction[J].The Journal of Physical Chemistry C, 2015, 119 (1) :75.
[42] Wang R, He X, He L, Wang F, Xiao R, Gu L.Atomic structure of Li2Mn O3after partial delithiation and Re-lithiation[J].Advanced Energy Materials, 2013, 3 (10) :1358.
[43] Muhammad S, Kim H, Kim Y, Kim D, Song J H, Yoon J.Evidence of reversible oxygen participation in anomalously high capacity Li-and Mn-rich cathodes for Li-ion batteries[J].Nano Energy, 2016, 21 (1) :172.
[44] Hy S, Felix F, Rick J, Su W N, Hwang B J.Direct in situ observation of Li2O evolution on Li-rich high-capacity cathode material, Li[Ni (x) Li ( (1-2x) /3) Mn ( (2-x) /3) ]O2 (0≤x≤0.5) [J].Journal of the American Chemical Society, 2014, 136 (3) :999.
[45] Oishi M, Yogi C, Watanabe I, Ohta T, Orikasa Y, Uchimoto Y.Direct observation of reversible charge compensation by oxygen ion in Li-rich manganese layered oxide positive electrode material, Li1.16Ni0.15Co0.19Mn0.50O2[J].Journal of Power Sources, 2015, 276:89.
[46] Han S, Xia Y, Wei Z, Qiu B, Pan L, Gu Q.A comparative study on the oxidation state of lattice oxygen among Li1.14Ni0.136Co0.136Mn0.544O2, Li2Mn O3, Li Ni0.5Co0.2Mn0.3O2and Li Co O2for the initial charge-discharge[J].J.Mater.Chem.A, 2015, 3 (22) :11930.
[47] Luo K, Roberts M R, Hao R, Guerrini N, Pickup D M, Liu Y S.Charge-compensation in 3d-transition-metaloxide intercalation cathodes through the generation of localized electron holes on oxygen[J].Nature Chemistry, 2016, 8 (7) :684.
[48] Shimoda K, Minato T, Nakanishi K, Komatsu H, Matsunaga T, Tanida H.Oxidation behaviour of lattice oxygen in Li-rich manganese-based layered oxide studied by hard X-ray photoelectron spectroscopy[J].J.Mater.Chem.A, 2016, 4 (16) :5909.
[49] Ates M N, Mukerjee S, Abraham K M.A Li-rich layered cathode material with enhanced structural stability and rate capability for Li-ion batteries[J].Journal of the Electrochemical Society, 2014, 161 (3) :A355.
[50] Liu J, Chen H, Xie J, Sun Z, Wu N, Wu B.Electrochemical performance studies of Li-rich cathode materials with different primary particle sizes[J].Journal of Power Sources, 2014, 251:208.
[51] Zhu Z, Zhu L.Synthesis of layered cathode material0.5Li2Mn O3-0.5Li Mn1/3Ni1/3Co1/3O2by an improved coprecipitation method for lithium-ion battery[J].Journal of Power Sources, 2014, 256:178.
[52] Chen C, Chen S, Luo J, Xu X, Hu D, Shui M.The preparation and electro-chemical performance of lithium rich cathode material Li (Li0.3Ni0.2Mn0.5Co0.1) O2prepared at strictly controlled p H level[J].Ceramics International, 2015, 41 (8) :10319.
[53] Chen Y, Chen Z, Xie K.Effect of annealing on the first-cycle performance and reversible capabilities of lithium-rich layered oxide cathodes[J].The Journal of Physical Chemistry C, 2014, 118 (22) :11505.
[54] Wang J, Yuan G, Zhang M, Qiu B, Xia Y, Liu Z.The structure, morphology, and electrochemical properties of Li1+xNi1/6Co1/6Mn4/6O2.25+x/2 (0.1≤x≤0.7) cathode materials[J].Electrochimica Acta, 2012, 66:61.
[55] Ma D, Zhang P, Li Y, Ren X.Li1.2Mn0.54Ni0.13Co0.13O2-encapsulated carbon nanofiber network cathodes with improved stability and rate capability for Li-ion batteries[J].Scientific Reports, 2015, 5 (2) :11257.
[56] Chen Y, Xie K, Zheng C, Ma Z, Chen Z.Enhanced Li storage performance of Li N0.5Mn1.5O4-coated0.4Li2Mn O3-0.6Li Ni1/3Co1/3Mn1/3O2cathode materials for Li-ion batteries[J].ACS Appl.Mater.Interfaces, 2014, 6 (19) :16888.
[57] Zhao T, Chen S, Li L, Zhang X, Chen R, Belharouak I, Wu F, Amine K.Synthesis, characterization, and electrochemistry of cathode material Li[Li0.2Co0.13Ni0.13Mn0.54]O2using organic chelating agents for lithium-ion batteries[J].Journal of Power Sources, 2013, 228:206.
[58] Yang F, Zhang Q, Hu X, Peng T.Synthesis of layered x Li2Mn O3- (1-x) Li Mn O2nanoplates and its electrochemical performance as Li-rich cathode materials for Li-ion battery[J].Electrochimica Acta, 2015, 165:182.
[59] Noh J K, Kim S, Kim H, Choi W, Chang W, Byun D.Mechanochemical synthesis of Li2Mn O3shell/Li MO2 (M=Ni, Co, Mn) core-structured nanocomposites for lithium-ion batteries[J].Scientific Reports, 2014, 4 (1) :4847.
[60] Zhao C, Wang X, Liu R, Xu F, Shen Q.β-Mn O2sacrificial template synthesis of Li1.2Ni0.13Co0.13Mn0.54O2for lithium ion battery cathodes[J].RSC Advances, 2014, 4 (14) :7154.
[61] Zheng J, Gu M, Genc A, Xiao J, Xu P, Chen X.Mitigating voltage fade in cathode materials by improving the atomic level uniformity of elemental distribution[J].Nano Letters, 2014, 14 (5) :2628.
[62] Kim M G, Jo M, Hong Y S, Cho J.Template-free synthesis of Li[Ni0.25Li0.15Mn0.6]O2nanowires for high performance lithium battery cathode[J].Chemical Communications, 2009, 10 (2) :218.
[63] Lengyel M, Atlas G, Elhassid D, Luo P Y, Zhang X, Belharouak I.Effects of synthesis conditions on the physical and electrochemical properties of Li1.2Mn0.54Ni0.13Co0.13O2prepared by spray pyrolysis[J].Journal of Power Sources, 2014, 262:286.
[64] Fu C, Li G, Luo D, Zheng J, Li L.Gel-combustion synthesis of Li1.2Mn0.4Co0.4O2composites with a high capacity and superior rate capability for lithium-ion batteries[J].J.Mater.Chem.A, 2014, 2 (5) :1471.
[65] Nayak P K, Grinblat J, Levi M, Aurbach D.Electrochemical and structural characterization of carbon coated Li1.2Mn0.56Ni0.16Co0.08O2and Li1.2Mn0.6Ni0.2O2as cathode materials for Li-ion batteries[J].Electrochimica Acta, 2014, 137:546.
[66] Zhao W, Harada S, Furuya Y, Yamamoto S, Noguchi H.A new route for the synthesis of Li2Mn O3based cathode material with enhanced first cycle efficiency and cycleability for lithium ion batteries[J].Journal of Power Sources, 2014, 261:324.
[67] Kim D, Kang S H, Balasubramanian M, Johnson C S.High-energy and high-power Li-rich nickel manganese oxide electrode materials[J].Electrochemistry Communications, 2010, 12 (11) :1618.
[68] Wang Z Y, Biao L, Jin M, Xia D G.Molten salt synthesis and high-performance of nanocrystalline Li-rich cathode materials[J].RSC Advances, 2014, 4 (30) :15825.
[69] Miao X, Yan Y, Wang C, Cui L, Fang J, Yang G.Optimal microwave-assisted hydrothermal synthesis of nanosized x Li2Mn O3· (1-x) Li Ni1/3Co1/3Mn1/3O2cathode materials for lithium ion battery[J].Journal of Power Sources, 2014, 247:219.
[70] Yuan X, Xu Q J, Wang C, Liu X, Liu H, Xia Y.A facile and novel organic coprecipitation strategy to prepare layered cathode material Li[Li0.2Mn0.54Ni0.13Co0.13]O2with high capacity and excellent cycling stability[J].Journal of Power Sources, 2015, 279:157.
[71] Yu X, Lyu Y, Gu L, Wu H, Bak S M, Zhou Y.Understanding the rate capability of high-energy-density Lirich layered Li1.2Ni0.15Co0.1Mn0.55O2cathode materials[J].Advanced Energy Materials, 2014, 4 (5) :134.
[72] Yang F, Liu Y, Martha S K, Wu Z, Andrews J C, Ice G E.Nanoscale morphological and chemical changes of high voltage lithium-manganese rich NMC composite cathodes with cycling[J].Nano Letters, 2014, 14 (8) :4334.
[73] Boulineau A, Simonin L, Colin J F, Canévet E, Daniel L, Patoux S.Evolutions of Li1.2Mn0.61Ni0.18Mg0.01O2during the initial charge/discharge cycle studied by advanced electron microscopy[J].Chemistry of Materials, 2012, 24 (18) :3558.
[74] Xu B, Fell C R, Chi M, Meng Y S.Identifying surface structural changes in layered Li-excess nickel manganese oxides in high voltage lithium ion batteries:a joint experimental and theoretical study[J].Energy&Environmental Science, 2011, 4 (6) :2223.
[75] Li X, Liu J, Meng X, Tang Y, Banis M N.Yang J.Significant impact on cathode performance of lithium-ion batteries by precisely controlled metal oxide nanocoatings via atomic layer deposition[J].Journal of Power Sources, 2014, 247:57.
[76] Choi M, Ham G, Jin B S, Lee S M, Lee Y M, Wang G.Ultra-thin Al2O3coating on the acid-treated0.3Li2Mn O3·0.7Li Mn0.60Ni0.25Co0.15O2electrode for Li-ion batteries[J].Journal of Alloys and Compounds, 2014, 608 (2) :110.
[77] Myung S T, Izumi K, Komaba S, Sun Y K, Yashiro H, Kumagai N.Role of alumina coating on Li-Ni-Co-MnO particles as positive electrode material for lithium-ion batteries[J].Chemistry of Materials, 2005, 17 (14) :3695.
[78] Zhao J, Aziz S, Wang Y.Hierarchical functional layers on high-capacity lithium-excess cathodes for superior lithium ion batteries[J].Journal of Power Sources, 2014, 247:95.
[79] Guo S, Yu H, Liu P, Liu X, Li D, Chen M.Surface coating of lithium-manganese-rich layered oxides with delaminated Mn O2nanosheets as cathode materials for Liion batteries[J].Journal of Materials Chemistry A.2014, 2 (12) :4422.
[80] Liu Y, Gao Y, Wang Q, Dou A.Influence of coated Mn O2content on the electrochemical performance of Li1.2Ni0.2Mn0.6O2cathodes[J].Ionics, 2013, 20 (6) :825.
[81] Wu F, Wang Z, Su Y, Yan N, Bao L, Chen S.Li[Li0.2Mn0.54Ni0.13Co0.13]O2-Mo O3composite cathodes with low irreversible capacity loss for lithium ion batteries[J].Journal of Power Sources, 2014, 247:20.
[82] Yuan W, Zhang H Z, Liu Q, Li G R, Gao X P.Surface modification of Li (Li0.17Ni0.2Co0.05Mn0.58) O2with Ce O2as cathode material for Li-ion batteries[J].Electrochimica Acta, 2014, 135:199.
[83] He F, Wang X, Du C, Baker A P, Wu J, Zhang X.The effect of samaria doped ceria coating on the performance of Li1.2Ni0.13Co0.13Mn0.54O2cathode material for lithium-ion battery[J].Electrochimica Acta.2015, 153:484.
[84] Wu F, Li N, Su Y, Lu H, Zhang L, An R.Can surface modification be more effective to enhance the electrochemical performance of lithium rich materials[J].Journal of Materials Chemistry, 2012, 22 (4) :1489.
[85] Shin D, Wolverton C, Croy J R, Balasubramanian M, Kang S H, Rivera C M.First-principles calculations, electrochemical and X-ray absorption studies of Li-NiPO4surface-treated x Li2Mn O3· (1-x) Li MO2 (M=Mn, Ni, Co) electrodes for Li-ion batteries[J].Journal of the Electrochemical Society, 2012, 159 (2) :A121.
[86] Liu X, Huang T, Yu A.Surface phase transformation and Ca F2coating for enhanced electrochemical performance of Li-rich Mn-based cathodes[J].Electrochimica Acta, 2015, 163:82.
[87] Zheng J, Gu M, Xiao J, Polzin B J, Yan P, Chen X.Functioning mechanism of Al F3coating on the Li-and Mn-rich cathode materials[J].Chemistry of Materials, 2014, 26 (22) :6320.
[88] Li G, Feng X, Ding Y, Ye S, Gao X.Al F3-coated Li (Li0.17Ni0.25Mn0.58) O2as cathode material for Li-ion batteries[J].Electrochimica Acta, 2012, 78:308.
[89] Lu C, Wu H, Chen B, Liu H, Zhang Y.Improving the electrochemical properties of Li1.2Mn0.52Co0.08Ni0.2O2cathode material by uniform surface nanocoating with samarium fluoride through depositional-hydrothermal route[J].Journal of Alloys and Compounds, 2015, 634 (1) :75.
[90] Wang D, Wang X, Yang X, Yu R, Ge L, Shu H.Polyaniline modification and performance enhancement of lithium-rich cathode material based on layered-spinel hybrid structure[J].Journal of Power Sources, 2015, 293:89.
[91] Ahn D, Koo Y M, Kim M G, Shin N, Park J, Eom J.Polyaniline nanocoating on the surface of layered Li[Li0.2Co0.1Mn0.7]O2nanodisks and enhanced cyclability as a cathode electrode for rechargeable lithium-ion battery[J].The Journal of Physical Chemistry C, 2010, 114 (8) :3675.
[92] Liu Y, Wang Q, Lu Y, Yang B, Su M, Gao Y.Enhanced electrochemical performances of layered cathode material Li1.5Ni0.25Mn0.75O2.5by coating with Li Al O2[J].Journal of Alloys and Compounds, 2015, 638 (1) :1.
[93] Zhang X, Sun S, Wu Q, Wan N, Pan D, Bai Y.Improved electrochemical and thermal performances of lay-ered Li[Li0.2Ni0.17Co0.07Mn0.56]O2via Li2Zr O3surface modification[J].Journal of Power Sources, 2015, 282:378.
[94] Zhao E, Liu X, Hu Z, Sun, Xiao X.Facile synthesis and enhanced electrochemical performances of Li2Ti O3-coated lithium-rich layered Li1.13Ni0.30Mn0.57O2cathode materials for lithium-ion batteries[J].Journal of Power Sources, 2015, 294:141.
[95] Cong L N, Gao X G, Ma S C, Guo X, Zeng Y P, Tai L H.Enhancement of electrochemical performance of Li[Li0.2Mn0.54Ni0.13Co0.13]O2by surface modification with Li4Ti5O12[J].Electrochimica Acta, 2014, 115:399.
[96] Fu Q, Du F, Bian X, Wang Y, Yan X, Zhang Y.Electrochemical performance and thermal stability of Li1.18Co0.15Ni0.15Mn0.52O2surface coated with the ionic conductor Li3VO4[J].Journal of Materials Chemistry A, 2014, 2 (20) :7555.
[97] Kang S H, Thackeray M M.Enhancing the rate capability of high capacity x Li2Mn O3· (1-x) Li MO2 (M=Mn, Ni, Co) electrodes by Li-Ni-PO4treatment[J].Electrochemistry Communications, 2009, 11 (4) :748.
[98] Liu J, Reeja J B, Manthiram A.Conductive surface modification with aluminum of high capacity layered Li[Li0.2Mn0.54Ni0.13Co0.13]O2cathodes[J].The Journal of Physical Chemistry C, 2010, 114 (20) :9528.
[99] Guo L, Zhao N, Li J, He C, Shi C, Liu E.Surface double phase network modified lithium rich layered oxides with improved rate capability for Li-ion batteries[J].ACS Appl.Mater.Interfaces, 2015, 7 (1) :391.
[100] Song B, Lai M O, Liu Z, Liu H, Lu L.Graphenebased surface modification on layered Li-rich cathode for high-performance Li-ion batteries[J].Journal of Materials Chemistry A, 2013, 1 (34) :9954.
[101] Yu R, Lin Y, Huang Z.Investigation on the enhanced electrochemical performances of Li1.2Ni0.13Co0.13Mn0.54O2by surface modification with Zn O[J].Electrochimica Acta, 2015, 173:515.
[102] Wang Q Y, Liu J, Murugan A V, Manthiram A.High capacity double-layer surface modified Li[Li0.2Mn0.54Ni0.13Co0.13]O2cathode with improved rate capability[J].Journal of Materials Chemistry.2009, 19 (28) :4965.
[103] Sun Y K, Lee M J, Yoon C S, Hassoun J, Amine K, Scrosati B.The role of Al F3coatings in improving electrochemical cycling of Li-enriched nickel-manganese oxide electrodes for Li-ion batteries[J].Advanced Materials, 2012, 24 (9) :1192.
[104] Qiao Q Q, Li G R, Wang Y L.To enhance the capacity of Li-rich layered oxides by surface modification with metal-organic frameworks (MOFs) as cathodes for advanced lithium-ion batteries[J].Journal of Materials Chemistry A, 2016, 4 (12) :4440.
[105] Johnson C S, Kim J S, Lefief C.The significance of the Li2Mn O3component in‘composite’x Li2Mn O3· (1-x) Li Mn0.5Ni0.5O2electrodes[J].Electrochem.Comm., 2004, 6 (1) :1085.
[106] Zheng J, Deng S, Shi Z.The effects of persulfate treatment on the electrochemical properties of Li[Li0.2Mn0.54Ni0.13Co0.13]O2cathode material[J].Journal of Power Sources, 2013, 221:108.
[107] Abouimrane A, Compton O C, Deng H, Belharouak I, Dikin D A, Nguyen S T, Amine K.Improved rate capability in a high-capacity layered cathode material via thermal reduction[J].Electrochemical and Solid-State Letters, 2011, 14 (9) :A126.
[108] Song B, Liu H, Liu Z, Xiao P, Lai M O, Lu L.High rate capability caused by surface cubic spinels in Lirich layer-structured cathodes for Li-ion batteries[J].Scientific Reports, 2013, 3:3094.
[109] Qiu B, Zhang M, Wu L, Wang J, Xia Y, Qian D.Gas-solid interfacial modification of oxygen activity in layered oxide cathodes for lithium-ion batteries[J].Nature Communications, 2016, 7 (1) :12108.
[110] Lim S N, Seo J Y, Jung D S, Ahn W, Song H S, Yeon S H.Rate capability for Na-doped Li1.167Ni0.18Mn0.548Co0.105O2cathode material and characterization of Li-ion diffusion using galvanostatic intermittent titration technique[J].Journal of Alloys and Compounds, 2015, 623 (2) :55.
[111] Li Q, Li G, Fu C, Luo D, Fan J, Li L.K+-doped Li1.2Mn0.54Co0.13Ni0.13O2:a novel cathode material with an enhanced cycling stability for lithium-ion batteries[J].ACS Appl.Mater.Interfaces, 2014, 6 (13) :10330.
[112] Chen M, Chen D, Liao Y, Huang Q, Li W.Influence of Fe substitution on cycling stability of Li[Li0.2Ni0.13Mn0.54Co0.13]O2cathode for lithium ion batteries[J].Ionics, 2015, 21 (7) :1827.
[113] Tabuchi M, Kitta M, Kageyama H, Shibuya H, Imaizumi J.Mn source effects on electrochemical properties of Fe-and Ni-substituted Li2Mn O3positive electrodematerial[J].Journal of Power Sources, 2015, 279:510.
[114] Zhao J, Wang Z, Guo H, Li X, He Z, Li T.Synthesis and electrochemical characterization of Zn-doped Lirich layered Li[Li0.2Mn0.54Ni0.13Co0.13]O2cathode material[J].Ceramics International, 2015, 41 (9) :11396.
[115] Park J H, Lim J, Yoon J, Park K S, Gim J, Song J.The effects of Mo doping on 0.3Li[Li0.33Mn0.67]O2·0.7Li[Ni0.5Co0.2Mn0.3]O2cathode material[J].Dalton Transactions, 2012, 41 (10) :3053.
[116] Park S H, Sun Y K.Synthesis and electrochemical properties of layered Li[Li0.15Ni (0.275-x/2) AlxMn (0.575-x/2) ]O2materials prepared by sol-gel method[J].Journal of Power Sources, 2003, 119:161.
[117] Jiao L F, Zhang M, Yuan H T, Zhao M, Guo J, Wang W.Effect of Cr doping on the structural, electrochemical properties of Li[Li0.2Ni0.2-x/2Mn0.6-x/2Crx]O2 (x=0, 0.02, 0.04, 0.06, 0.08) as cathode materials for lithium secondary batteries[J].Journal of Power Sources, 2007, 167:178.
[118] Kang S H, Amine K.Layered Li (Li0.2Ni0.15+0.5z Co0.10Mn0.55-0.5z) O2-z Fzcathode materials for Li-ion secondary batteries[J].Journal of Power Sources, 2005, 146:654.
[119] Lim S N, Seo J Y, Jung D S, Park S B, Yeon S H.The crystal structure and electrochemical performance of Li1.167Mn0.548Ni0.18Co0.105O2composite cathodes doped and co-doped with Mg and F[J].Journal of Electroanalytical Chemistry, 2015, 740 (1) :88.
[120] Li B, Yan H, Ma J, Yu P, Xia D, Huang W.Manipulating the electronic structure of Li-rich manganesebased oxide using polyanions:towards better electrochemical performance[J].Advanced Functional Materials, 2014, 24 (32) :5112.
[121] Yamamoto S, Noguchi H, Zhao W.Improvement of cycling performance in Ti substituted 0.5Li2Mn O3-0.5Li Ni0.5Mn0.5O2through suppressing metal dissolution[J].Journal of Power Sources, 2015, 278:76.
[122] Xiao P, Deng Z Q, Manthiram A, Henkelman G.Calculations of oxygen stability in lithium-rich layered cathodes[J].The Journal of Physical Chemistry C, 2012, 116 (44) :23201.
[123] Feng X, Gao Y, Ben L, Yang Z, Wang Z, Chen L.Enhanced electrochemical performance of Ti-doped Li1.2Mn0.54Co0.13Ni0.13O2for lithium-ion batteries[J].Journal of Power Sources, 2016, 317:74.
[124] Zhao Y, Xia M, Hu X, Zhao Z, Wang Y, Lv Z.Effects of Sn doping on the structural and electrochemical properties of Li1.2Ni0.2Mn0.8O2Li-rich cathode materials[J].Electrochimica Acta, 2015, 174:1167.
[125] He Z, Wang Z, Chen H, Huang Z, Li X, Guo H.Electrochemical performance of zirconium doped lithium rich layered Li1.2Mn0.54Ni0.13Co0.13O2oxide with porous hollow structure[J].Journal of Power Sources, 2015, 299:334.
[126] Ma J, Zhou Y N, Gao Y, Kong Q, Wang Z, Yang X Q.Molybdenum substitution for improving the charge compensation and activity of Li2Mn O3[J].Chemistry, 2014, 20 (28) :8723.
[127] Kim S M, Jin B S, Lee S M, Kim H S.Effects of the fluorine-substitution and acid treatment on the electrochemical performances of 0.3Li2Mn O3·0.7Li Mn0.60Ni0.25Co0.15O2cathode material for Li-ion battery[J].Electrochimica Acta, 2015, 171:35.
[128] Zhang H Z, Qiao Q Q, Li G R, Gao X P.PO3-4polyanion-doping for stabilizing Li-rich layered oxides as cathode materials for advanced lithium-ion batteries[J].Journal of Materials Chemistry A, 2014, 2 (20) :7454.
[129] Hou P, Li G, Gao X.Tailoring atomic distribution in micron-sized and spherical Li-rich layered oxides as cathode materials for advanced lithium-ion batteries[J].Journal of Materials Chemistry A, 2016, 4 (20) :7689.
[130] Chen L, Su Y, Chen S, Li N, Bao L, Li W.Hierarchical Li1.2Ni0.2Mn0.6O2nanoplates with exposed{010}planes as high-performance cathode material for lithiumion batteries[J].Advanced Materials, 2014, 26 (39) :6756.
[131] Li Y, Bai Y, Wu C.Three-dimensional fusiform hierarchical micro/nano Li1.2Ni0.2Mn0.6O2with a preferred orientation (110) plane as a high energy cathode material for lithium-ion batteries[J].Journal of Materials Chemistry A, 2016, 4 (16) :5942.