- Abstract:
- 1 Introduction▲
- 2 Experimental▲
- 3 Results and discussion▲
- 3.1 Influence of pH on distribution coefficient (D)
- 3.2 Isotherm adsorption curve
- 3.3 Determination of adsorption rate constant at different temperatures and apparent activation energies
- 3.4 Influence of adsorption temperature on distribution coefficient and determination of thermodynamic parameters
- 3.5 Effect of shaking speed on adsorption rate
- 3.6 Elution
- 3.7 Dynamic adsorption and desorption
- 4 Conclusions▲
- References
- Figure
- Fig.1 Influence of pH on distribution coefficient of Pb2+ adsorption by D152 resin (resin 15.0 mg, T=298 K, C0=0.333 mg/mL, r=100 r/min)
- Fig.2 Langmuir plot for adsorption of Pb2+ by D152 resin (resin 15.0 mg, r=100 r/min, pH 6.00)
- Fig.3 Adsorption rate curves of Pb2+ adsorption by D152 resin (C0(Pb2+)=0.333 mg/mL, resin 30.0 mg, pH 6.00)
- Fig.4 Determination of adsorption rate constant of Pb2+ adsorption by D152 resin (C0(Pb2+)=0.333 mg/mL, resin 30.0 mg, pH 6.00)
- Fig.5 Linear plot of lg k vs 1/T for adsorption of Pb2+ by D152 resin (C0(Pb2+)=0.333 mg/mL, resin 30.0 mg, pH 6.00)
- Fig.6 Influence of temperature on distribution coefficient of Pb2+ adsorption by D152 resin (C0(Pb2+)=0.333 mg/mL, resin 15.0 mg, pH 6.00)
- Fig.7 Influence of shaking speed on Pb2+ adsorption rate by D152 resin (C0(Pb2+)=0.333 mg/mL, resin 30.0 mg, pH 6.00, T=298 K)
- Fig.8 Dynamic adsorption curve for Pb2+ adsorption by D152 resin at C2=0.200 mg/mL and flow rate of 0.25 mL/min
- Fig.9 Dynamic desorption curve for Pb2+ adsorption by D152 resin at flow rate of 0.15 mL/min
J. Cent. South Univ. Technol. (2009) 16: 0569-0574
DOI: 10.1007/s11771-009-0095-2
Adsorption of Pb2+ on macroporous weak acid adsorbent resin from aqueous solutions: Batch and column studies
XIONG Chun-hua(熊春华), FENG Yu-jie(冯宇杰), YAO Cai-ping(姚彩萍)
(Department of Applied Chemistry, Zhejiang Gongshang University, Hangzhou 310012, China)
Abstract:
The adsorption properties of a novel macroporous weak acid resin (D152) for Pb2+ were investigated with chemical methods. The optimal adsorption condition of D152 resin for Pb2+ is at pH 6.00 in HAc-NaAc medium. The statically saturated adsorption capacity is 527 mg/g at 298 K. Pb2+ adsorbed on D152 resin can be eluted with 0.05 mol/L HCl quantitatively. The adsorption rate constants determined under various temperatures are k288 K=2.22×10-5 s-1, k298 K=2.51×10-5 s-1, and k308 K= 2.95×10-5 s-1, respectively. The apparent activation energy, Ea is 10.5 kJ/mol, and the adsorption parameters of thermodynamics are ?HΘ=13.3 kJ/mol, ?SΘ=119 J/(mol?K), and ?GΘ298 K =-22.2 kJ/mol, respectively. The adsorption behavior of D152 resin for Pb2+ follows Langmuir model.
Key words:
Pb2+; macroporous weak acid resin; adsorption; thermodynamics;
1 Introduction
The contamination of water recourses by heavy metal is a serious worldwide environmental problem. Lead is one of the most important pollutants and is of particular interest because of its toxicity and widespread presence in the environment [1]. The removal of heavy metal ions from contaminated water can be achieved by several processes, such as chemical precipitation [2], solvent extraction [3], membrane separation [4] and electrochemical reduction [5]. Most of these processes may be ineffective, extremely expensive, or generate secondary pollution. In recent years, the adsorption process has received much attention and becomes one of the more popular methods for the removal of heavy metals from the wastewater because of its competitive and effective process for the above purpose. Though numerous adsorbents have been reported for the removal of Pb2+, such as activated carbon [6], chitosan [7] and clay [8], additional alternative materials for this purpose are still needed. An effective adsorbing material should consist of a stable and insoluble matrix. And polymeric materials have been demonstrated to be such kind of material [9-11]. Many studies have shown the functionalization of a polymeric matrix with different chelating functionalities for metal ions removal or separation [12-15]. As one of these materials, macroporous weak acid resin (D152) is an excellent absorbent because of its strong mechanical stability to high linear flow rates, and high chemical resistibility to harsh conditions. D152 resin contains the functional group of —COOH, which possesses not only protons that can exchange with cations, but also oxygen atoms that can coordinate directly with metal ions. Therefore, the adsorption ability of D152 resin for enriching metal ion may be very strong. In addition, D152 resin is also inexpensive and easy to regenerate.
Until now, little work has been published on the application of D152 resin for the removal of Pb2+. The present work was undertaken to study the adsorption of Pb2+ on macroporous D152 resin in aqueous solutions in the form of batch and column processes. The effects of various adsorption conditions, such as pH, temperature and shaking speed were also investigated.
2 Experimental
2.1 Materials and instrument
Macroporous D152 resin was provided by Nankai University, China. The properties are shown in Table 1. Standard solution of Pb2+ was prepared by dissolving Pb(NO3)2·6H2O (analytical reagent grade) and concentrated nitric acid in purified water. The buffer solution with pH 3.50-6.50 was prepared from HAc-NaAc solution. Other chemicals used were of analytical reagent grade. Pb2+ was determined with Shimadzu UV-2550 UV-VIS spectrophotometer. Mettler toledo delta 320 pH meter was used for measuring pH. The sample was shaken with a DSHZ-300A temperature constant shaking machine.
Table 1 General description and properties of absorbent
2.2 Experimental method
2.2.1 Batch adsorption experiment
A desired amount of treated resin was weighed and added into a conical flask, in which a known volume of buffer solution with pH 5.4 was added. After 24 h, a required amount of standard solution of Pb2+ was added. The flask was shaken in a DSHZ-300A temperature constant shaking machine at the constant temperature. Aliquot samples were taken from the flask at appropriate time intervals for analysis until adsorption equilibrium reached. The adsorption capacity Q and distribution coefficient D were calculated with the following formulas, respectively:
Q=(C0-Ce)V/m (1)
D=Q/Ce (2)
where C0 and Ce are the initial and equilibrium concentrations of Pb2+ in solution, mg/mL; V is the total volume of solution, mL; and m is the mass of the resin, g.
2.2.2 Measurement of Pb2+
The concentration of unadsorbed Pb2+ in the sorption medium was determined by spectrophotometry. A solution containing Pb2+ less than 100 μg was accurately added into a 25 mL colorimetric tube, and then 2 mL of 0.1% xylenol orange solution and 10 mL methenamine-HNO3 buffer solution (pH 5.4) were added. After the addition of redistilled water to the mark of colorimetric tube, the absorbency was determined in a vessel (1 cm) on a Shimadzu UV-2550 UV-VISIBLE spectrophotometer at the wavelength of 560 nm and was compared with that of the blank test.
2.2.3 Elution test
A desired amount of resin was added into a mixed solution composed of buffer solution (pH 6.00) and a known amount of standard solution of Pb2+. After the equilibrium reached, the concentration of Pb2+ in the aqueous phase was determined, and then the adsorption capacity of the resin for Pb2+ was obtained. The resin separated from aqueous phase was washed three times with buffer solution (pH 6.00). The resin that adsorbed Pb2+ was shaken with eluant. After reaching equilibrium, the concentration of Pb2+ in aqueous phase was determined and then the percentage of elution was obtained.
3 Results and discussion
3.1 Influence of pH on distribution coefficient (D)
The pH of the aqueous solution is an important parameter that controls the sorption process. The adsorption behavior of Pb2+ on the D152 resin in HAc-NaAc medium was investigated in the pH range of 3.50-6.50. The results are shown in Fig.1, indicating that the distribution coefficient is the highest at pH 6.00 and is decreased by either raising or lowering pH [16]. Therefore, the following experiments were performed at pH 6.00 in the HAc-NaAc system.
Fig.1 Influence of pH on distribution coefficient of Pb2+ adsorption by D152 resin (resin 15.0 mg, T=298 K, C0=0.333 mg/mL, r=100 r/min)
3.2 Isotherm adsorption curve
The Langmuir model is perhaps the best known isotherm to describe adsorption from a liquid solution [17]. The model assumes uniform energies of adsorption on the surface and no interaction between adsorbed molecules. The model also assumes that adsorption is limited to complete surface coverage by a monomolecular layer and can be represented by the following equation:
Q=Q0bCe/(1+bCe) (3)
where Ce is the equilibrium concentration of metal ion, Q is the adsorbing capacity in equilibrium state, Q0 is the saturated sorbing capacity, and b is the Langmuir constant. It is usually rewritten in the following form:
Ce/Q=Ce/Q0+1/(Q0b) (4)
It is equally used to analyze batch equilibrium data by plotting Ce/Q vs Ce, which yields a linear plot if the data conform to the Langmuir isotherm. Five parts of 15.0 mg resin were added into a mixed solution composed of buffer solution (pH 6.00) individually. After 24 h, standard solutions of Pb2+ at initial concentrations of 0.267, 0.300, 0.333, 0.366 and 0.400 mg/mL were added respectively. When the adsorption equilibrium arrived, the experimental data were recorded.
Plotting of Ce/Q vs Ce gives a straight line. The correlation coefficient of straight line R2=0.997 6 was achieved. The values of Q0 and b determined from the figure are found to be 527 mg/g and 379.5 mL/mg, respectively. The results shown in Fig.2 indicate that the Langmuir-type adsorption isotherm is suitable for equilibrium studies.
Fig.2 Langmuir plot for adsorption of Pb2+ by D152 resin (resin 15.0 mg, r=100 r/min, pH 6.00)
A comparison of the maximum capacity of D152 resin with that of some other adsorbents reported in literatures is given in Table 2. Differences of metal uptake are due to the properties of each adsorbent such as structure, functional groups and surface area.
3.3 Determination of adsorption rate constant at different temperatures and apparent activation energies
The experiments were carried out by the above-mentioned method at 288, 298 and 308 K, respectively, and a series of data about the adsorption amounts and time were obtained (Fig.3). According to Ref.[26], the adsorption rate constant k can be calculated from the following two equations, respectively:
-ln(1-F)=kt (5)
F=Qt/Q∞ (6)
where Qt and Q∞ are the adsorption amounts at time t and at equilibrium state, respectively. A straight line was
Table 2 Comparison of the maximum adsorption capacity capacities of Pb2+ by various adsorbents
Fig.3 Adsorption rate curves of Pb2+ adsorption by D152 resin (C0(Pb2+)=0.333 mg/mL, resin 30.0 mg, pH 6.00)
obtained by plotting -ln(1-F) vs t (Fig.4). The adsorption rate constant of D152 resin for Pb2+ can be found from the slope of the straight line, which are k288 K=2.22×10-5 s-1, k298 K=2.51×10-5 s-1, and k308 K= 2.95×10-5 s-1, respectively. The linear relationship of -ln(1-F)—t indicates that the liquid film diffusion is the predominating step of the adsorption process.
According to the Arrhenius formula:
lgk=-Ea/(2.30RT)+lg A (7)
the slope of straight line, which was attained by plotting lg k vs 1/T (Fig.5) and calculated by linear fitting, yields the apparent activation energy of Ea=10.5 kJ/mol. It can
Fig.4 Determination of adsorption rate constant of Pb2+ adsorption by D152 resin (C0(Pb2+)=0.333 mg/mL, resin 30.0 mg, pH 6.00)
Fig.5 Linear plot of lg k vs 1/T for adsorption of Pb2+ by D152 resin (C0(Pb2+)=0.333 mg/mL, resin 30.0 mg, pH 6.00)
be seen from the rate constant that the adsorption speed accelerates when the temperature increases within the scope of experimental temperature.
3.4 Influence of adsorption temperature on distribution coefficient and determination of thermodynamic parameters
Under the experimental condition shown in Fig.6, the distribution coefficient of the resin for Pb2+ at the temperature ranging from 288 to 308 K was measured. A straight line was obtained by plotting lg D against 1/T with a correlation coefficient of 0.996 0. The result obviously indicates that it is favorable for the adsorption with the adsorption temperature going up. This implies that the adsorption process is an endothermic process. So the adsorption reaction is a chemical adsorption. According to the following equation:
lg D=-?HΘ/(2.30RT)+?SΘ/(2.30R) (8)
?H=13.3 kJ/mol can be computed from the slope of the line, and then ?S=119 J/(mol·K) can be obtained from the intercept of the line. According to
?GΘ=?HΘ-T?SΘ (9)
?GΘ288 K=-21.0 kJ/mo1, ?GΘ298 K=-22.2 kJ/mo1, ?GΘ308 K= -23.3 kJ/mo1 are obtained. These reveal that the adsorption reaction is a spontaneous one under the experimental condition [27].
Fig.6 Influence of temperature on distribution coefficient of Pb2+ adsorption by D152 resin (C0(Pb2+)=0.333 mg/mL, resin 15.0 mg, pH 6.00)
3.5 Effect of shaking speed on adsorption rate
The adsorption rate curves at different shaking speeds were studied in the range from 50 to 150 r/min. Three portions of 30.0 mg of resin were weighed accurately and put into conical flasks individually. The experiment was carried out by using the above mentioned method. The data are shown in Fig.7. The result shows that it is advantageous for the adsorption rate when the shaking speed rises, but the influence of shaking speed on adsorption capacity is not very obvious.
Fig.7 Influence of shaking speed on Pb2+ adsorption rate by D152 resin (C0(Pb2+)=0.333 mg/mL, resin 30.0 mg, pH 6.00, T=298 K)
3.6 Elution
The elution test was carried out according to the above mentioned method. 30.0 mL eluant was added. The results listed in Table 3 show the elution percentages are different when the concentration of HCl is changed and 0.05 mol/L HCl is the best.
Table 3 Results of elution test of Pb2+ (resin 15.0 mg, pH 6.00, T=298 K)
3.7 Dynamic adsorption and desorption
3.7.1 Dynamic adsorption curve
Dynamic adsorption was conducted using a glass column packed with the newly prepared D152 resin at room temperature [28]. 300 mg of resin was accurately weighed. After the column was conditioned, a solution with a concentration of 0.200 mg/mL Pb2+ was continuously fed into the column at a constant flow rate of 0.25 mL/min. The effluents from the column were analyzed quantitatively according to the above mentioned method. Breakthrough data were acquired by plotting the volume of solution that was passed through the column vs the concentration of Pb2+ in the effluent. When the concentration of Pb2+ emerging from the bottom of the column (C1, mg/mL) is equal to that entering the top of the resin bed (C2, mg/mL), the experiment was terminated. Then a plot of C1/C2 vs the volume of effluent gives a typical breakthrough curve in Fig.8.
Fig.8 Dynamic adsorption curve for Pb2+ adsorption by D152 resin at C2=0.200 mg/mL and flow rate of 0.25 mL/min
3.7.2 Dynamic desorption curve
The dynamic desorption curve of D152 resin for Pb2+ was obtained based on the volume of desorption solution and the Pb2+ concentration in the desorption solution (Fig.8). With respect to the stripping of Pb2+ from D152 resin, 0.05 mol/L HCl was employed and a flow rate of 0.15 mL/min for elution of Pb2+ from the column was used. The results are shown in Fig.9.
Fig.9 Dynamic desorption curve for Pb2+ adsorption by D152 resin at flow rate of 0.15 mL/min
4 Conclusions
(1) A novel macroporous weak acid resin (D152) is employed as a new adsorbent material for uptaking of Pb2+ in aqueous solutions. The adsorption characteristics are examined at different pH values, temperatures, shaking speeds and initial metal ion concentrations, in the form of batch and column processes.
(2) The maximum adsorption capacity of D152 resin for Pb2+ is evaluated to be 527 mg/g at 298 K in the HAc-NaAc system. And it is found that 0.05 mol/L HCl solution provides effectiveness of the desorption of Pb2+ from D152 resin.
(3) Isotherm studies show that the adsorption process of D152 resin for Pb2+ obeys the Langmuir model. This suggests that the adsorption of Pb2+ by D152 resin is monolayer-type and agrees with the observation that the metal ion adsorption from an aqueous solution usually forms a layer on the adsorbent surface.
(4) Thermodynamic parameters including standard enthalpy (?HΘ), standard entropy (?SΘ) and standard free energy (?GΘ) indicate that the adsorption of Pb2+ on D152 resin is a spontaneous reaction and is endothermic in nature.
(5) Breakthrough curves are obtained from column experiments. The experimental results demonstrate that D152 resin can be used for the removal of Pb2+ from aqueous solution.
References
[1] PAULINO A T, GUILHERME M R, REIS A V, TAMBOURGI E B, NOZAKI J, MUNIZ E C. Capacity of adsorption of Pb2+ and Ni2+ from aqueous solutions by chitosan produced from silkworm chrysalides in different degrees of deacetylation [J]. Journal of Hazardous Materials, 2007, 147(1/2): 139-147.
[2] MAUCHAUFFEE S, MEUX E. Use of sodium decanoate for selective precipitation of metals contained in industrial wastewater [J]. Chemosphere, 2007, 69(5): 763-768.
[3] GALAN B, CASTANEDA D, ORTIZ I. Integration of ion exchange and non-dispersive solvent extraction processes for the separation and concentration of Cr(VI) from ground waters [J]. Journal of Hazardous Materials, 2008, 152(2): 795-804.
[4] MELITA L, POPESCU M. Removal of Cr(VI) from industrial water effluents and surface water using activated composite membranes [J]. Journal of Membrane Science, 2008, 312(1/2): 157-162.
[5] BASHA C A, BHADRINARAYANA N S, ANANTHARAMAN N, MEERA SHERIFFA BEGUM K M. Heavy metal removal from copper smelting effluent using electrochemical cylindrical flow reactor [J]. Journal of Hazardous Materials, 2008, 152(1): 71-78.
[6] GAN Tian, WU Kang-bing. Sorption of Pb(II) using hydrogen peroxide functionalized activated carbon [J]. Colloids and Surfaces A: Physicochemical and Engineering Aspects, 2008, 330(2/3): 91-95.
[7] RANGEL-MENDEZ J R, MONROY-ZEPEDA R, LEYVA-RAMOS E, DIAZ-FLORES P E, SHIRAI K. Chitosan selectivity for removing cadmium (II), copper (II), and lead (II) from aqueous phase: pH and organic matter effect [J]. Journal of Hazardous Materials, 2009, 162(1): 503-511.
[8] CHAARI I, FAKHFAKH E, CHAKROUN S, BOUIID J, BOUJELBEN N, FEKI M, ROCHA F, JAMOUSSI F. Lead removal from aqueous solutions by a tunisian smectitic clay [J]. Journal of Hazardous Materials, 2008, 156(1/3): 545-551.
[9] RAJESH N, JALAN R K, HOTWANY P. Solid phase extraction of chromium(VI) from aqueous solutions by adsorption of its diphenylcarbazide complex on an Amberlite XAD-4 resin column [J]. Journal of Hazardous Materials, 2008, 150(3): 723-727.
[10] XIONG Chun-hua, YAO Cai-ping. Sorption behavior of weak acid resin (D113) for zinc [J]. The Chinese Journal of Nonferrous Metals, 2008, 18(4): 745-749. (in Chinese)
[11] XIONG Chun-hua, YAO Cai-ping, WANG Yong-jiang. Sorption behaviour and mechanism of ytterbium(Ⅲ) on imino-diacetic acid resin [J]. Hydrometallurgy, 2006, 82(3/4): 190-194.
[12] JI Chun-nuan, QU Rong-jun, WANG Chun-hua, CHEN Hou, SUN Chang-mei, XU Qiang, SUN Yan-zhi, WEI Chao. A chelating resin with bis[2-(2-benzothiazolylthioethyl) sulfoxide]: Synthesis, characterization and properties for the removal of trace heavy metal ion in water samples [J]. Talanta, 2007, 73(2): 195-201.
[13] DONIA A M, ATIA A A, HENIESH A M. Efficient removal of Hg(II) using magnetic chelating resin derived from copolymerization of bisthiourea/thiourea/glutaraldehyde [J]. Separation and Purification Technology, 2008, 60(1): 46-53.
[14] XIONG Chun-hua, YAO Cai-ping, WU Xiang-mei. Adsorption of rhenium(Ⅶ) on 4-amino-1, 2, 4-triazole resin [J]. Hydrometallurgy, 2008, 90(2/4): 221-226.
[15] SHU Zeng-nian, XIONG Chun-hua, WANG Xu. Adsorption behavior and mechanism of amino methylene phosphonic acid resin for Ag(I) [J]. Transactions of Nonferrous Metals Society of China, 2006, 16(3): 700-704.
[16] GODE F, PEHLIVAN E. Removal of chromium(III) from aqueous solutions using Lewatit S 100: The effect of pH, time, metal concentration and temperature [J]. Journal of Hazardous Materials, 2006, 136(2): 330-337.
[17] CHEN Yong-gui, ZHANG Ke-neng, ZOU Yin-sheng, DENG Fei-yue. Removal of Pb2+ and Cd2+ by adsorption on clay-solidified grouting curtain for waste landfills [J]. Journal of Central South University of Technology, 2006, 13(2): 166-170.
[18] GUPTA V K, GUPTA M, SHARMA S. Process development for the removal of lead and chromium from aqueous solutions using red mud—An aluminium industry waste [J]. Water Research, 2001, 35(5): 1125-1134.
[19] COLEMAN N J, LEE W E, SLIPPER I J. Interactions of aqueous Cu2+, Zn2+ and Pb2+ ions with crushed concrete fines [J]. Journal of Hazardous Materials, 2005, 121 (1/3): 203-213.
[20] HO Y S, MCKAY G. The sorption of lead(II) ions on peat [J]. Water Research, 1999, 33(2): 578-584.
[21] ISSABAYEYA G, AROUA M K, SULAIMAN N M N. Removal of lead from aqueous solutions on palm shell activated carbon [J]. Bioresource Technology, 2006, 97(18): 2350-2355.
[22] ABDEL-HALIM S H, SHEHATA A M A, El-SHAHAT M F. Removal of lead ions from industrial waste water by different types of natural materials [J]. Water Research, 2003, 37(7): 1678-1683.
[23] MEMON S Q, HASANY S M, BHANGER M I, KHUHAWAR M Y. Enrichment of Pb(II) ions using phthalic acid functionalized XAD-16 resin as a sorbent [J]. Journal of Colloid and Interface Science, 2005, 291(1): 84-91.
[24] YURTSEVER M, AYHAN SENGIL I. Biosorption of Pb(II) ions by modified quebracho tannin resin [J]. Journal of Hazardous Materials, 2009, 163(1): 58-64.
[25] XIONG Chun-hua, YAO Cai-ping. Adsorption behavior of gel-type weak acid resin (110-H) for Pb2+ [J]. Transactions of Nonferrous Metals Society of China, 2008, 18(5): 1290-1294.
[26] ZHANG Shu-qin, HOU Wan-guo. Adsorption behavior of Pb2+ on montmorillonite [J]. Colloids and Surfaces A: Physicochemical and Engineering Aspects, 2008, 320(1/3): 92-97.
[27] El-SOFANY E A. Removal of lanthanum and gadolinium from nitrate medium using Aliquat-336 impregnated onto Amberlite XAD-4 [J]. Journal of Hazardous Materials, 2008, 153(3): 948-954.
[28] WEI Jun-fu, WANG Zhi-peng, ZHANG Jing, WU Yue-ying, ZHANG Zheng-pu, XIONG Chun-hua. The preparation and the application of grafted polytetrafluoroethylene fiber as a cation exchanger for adsorption of heavy metals [J]. Reactive and Functional polymers, 2005, 65(1/2): 127-134.
(Edited by CHEN Wei-ping)
Foundation item: Project(2008F70059) supported by the Scientific and Technological Research Planning of Zhejiang Province, China
Received date: 2008-11-03; Accepted date: 2009-01-06
Corresponding author: XIONG Chun-hua, Professor; Tel: +86-571-88932083; E-mail: xiongch@163.com
- Adsorption of Pb2+ on macroporous weak acid adsorbent resin from aqueous solutions: Batch and column studies
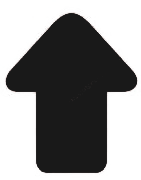