- Abstract:
- 1 Introduction▲
- 2 Coating structure ...▲
- 3 Antioxidant coatin...▲
- 4 Antioxidant mechan...▲
- 5 Coating techniques▲
- 6 Summary▲
- References
- Figure
- Figure 1 Structure of anti-oxidation resistance coating on surface of matrix graphite
- Figure 2 Structure schematic of SiC-coated sphere after two-step PC [28] (a) and dual layer SiC-coated sphere [29] (b)
- Figure 3 SEM images of SiC coatings after oxidation for 3 h at 1173 K:
- Figure 4 Oxidation model of single SiC coating for carbon materials
- Figure 5 Oxygen model of carbides coating for C/C composites
- Figure 6 Oxidation model of mullite/SiC coating for C/C composites
- Figure 7 Schematic illustrations of oxidation process of coating:
J. Cent. South Univ. (2019) 26: 2915-2929
DOI: https://doi.org/10.1007/s11771-019-4224-2
Review of oxidant resistant coating on graphite substrate of HTR fuel element
YANG Hui(杨辉), ZHAO Hong-sheng(赵宏生), LI Zi-qiang(李自强), LIU Xiao-xue(刘小雪),
ZHANG Kai-hong(张凯红), WANG Tao-wei(王桃葳), LIU Bing(刘兵)
Institute of Nuclear and New Energy Technology of Tsinghua University, Collaborative Innovation Center of Advanced Nuclear Energy Technology, Beijing 100084, China
Central South University Press and Springer-Verlag GmbH Germany, part of Springer Nature 2019
Abstract:
The core components of HTR mainly consist of graphite, which is an excellent solid moderating material and structure material with good irradiation performance. However, graphite is easier to be oxidized at high temperature. It is important to improve the anti-oxidation performance of graphite substrate to enhance the safety of HTR. Anti-oxidation coating has been proved that it can effectively improve the oxidation resistance of graphite substrate. The selection of coating includes the coating materials system and the preparation techniques. In addition, attention also should be paid to the anti-oxidation mechanism. SiC based coating system is most commonly used in HTR and some other coating systems such as zirconium compounds coating, noble metal coating also can be used in nuclear. However, some coating systems are not suitable in HTR because the elements contained cannot be used in high irradiation environment. The emphasis is on the antioxidant mechanism of different coating systems. Some anti-oxidation coating techniques for graphite substrate are also reviewed in this paper, such as PC, CVD, PS and slurry. Finally, the existing problems of the oxidation resistance coating are proposed and an outlook is made for the development of the protection coating system for graphite substrate used in nuclear.
Key words:
graphite; coating; nuclear; oxidation; mechanism;
Cite this article as:
YANG Hui, ZHAO Hong-sheng, LI Zi-qiang, LIU Xiao-xue, ZHANG Kai-hong, WANG Tao-wei, LIU Bing. Review of oxidant resistant coating on graphite substrate of HTR fuel element [J]. Journal of Central South University, 2019, 26(11): 2915-2929.
DOI:https://dx.doi.org/https://doi.org/10.1007/s11771-019-4224-21 Introduction
Due to their excellent nuclear properties and high-temperature performance, such as higher scattering cross section, low thermal neutron absorption cross section, low thermal expansion coefficient, good thermal shock resistance, and outstanding thermal conductivity, graphite materials have been used as a moderating material and an important structure material for the fuel elements in high-temperature gas-cooled reactor (HTR) [1-3]. But in the oxidizing atmosphere above 773 K, graphite is prone to oxidation to make its performance drop. In the event of a leakage of water or gas in a road in HTR, the partial pressure of the oxidizing gas inside the reactor gradually increases. As a result, the graphite component and matrix graphite of fuel element would be oxidized. The release of fission products from the reactor is increased. Thus, the safety of the reactor is decreased. Spherical fuel elements with 60 mm in diameter are composed of a fuel zone with a diameter of 50 mm and a fuel-free shell with a thickness of 5 mm. So, the antioxidant properties of graphite bulk material are very important to improve the safety of HTR. In addition, carbon materials are an important conductive material and structural material in metallurgy, chemical industry, electric power, electronics, aerospace, machinery and other industrial sectors. But the poor oxidation resistance limits their use in oxidizing atmosphere. The research results of improving the antioxidant properties of carbon materials also have important value for the application of graphite in these fields [4, 5].
Besides, the antioxidant research of carbon fiber reinforced carbon (C/C) composites is the most extensive in all the carbon materials. Due to the similarity of its structural properties, the antioxidation research of C/C composites is often used for reference to the oxidation process of graphite substrate. There are two main methods to protect C/C composites from oxidation: surface modification and surface coating technology. The surface modification technology is to change the components of the matrix during the preparation of the material, so that the matrix has a strong antioxidant capacity. The commonly used methods include impregnation method, additive method and matrix replacement method [6-10]. Although the modifier used in this technique has the similar properties to the matrix, the composition of the matrix is changed, so the physical and chemical properties of the matrix have been changed and the application of the matrix needs to be retested. Due to the fact that the final step in the preparation of nuclear fuel elements is purification at 2173 K to remove the impurities introduced in the manufacture of components, the low content modifier would be consumed at this step. Therefore, surface modification technology is not suitable for the improvement of graphite oxidation resistance in HTR.
The basic principle of surface coating technology is using the coating as a barrier to prevent the contact of oxygen with the substrate, to eliminate the reactant of the chemical reaction and achieve high temperature oxidation resistance. Since the coating material does not enter the substrate interior, the properties of substrate can be retained to a maximum extent, and the applicability of the substrate will not change. Due to the fact that the coating is very compact generally, it is very hard for the oxidation medium diffusion through the coating to contact the substrate. Therefore, its antioxidant ability is relatively more effective and thorough. It is the main method to improve the antioxidation performance of graphite substrate in HTR. According to the mechanism of oxidation resistance, the study of anti-oxidation coating mainly focuses on the coating preparation techniques and the selection of coating materials systems.
In this paper, different anti-oxidation coating materials systems are reviewed and the anti-oxidation mechanism of different coating materials systems are also analyzed. Finally, the coating technique is introduced.
2 Coating structure design
In the application of high-temperature anti- oxidation coating, the application conditions, the interaction between the coating and the substrate and the components in the coating should be considered. So, high-temperature anti-oxidation coating should meet the following requirements:
1) The elements in the coating must meet the requirements of the working conditions. For example, some elements are not suitable for use in the nuclear environment, such as B, P, LI [11, 12].
2) The coating material itself and its oxidation products have low-oxygen permeability and good high-temperature stability in the case that the excessive loss of the coating causes the substrate to expose directly to the oxidation environment.
3) The coating is compact and has little defects.
4) The coating has certain fluidity at high temperature in order to seal the defects in the coating.
5) Good coefficient of thermal expansion (CTE) matching and bonding ability should exist between the coating and the substrate, so the coating is not easy to peel off.
A single coating can’t meet the requirements of oxidation resistance [13, 14]. Composite coating with multilayer structure is an important development direction of the coating technology. According to the effect of different coating structures, it can be divided into four types as shown in Figure 1.
1) Transition layer. The function of transition layer is to minimize the CTE mismatch between the coating and the substrate and alleviate the stress in the thermal shock cycle. The most common used material is C/SiC coating [15-17]. The gradient distribution of Si and C elements in the coating can inhibit the generation of thermal stress, so that the coating has excellent oxidation resistance.
Figure 1 Structure of anti-oxidation resistance coating on surface of matrix graphite
2) Barrier layer. The function of barrier layer is to slow oxygen penetration. The most common used materials are SiO2, Al2O3, etc. [18-20], which have lower oxygen diffusion coefficient.
3) Sealing layer. Under the thermal expansion stress of the coating and the substrate, cracks are inevitable in the coating. The function of the sealing layer is to seal the cracks at elevated temperature to maintain the integrity of the coating. Due to the good fluidity of glass, glass coating is often used as the sealing layer in multilayer structure [21-23].
4) Functional layer. According to the use of graphite substrate, a coating is prepared on the outer surface to meet specific application conditions. For example, some C/C composite materials are used in the field of aerospace, facing the problem of high- temperature oxygen ablation, and a layer of corrosion resistant layer is needed [24-26].
In conclusion, the single material system cannot meet the needs of coating antioxidant. In practice, different coating material systems and coating technologies were utilized to fabricat a good oxidation resistance coating. MORIMOTO et al [27] designed a multilayer coating consisting of a SiC inner layer and a refractory oxide outer layer. The inner SiC layer functions as a transition layer to adhere tightly to C/C composites and release thermal stress caused by the mismatch of CTE between C/C composites and the oxide outer layer. The outer layer can protect C/C composites from oxidation and erosion. The results show that the multilayer coating can obtain better oxidation resistance [27]. ZHOU et al [28] used two-step pack cementation (PC) technique to prepare a micro four-layer SiC coating on the graphite substrate spheres. The structure of the coating shown in Figure 2(a) is similar to the TRISO coated particles. The main difference in different coatings is the SiC grain size and microstructure. After oxidation at 1773 K for 200 h, the weight gain of the coated sample is only 0.52 wt%, and the fine-grained and dense bulk layers are as the functional layers. A gradient region with a fiber-woven structure as the inner transition layer is beneficial to the release of the stress during the thermal shock [28]. They also used PC and fluidized-bed chemical vapor deposition (FB-CVD) process to prepare a dual-layer SiC coating including inner porous SiC layer and outer dense SiC layer on graphite substrate used in HTR [29]. The structure is shown in Figure 2(b). The basic design concept for this study is that the outer p-SiC layer is as the main functional layer to protect matrix graphite from oxidation, and the inner d-SiC layer is as the transition layer to improve the bond strength between the substrate and outer p-SiC layer. In addition, the p-SiC also can release the stress occurring in the thermal shocking tests. The dual- layer SiC coating can effectively improve the oxidation resistant property of MG sphere at the temperature up to 1773 K for 200 h and could understand more than 50 thermal shocking cycles from 1773 K to room temperature with no stress cracking.
Figure 2 Structure schematic of SiC-coated sphere after two-step PC [28] (a) and dual layer SiC-coated sphere [29] (b)
3 Antioxidant coating material systems
3.1 Ceramic coatings
Ceramic coating is the most advanced anti-oxidation coating system. The key technique is to use the ceramic oxides generated at high temperature to fill the cracks in the coating. And the oxides also can be used as a sealing material to prevent the infiltration of oxygen. The most common used ceramic coating systems are Si-based and Zr-based coating systems.
3.1.1 SiC coating
Due to its outstanding oxidation resistance, low neutron absorption cross section, high thermal stability and good compatibility with C/C composites, SiC coating is widely used as a protective coating for graphite substrate in HTR. When the SiC coated C/C composites are placed in air at elevated temperature, the glassy SiO2 film will be formed on the surface of the coating with the typical oxidation surface micrographs shown in Figure 3, which provides efficient barrier to limit oxygen diffusing into the substrate [30]. SiC coating cannot be prepared by thermal spraying because it would be decomposed before melting. The most common preparation method is PC, CVD and slurry.
TANG et al [31] prepared a continuous and uniform SiC coating on the surface of graphite substrate through the reaction between graphite and molten silicon. There is a gradient region between coating and substrate, which not only enhances the adhesion of the SiC coating to substrate, but also releases the stress from the mismatch of CTE between the SiC coating and substrate. After oxidation at 1273 K for 8 h, the weight loss of the coated graphite is only 1.7 wt%. ZHU et al [32] prepared a SiC coating on the surface of graphite substrate. The coating can retain its integrity after oxidation over 1000 h at 1473 K with 100 thermal cycles and 200 h at 1673 K with 20 thermal cycles. The coating shows excellent oxidation resistance and thermal shock resistance, which is attributed to the composition gradient of the coating. FU et al [33] obtained a uniform dense SiC coating fabricated from polycarbosilane (PCS) on graphite substrate. The coating is composed of β-SiC and free carbon and there is a gradient transition layer at the interface between the coating and substrate. The thickness and the density of the SiC coating increased with the increase of PCS concentration in (10-30) wt%.
Figure 3 SEM images of SiC coatings after oxidation for 3 h at 1173 K:
However, the defects such as cracks and pores are inevitable during the preparation of a single SiC coating. In addition, SiO2 is easy to crystallize, so a lot of grain boundaries would be formed, which provide pathway for oxygen to attack the substrate. So, multilayer coating with a SiC coating as an inner layer is needed to protect carbon materials. FU et al [34] prepared a dual SiC coating on the surface of C/C composites, including an inner β-SiC layer and an outer coating consisting of α-SiC, Si and β-SiC by two-step PC. The results show that the coating exhibits excellent anti- oxidation resistance. After more than 310 h oxidation at 1773 K in air, the mass loss rate of the coated sample is only 0.9×10-5 g/(cm2·h), which is caused by the formation of holes in the coating and the peeling off of the glass phase [34]. SUN et al [13] also prepared a double SiC coating to protect C/C composites from oxidation. The coating system can effectively block the entry of oxygen. After oxidation at 1773 K for 178 h, the weight loss of the coated sample is 1.25%. After 16 thermal shock cycles from 1773 K to room temperature, the mass loss of the coated sample is 2.74%. The coating loses its protective effect due to the slow formation of sealing SiO2 film.
In addition, there are also some Si-based materials widely used as coating materials, such as MoSi2 and CrSi2. FU et al [35] used a two-step PC process to prepare a CrSi2-SiC coating for C/C composites. Filling liquid CrSi2 in the defects of SiC coating can greatly improve the antioxidant capacity of coated samples. The weight loss of the CrSi2-SiC coated C/C composites is only 4.15% after 34 h oxidation at 1773 K in air. ZHAO et al [36] prepared a SiC/Si-MoSi2 coating on graphite substrate. The coating shows excellent anti-oxidation resistance and excellent shock resistance. The reason may be that during the preparation of the Si-MoSi2 coating, the inner SiC coating can effectively block the infiltration of liquid Si into the defects in the substrate. LI et al [37] prepared a MoSi2-CrSi2-Si coating on the surface of the SiC pre-coated C/C composites. The coating obtains an excellent anti-oxidation capability and the coated C/C composites lose only 0.8% of their weight after 500 h oxidation at 1873 K. The formed silica glass layer contributes to the excellent antioxidant capacity of the coating at elevated temperature. And the glass layer also can seal the cracks in the coating [36, 38]. The antioxidant mechanism of the silicide coating is that under high temperature oxygen environment, the antioxidant capacity can be improved by the formation of other low-oxygen permeability oxide to make up for the deficiency of single SiO2 glass layer. These oxides can combine with SiO2 to seal the defects in the coating, so that the coating keeps its integrity. Thus, the antioxidation of the silicate coating can be improved compared with the single SiC coating.
3.1.2 Zirconium compounds coating
The neutron absorption cross section of zirconium alloys is only (0.2-0.24)×10-28 m2, which is similar to graphite substrate. In addition, ZrO2 can be generated through the oxidation of zirconium compounds, which would react with SiO2 to product ZrSiO4. HENNIGE et al [39] found that ZrO2 can react with SiO2 to generate ZrSiO4 start at 1373 K, and completely react at 1873 K. In addition, ZrSiO4 has a low CTE (αZrSiO4≈4.9×10-6 K-1 [40]) and high stability in oxidation and corrosion environment [41, 42]. So, zirconium compounds are one of the candidate coating materials used in nuclear reactor. POURASAD et al [43] prepared a nanostructure SiC/SiC-ZrO2 coating using two-step PC technique on the surface of graphite substrate. The formation of the SiC nanofibers, SiO2 glass layer and the zirconium silicate phase in the coating enhance the antioxidant capacity of the coating.
Due to the feature of a low density, high hardness, high strength and high modulus, ZrC is most commonly used zirconium compound. DONG et al [44] prepared a SiC-ZrC ceramic coating on C/C composites using reactive melting infiltration technique. The prepared coating shows better anti-oxidation resistance than the single SiC coating. The rapid formation of a continuous glass-like layer including ZrO2, ZrSiO4 and SiO2 covers the surface of the coating and inhibits the diffusion of oxygen to C/C composites. SUN et al [26] prepared a ZrC coating on C/C composites by CVD. The coating has an excellent anti-oxidation ability for C/C composites. After ablation for 240 s in an oxy-acetylene torch system, the mass ablation rate and the linear ablation rate of the coated sample are 1.1×10-4 g/cm2 s and 0.3×10-3 mm/s, respectively. After oxidation, a dense ZrO2 layer is formed on the surface of the coating, which can reduce the diffusion of oxygen to substrate. Compared to the conventional ZrC coating, WANG et al [45] found that the ZrC coating with a nanostructure can improve the anti-ablation resistance. And the linear and mass ablation rates of the sample are improved by 50.7% and 42.1%, respectively. The improvement of the performance of the coating is mainly contributed to the formed ZrO2 layer consisting of nanorods, presenting better ablation resistance, which is dense and crack-free. Next, they also studied the oxidation of ZrC coating under oxyacetylene torch environment [46]. During the oxidation process, ZrC is oxidized into ZrCxOy firstly. As the oxygen continues to spread, ZrCxOy is oxidized to ZrO2.
Besides, there are also some zirconium compounds coatings showing excellent anti- oxidation resistance [47, 48]. YAO et al [49] prepared a SiC-Si-ZrB2 multiphase coating on the surface of SiC-coated C/C composite by the process of PC. The coating can protect C/C against oxidation at 1773 K for more than 386 h. WANG et al [50] prepared a ZrB2-SiC coating on the SiC coated graphite materials by PC technique. Due to a dense glass layer formed after oxidation, the coating can withstand thermal shock cycles between 1773 K and room temperature the fact that 15 times, with a weight loss of 20.2%. But due to B is not suitable used in nuclear, these coatings cannot be used in the protection of graphite substrate.
3.1.3 Mullite coating
Due to its high melting point, low thermal conductivity, stability and lower oxygen permeability at elevated temperatures, mullite coating (3Al2O3·2SiO2) is a promising candidate coating material for carbon materials. In addition, it also has a close CTE with SiC (αmullite≈5.3×10-6 K-1) [51-53], so it’s usually as an outer layer for the SiC coated carbon materials. When oxidation in dry air, a slowly growing SiO2 scale would be formed at the mullite-SiC interface [54]. Depending on the content of SiO2, a liquid phase can be formed from mullite coating. The liquid phase is very viscous, which can seal the cracks and pores in the coating.
YANG et al [53] prepared a double coating including inner β-SiC layer and an outer layer composed of SiO2 and mullite. The mass gain of the coated sample is only 0.085% after being exposed to air at 1423 K for 109 h and 12 thermal shock cycles between 1423 K and room temperature. The results show that the coating system has excellent oxidation resistance and can provide a long-term effective antioxidant protection for carbon materials [55]. WANG et al [55] prepared a mullite/SiC coating of C/C composites. The mass loss of the coated sample is only 4.89×10-4 g/(cm2·h) after oxidation at 1773 K for 322 h. After a long period of oxidation, the coating failed due to the evaporation of the silicate glass layer and some defects formed in the coating.
3.1.4 Glass coating
Due to its low viscosity, good wettability with the matrix and good thermal stability at high temperature, glass coating has good oxidation and self-seal properties. In addition, the glass coating can maintain its integrity under oxygen-containing environment at elevated temperature. Because the mismatch of the CTE, SiC is usually as an internal buffer layer and glass coating is as an external layer in a coating system for carbon materials. Up to now, borosilicate and phosphate are the most commonly used coating systems [56-58].
HUANG et al [59] prepared a borosilicate glass coating on the SiC pre-coated C/C composites. The escape of CO and CO2 and the volatilization of borosilicate glass layer cause many microcracks and oxidation holes in the coating, which causes the failure of the coating. FU et al [60] also prepared a borosilicate glass coating on SiC pre-coated C/C composites. After 140 h oxidation at 1773 K in air, the mass loss of the sample is only 0.98%, which may be due to the formation of oxidation holes and microcracks in the coating. They also prepared a outer layer of magnesium boron aluminosilicate glass with some molybdenum disilicide particles dispersed in it. The weight loss of the sample is only 1.07%, after oxidation for 150 h at 1573 K in air and 20 thermal shock cycles from 1573 K to room temperature. The oxidation of the sample was caused by the degumming of some glass layer on the surface of the coating [61]. A SiC-zirconia-glass coating was prepared by WANG et al [62] on the surface of C/C composites at the temperature of 1123-1273 K. During the sintering process, the SiO2-Al2O3-CaO-MgO coating gradually changed into amorphous substance. The cracks in the coating were the main pathway for oxygen to attack the substrate, and the oxygen diffusion rate through the coating was the main control factor for the oxidation. The mass loss rate of the sample increased nearly linearly with time as temperature rose.
3.2 Noble metal coating
Noble metal has a characteristic of high melting point. Under the conditions of high- temperature oxygen environment, the metal coating not only consumes oxygen but also forms oxidation product with very low oxygen permeability [18, 63-65]. Thus, noble metal coating would be a good choice for the protection of matrix graphite.
HUANG et al [66] used a two-step PC and slurry method to prepare a SiC/Cr-Al-Si coating on the surface of C/C composites. The study shows that the oxygen resistance depends on the thin thickness and porous structure of the mono-layer SiC coating. The coating with a dense surface can protect C/C composites with a mass gain rate only 0.93×10-6 g/(cm2·h) after oxygen at 1773 K for 197 h. The failure of the double coating is due to the excessive consumption of the outer Cr-Al-Si alloy coating and there are also some defects appearing in the SiO2 glass layer. SHI et al [67] prepared a La-Mo-Si-O-C coating on the surface of porous SiC pre-coated C/C composites. The results show that the coating can protect C/C composites for 85 h at 1773 K in air environment. After oxidation, the coating was crack free and a glass layer composed of La2O3, La2SiO5, SiO2 was formed on the surface of the coating, which can effectively inhabit the oxygen diffusing into the inner coating. LI et al [68] used PC technique to prepare a SiC/Si-W-Mo coating on the surface of C/C composites. The multi-coating was composed of α-SiC, Si and (WxMo1-x) Si2. The weight gain of the coated sample is only 0.02% after oxidation at 1773 K for 315 h in air and thermal shock cycles from 1773 K to room temperature. During the process of oxidation, a continuous and dense SiO2 glass layer was formed on the surface of the dense multi- coating surface, which makes the coating have excellent oxidation resistance.
4 Antioxidant mechanism
4.1 SiC coating
According to the different oxygen partial pressure, the oxidation behavior of SiC coating can be divided into two modes: the passive oxidation by the reaction of Eqs. (1) and (2), which would result in a mass increase, and active oxidation by the reaction of Eq. (3), which would result in a mass decrease.
SiC(s)+O2(g)→SiO2(s)+CO(g)↑ (1)
SiC(s)+O2(g)→SiO2(s)+CO2(g)↑ (2)
SiC(s)+O2(g)→SiO(g)↑+CO(g)↑ (3)
When postulated accidents occur, a primary coolant pipe is ruptured, which would cause air or water ingress into the reactor core. As a result, the coated graphite substrate would be exposed to air, so a passive oxidation of the SiC coating would occur. During the oxidation process, a dense SiO2 glass layer will formed on the surface of the SiC coating, which would be provide an effectively protection for substrate. The results also show that the silicon oxycarbides can be formed at the interface of SiO2/SiC in the sequence of SiO3C, SiO2C2, and SiOC3 [69]. The passive oxidation process of the single SiC coating protected C/C composites can be concluded just as shown in Figure 4. The anti-oxidation mechanism is as follows:
First, a glass layer mainly composed of SiO2 is formed on surface of the coating caused by the formation of amorphous silica. Due to the very low oxygen permeability, the glass layer can protect C/C composites from oxidation efficiently. Meanwhile, some gas is also generated and escapes from the glass layer. Due to the better viscosity of the SiO2, it can seal some defects in the original coating.
Figure 4 Oxidation model of single SiC coating for carbon materials
Second, due to the mismatch of CTE, some cracks will be generated at the interface of SiC coating and C/C composites. With the oxidation time increase, some cracks formed in the glass layer and the glass phase layer begins to vaporize. Combined all the factors, the coating will crack and create a diffusing channel for oxygen to access C/C composites (Eqs. (4) and (5)). Thus, the coating loses its protection for C/C composites.
C(s)+O2(g)→CO2 (g)↑ (4)
C(s)+O2(g)→CO(g)↑ (5)
The active oxidation of SiC would occur in the conditions of low partial pressure of oxidative gas in helium with little impurities and SiC would be oxidized into gaseous SiO and CO, which would result in a notable mass loss of the samples [70]. As a result, SiC coating would be consumed gradually with the time increase. FU et al [71] calculated the critical temperature of the passive oxidation of SiC and the decomposition of SiO2 in He-O2 using HSC chemical reaction and equilibrium software. The results show that the multilayer SiC/SiO2 coating is more effectively than single SiC coating to improve the oxidation resistance of graphite in He-O2 system with a low partial pressure. FU et al [72] also prepared a SiO2/SiC coating on the surface of graphite substrate. The results show that the SiO2/SiC coating can protect graphite substrate from oxidation both in air and in argon with little oxygen conditions. The coating can still keep stability and provide great protection for graphite substrate from oxidation, after oxidation at 1273 K for 100 h in high purity helium. In addition, the coating also has an excellent thermal shock property.
4.2 Zirconium compounds coating
According to the previous works [26, 46, 73], the oxidation process of the carbides coating for carbon materials can be divided into four stages. Taking ZrC coating as an example, the oxidation model of the ZrC coated sample is shown in Figure 5.
ZrC(s)+O2(g)→ZrCxOy (s)+CO(g)↑ (6)
ZrCxOy(s)+O2(g)→ZrO2(s)+CO(g)↑ (7)
The ZrC is oxidized into ZrCxOy firstly according to Eq. (6). The dense ZrCxOy layer could block the diffusion of oxygen to C/C composites because of its low oxygen permeability. The oxidation is controlled by the reaction rate between ZrC and oxygen.
With the steady diffusion of oxygen, the ZrCxOy is oxidized into ZrO2 (Eq. (7)) [74]. The melted ZrO2 layer spreads on the surface of the coating and the defects in the coating can be sealed in time. Due to the fact that ZrO2 has high melting point and the good viscosity of the molten ZrO2, the oxygen resistance of the coating can be improved sufficiently. At the same time, the oxidation reaction produces a large number of CO gases, which will escape through the molten ZrO2 layer. The oxidation is determined by the diffusion rate of oxygen through the ZrO2 layer and ZrCxOy layer.
As the oxidation process continues and the glass vaporizes, part of the ZrO2 layer is consumed. Meanwhile, the pores and cracks in the coating become more and more, even some longitudinal cracks occur. So, oxygen can through these channels to attack C/C composites. With the reactions Eq. (4) and Eq. (5) occur, C/C composites are oxidized. And the ZrO2 and ZrCxOy layers lose their protection for C/C composites from oxygen. The oxidation is determined by the diffusion rate of oxygen through the defects in the coating.
Figure 5 Oxygen model of carbides coating for C/C composites
4.3 Mullite coating
The oxidation model of the mullite/SiC coating can be divided into five stages according to the previous works [50, 71], as shown in Figure 6.
The oxidation is started with the oxygen diffusion through the defects in coating to the interface of mullite and SiC in the first stage. According to Eqs. (1) and (2), the inner SiC is oxidized and some SiO2 glass is generated. The oxidation is determined by the diffusion of oxygen through defects in the mullite coating [52].
With the continuation of oxygen diffusion, the SiO2 glass layer will be generated and as a buffering layer to seal the defects in the mullite layer and SiC layer. Due to the fact that SiO2 has low oxygen permeation rate, the coating at this stage has a good oxygen resistance.
Then the mullite layer can be gradually transformed into a dense and smooth silicate glass layer by the reaction of mullite and SiO2 (Eq. (8)). Meanwhile, Al2O3 will be formed through the decomposition of the silicates glass (Eq.(9)).
SiO2(s)+ Al2O3·2SiO2(s)→silicates glass(m) (8)
silicates glass(m)→Al2O3+SiO2(s) (9)
Next the mullite layer begins to decompose and a thicker silicates glass will be formed. The oxidation of the coated sample is determined by the oxygen diffusion rate through the silicates layer. Owning to the low oxygen permeability and good self-healing ability, silicates coating provides an optimum protection for the carbon materials.
With the time increase, the evaporation of the glass layer causes its thickness to decrease gradually. Oxygen can diffuse through the residual glass layers to attack C/C composites (Eq. (4) and Eq. (5)). In addition, micro-holes and micro-cracks will be formed due to the escape of the oxidation product CO and CO2, which provide pathway for oxygen to further diffuse to C/C composites. Thus, the coating loses its protection for carbon materials and the substrate will be oxidized.
4.4 Noble metal coating
The antioxidant mechanism of the noble metal coating can be refereed to Figure 7. Under high temperature oxidation environment, metal can consume oxygen to avoid the attack to substrate. Although the oxidation of metal can cause some defects to the coating, the oxidation product of noble metal usually has good liquidity, which can combine with SiO2 to seal the defects in the coating [65]. Thus, the coating can keep its density and integrity. In order to obtain coatings having excellent antioxidation, the metal which can be oxidized to oxides with low oxygen diffusion rate is the preferential selection. The failure of the metal coating is that due to the excessive oxidation of the metal, the oxidation product cannot quickly seal the defect. The oxygen has channels to access C/C composites, and the coating loses its protection.
4.5 Glass coating
The glass coating has the characteristics of low mobility and low rate for the permeability of oxygen, which can effectively fill the defects of the coating. In addition, the outer glass coating can inhabit the volatilization of the inner SiC coating during oxidation process. The main problem of glass coating is that it is very easy to volatile at high temperature. At high temperatures, the glass is too viscous to flow, so the cracks cannot be sealed in time. As a result, oxygen can diffuse through these microcracks to attack C/C composites. In addition, since self-healing requires a certain amount of time and the thermal shock of multiple coatings, the long-term oxidation resistance of the coating is better. So, the glass coating is preferred for long-term protection for carbon materials used at low or medium temperature, which is not suitable to improve the oxidation resistance of graphite substrate. In addition, the glass coating contains B and P, so glass coating cannot be used in HTR.
Figure 6 Oxidation model of mullite/SiC coating for C/C composites
Figure 7 Schematic illustrations of oxidation process of coating:
5 Coating techniques
The commonly used coating technology can be divided into two groups. One is that graphite substrate is involved in the reaction to form coatings, such as PC, in-situ formation, reaction infiltration, slurry-sintering. On one hand, a gradient coating can be produced on the matrix to release the mismatch of CTE between the coating and substrate. Meanwhile, a strong bonding will be formed. On the other hand, due to the higher reaction temperature and diffusion reaction, these techniques always cause many structural defects to graphite substrate. So, it is inefficient if that this coating is directly used as an antioxidant coating, it is usually used as an inner coating in the multilayer coating system, and an outer coating is needed.
Another method is that graphite substrate does not take part in the formation of the coatings, such as CVD. In general, the coating preparation temperature of this coating method is lower than the former. The coating obtained by this method is very uniform and compact. And this type of coating has little defects, which can effectively block the diffusion of oxygen. But there is no chemical reaction between the coating and substrate, the bonding between coating and graphite substrate is very weak. There exists an obvious interface between the coating and substrate. So, this type of coating has poor thermal shock resistance and is easy to peel off from substrate. Therefore, this type of coating is usually as an outer coating in the multilayer coating system.
5.1 Pack cementation (PC)
PC is a widely used coating preparation technique. The sample is embedded in the mixed powder and through high temperature sintered to obtain a coating. This technology is very simple and can be used for objects having a complex geometry. The main principle of PC is that the reactant is converted into molten state at high temperature, and then penetrates and diffuses into the substrate to a certain depth and reacts with substrate. So, the process is often performed at the temperature higher than the melting point of the reactants. Therefore, the coating has good adhesion with substrate. In addition, due to the presence of osmotic gradient, it is easy to form a good gradient transition between the coating and substrate, which can alleviate the stress in the process of oxidation and thermal shock. So, the coating obtained by PC technique is often used as the inner layer [34, 75]. ZHOU et al [77] found that the ratio of the raw materials, power size and sintering time would influence the thickness of the coating.
5.2 Chemical vapor deposition (CVD)
CVD is a kind of process to make the gas molecules collide and react with each other, to form stable solid products on the substrate surface to form grain and quickly forming coatings. During the preparation process, gas has high reactivity, strong diffusivity and mixture is more uniform. It can be controlled effectively by adjusting the reaction atmosphere, temperature and reactant concentration to form a ideal coating on the substrate surface [76-79]. The technique is usually used to prepare the SiC layer in tristructural- isotropic (TRISO) particle [80-82]. The biggest limit of this technology is that there is a clear boundary between coating and substrate surface and the stress cracking between the coating and substrate cannot be effectively solved, so the thermal shock resistance of the coating is poor. Due to the fact that the coating has a uniform structure, the coating obtained by this technique is often as an outer layer, which can effectively block the diffusion of oxygen to graphite substrate. As a result, this technology is often combined with other technology to prepare an antioxidation coating [30, 83].
5.3 Plasma spray (PS)
PS is a kind of a technique of material surface strengthening and surface modification. It mainly uses the characteristics of fast heating under strong current, and rapidly reacts to the substrate surface after rapid heating up to high temperature, to form coatings through rapid high-temperature reaction. Because reactants and the substrate surface react at high temperature and high-speed during sputtering and spraying, coating obtained by plasma sprayed has a gradient transition area. In addition, the growth rate and growth time of the coating can be effectively controlled by controlling the reactant content and temperature in the jet [84, 85]. Due to the use of inert gas as the working gas, spraying material is not easy to oxidize. The limit of this technique is that there is a very high requirement for the equipment, so only simple samples can be prepared [19, 86, 87].
5.4 Slurry
The slurry coating process is to mix the reaction powder with water, polyvinyl alcohol and other liquids to form suspension slurry according to certain proportions. Then the substrate is immersed in the suspension to a certain thickness and finally dried and sintered to form coatings. The pores on the substrate surface are the precondition to use this technology. And the obtained coating is loose and has many pores, so the antioxidation ability is limited [88].
Many other coating techniques are still under development to protect C/C composites from oxidation [25, 89-91]. A single coating preparation technique cannot meet the requirement of the application needs. In practice, it is often to combine several techniques together to prepare coatings with excellent antioxidant properties.
6 Summary
Surface coating technique expands the application of C/C composites enormously, which also provides a good reference for the application of matrix graphite in HTR. However, due to the particularity of nuclear reactor, there is still a choice in application of the coating materials. Besides, there are still many challenges in the fields of materials preparation, experimental techniques, and characterization methods for graphite substrate used in HTR. The first is that the coating is usually prepared on bulk substrate, but the preparation conditions may be not suitable for fuel element or cause some damages to it. Secondly, the fuel element is bigger than C/C composites used in the experiment, so there would exist some challenges when the coating is prepared on the actual graphite substrate element. And the mechanical properties and thermal properties of the coated samples also should be tested. At present, the research on the anti-oxidation of graphite substrate shows that SiC coating is the most promising anti-oxidation coating system. The next work should take SiC coating as the center, and further enrich the coating system. Up to now, the anti-oxidation mechanism is only having preliminary data. The preparation mechanism and anti-oxidation mechanism of SiC coating still need a lot of experimental and theoretical calculation to support.
References
[1] SHIBATA T J, SUMITA J Y, TADA T, SAWA K. Development of non-destructive evaluation methods for degradation of HTGR graphite components[J]. Journal of Nuclear Materials, 2008, 381(1, 2): 204-209.
[2] BOEHM H P. Surface oxides on carbon and their analysis: A critical assessment [J]. Carbon, 2002, 40(2): 145-149.
[3] DZYADIKEVICH Y V, OLIINIK V E. Films for protecting graphite materials from oxidation (review) [J]. Powder Metallurgy and Metal Ceramics, 1996, 35(3, 4): 150-153.
[4] JIN Hua, MENG Song-he, ZHANG Xing-hong, ZENG Qing-xuan, NIU Jia-hong. Effects of oxidation temperature, time, and ambient pressure on the oxidation of ZrB2-SiC-graphite composites in atomic oxygen [J]. Journal of the European Ceramic Society, 2016, 36(8): 1855-1861.
[5] LI Lu, LI He-jun, YIN Xue-min, CHU Yan-hui, CHEN Xi, FU Qian-gang. Oxidation protection and behavior of in-situ zirconium diboride-silicon carbide coating for carbon/carbon composites [J]. Journal of Alloys and Compounds, 2015, 645: 164-170.
[6] CHENG Lai-fei, XU Yong-dong, ZHANG Li-tong, GAO Rong. Effect of glass sealing on the oxidation behavior of three dimensional C/SiC composites in air [J]. Carbon, 2001, 39(8): 1127-1133.
[7] KRISZTINA L, ETELKA T, KATALIN J. Effect of activation on the surface chemistry of carbons from polymer precursors [J]. Carbon, 2001, 39(8): 1217-1228.
[8] LU Wei-ming, CHUNG D D L. Oxidation protection of carbon materials by acid phosphate impregnation [J]. Carbon, 2002, 40(8): 1249-1254.
[9] MCKEE D W. Effect of adsorbed phosphorus oxychloride on the oxidation behavior of graphite [J]. Carbon, 1972, 10: 491-497.
[10] JAROSLAW W, AGNIESZKA J, TOMASZ C, ANDRZEJ O. Surface modification of graphene oxide nanoplatelets and its influence on mechanical properties of alumina matrix composites [J]. Journal of the European Ceramic Society, 2017, 37(4): 1587-1592.
[11] CHENG E T. Concentration limits of natural elements in low activation fusion materials [J]. Journal of Nuclear Materials, 1998, 258: 1767-1772.
[12] RAJU Kati, YOON Dang-Hyok. Sintering additives for SiC based on the reactivity: A review [J]. Ceramics International, 2016, 42(16): 17947-17962.
[13] SUN Can, LI He-jun, FU Qian-gang, ZHANG Jia-ping, PENG Han. Double SiC coating on carbon/carbon composites against oxidation by a two-step method [J]. Transactions of Nonferrous Metals Society of China, 2013, 23(7): 2107-2112.
[14] YAO Dong-jia, LI He-jun, WU heng, FU Qian-gang, QIANG Xin-fa. Ablation resistance of ZrC/SiC gradient coating for SiC-coated carbon/carbon composites prepared by supersonic plasma spraying [J]. Journal of the European Ceramic Society, 2016, 36(15): 3739-3746.
[15] ZHANNG Yu-lei, LI He-jun, FU Qian-gang, LI Ke-zhi, WEI Jian, WANG Peng-yun. A C/SiC gradient oxidation protective coating for carbon/carbon composites [J]. Surface and Coatings Technology, 2006, 201(6): 3491-3495.
[16] ZHANG Yu-lei, LI He-jun, YAO Xi-yuan, LI Ke-zhi, ZHANG Shou-yang. C/SiC/Si-Mo-B/glass multilayer oxidation protective coating for carbon/carbon composites [J]. Surface and Coatings Technology, 2011, 206(2, 3): 492-496.
[17] ZHANG Yu-lei, LI He-jun, LI Ke-zhi, FEI Jie, ZENG Xie-rong. C/SiC/Si-Mo-Cr multilayer coating for carbon/ carbon composites for oxidation protection [J]. New Carbon Materials, 2012, 27(2): 105-109.
[18] LI Ting, LI He-jun, SHI Xiao-hong, CHENG Jing, LIU Lei. Mo-Si-Al-C multiphase oxidation protective coating for carbon/carbon composites [J]. Applied Surface Science, 2013, 276: 154-158.
[19] MA Chao, LI He-jun, WU Heng, FU Qian-gang, SUN Can, SHI Xiao-hong, ZHANG Yu-lei, ZHANG Zheng-zhong, TAO Jun, HAN Zhi-hai. Mullite oxidation resistant coating for SiC-coated carbon/carbon composites by supersonic plasma spraying [J]. Journal of Materials Science & Technology, 2013, 29(1): 29-33.
[20] HUANG Jian-feng, LI He-jun, ZENG Xie-rong, XIONG Xin-bo, LI Ke-zhi. Influence of preparation technology on the microstructure and anti-oxidation property of SiC-Al2O3-mullite multi-coatings for carbon/carbon composites [J]. Applied Surface Science, 2006, 252(12): 4244-4249.
[21] HU Cheng-long, PANG Sheng-yang, TANG Su-fang, YANG Zhi, WANG Shi-jun, CHENG Hui-ming. Long-term oxidation behavior of carbon/carbon composites with a SiC/B4C-B2O3-SiO2-Al2O3 coating at low and medium temperatures [J]. Corrosion Science, 2015, 94: 452-458.
[22] CAIRO C A A, GRACA M L A, SILVA C R M, BRESSIANI J C. Functionally gradient ceramic coating for carbon-carbon antioxidation protection [J]. Journal of the European Ceramic Society, 2001, 21(3): 325-329.
[23] REN Xuan-ru, LI He-jun, FU Qian-gang, LI Ke-zhi. Oxidation protective TaB2-SiC gradient coating to protect SiC-Si coated carbon/carbon composites against oxidation [J]. Composites Part B: Engineering, 2014, 66: 174-179.
[24] WANG Yong-jie, LI He-jun, FU Qian-gang, WU Heng, YAO Dong-jia, LI Hai-liang. SiC/HfC/SiC ablation resistant coating for carbon/carbon composites [J]. Surface and Coatings Technology, 2012, 206(19, 20): 3883-3887.
[25] WEN G, SUI S H, SONG L, WANG X Y, XIA L. Formation of ZrC ablation protective coatings on carbon material by tungsten inert gas cladding technique [J]. Corrosion Science, 2010, 52(9): 3018-3022.
[26] SUN Wei, XIONG Xiang, HUANG Bai-yun, LI Guo-dong, ZHANG Hong-bo, CHEN Zhao-ke, ZHENG Xiang-lin. ZrC ablation protective coating for carbon/carbon composites [J]. Carbon, 2009, 47(14): 3368-3371.
[27] MORIMOTO T, OGURA Y, KONDO M, UEDA T. Multilayer coating for carbon-carbon composites [J]. Carbon, 1995, 33(4): 351-357.
[28] ZHOU Ping, LIU Xiao-xue, ZHAO Hong-sheng, LI Zi-qiang, ZHENG Zu-jie, ZHANG Kai-hong, LIU Bing. Micro four-layer SiC coating on matrix graphite spheres of HTR fuel elements by two-step pack cementation [J]. Journal of the American Ceramic Society, 2016, 99(11): 3525-3532.
[29] ZHOU Ping, ZHENG Zu-jie, LIU Rong-zheng, LIU Xiao-xue, LIU Ma-lin, WANG Tao-wei, LI Zi-qiang, SHAO You-lin, ZHAO Hong-sheng, LIU Bing. Dual layer SiC coating on matrix graphite sphere prepared by pack cementation and fluidized-bed chemical vapor deposition [J]. Journal of the American Ceramic Society, 2017, 100(8): 3415-3424.
[30] LI Lu, LI He-jun, LIN Hong-jiao, ZHUANG Lei, WANG Shao-long, FENG Tao, YAO Xi-yuan, FU Qian-gang. Comparison of the oxidation behaviors of SiC coatings on C/C composites prepared by pack cementation and chemical vapor deposition [J]. Surface and Coatings Technology, 2016, 302: 56-64.
[31] TANG Chun-he, GUAN Jie. Improvement in oxidation resistance of the nuclear graphite by reaction-coated SiC coating [J]. Journal of Nuclear Materials, 1995, 224(1): 103-108.
[32] ZHU Qing-shan, QIU Xue-liang, MA Chang-wen. Oxidation resistant SiC coating for graphite materials [J]. Carbon, 1999, 37(9): 1475-1484.
[33] FU Zhi-qiang, TANG Chun-he, LIANG Tong-xiang. Structure of SiC coatings from polycarbosilane on graphite for fuel element matrix of high temperature gas-cooled reactor [J]. Surface and Coatings Technology, 2006, 200(12, 13): 3950-3954.
[34] FU Qian-gang, LI He-jun, SHI Xiao-hong, LI Ke-zhi, SUN Guo-dong. Silicon carbide coating to protect carbon/carbon composites against oxidation [J]. Scripta Materialia, 2005, 52(9): 923-927.
[35] FU Qian-gang, LI He-jun, SHI Xiao-hong, LIAO Xiao-ling, LI Ke-zhi, HUANG Min. Microstructure and anti-oxidation property of CrSi2-SiC coating for carbon/carbon composites [J]. Applied Surface Science, 2006, 252(10): 3475-3480.
[36] ZHAO Juan, GUO Quan-gui, SHI Jing-li, ZHAI Geng-tai, LIU Lang. SiC/Si-MoSi2 oxidation protective coatings for carbon materials [J]. Surface and Coatings Technology, 2006, 201(3, 4): 1861-1865.
[37] LI He-jun, FENG Tao, FU Qian-gang, WU Heng, SHEN Xue-tao. Oxidation and erosion resistance of MoSi2-CrSi2- Si/SiC coated C/C composites in static and aerodynamic oxidation environment [J]. Carbon, 2010, 48(5): 1636-1642.
[38] FANG Hai-tao, ZHU Jing-chuan, YIN Zhong-da, JEON Jae-ho, HAHN Yoo-dong. A Si-Mo fused slurry coating for oxidation protection of carbon-carbon composites [J]. Journal of Materials Science Letters, 2001, 20: 175-177.
[39] HENNIGE V D, HAUSSELT J, RITZHAUPT-KLEISSL H J, WINDMANN T. Shrinkage-free ZrSiO2-ceramics: Characterisation and application [J]. Journal of the European Ceramic Society, 1999, 19: 2901-2908.
[40] SUN Can, LI He-jun, LUO Hui-juan, XIE Jing, ZHANG Jia-ping, FU Qian-gang. Effect of Y2O3 on the oxidation resistant of ZrSiO4/SiC coating prepared by supersonic plasma spraying technique for carbon/carbon composites [J]. Surface and Coatings Technology, 2013, 235: 127-133.
[41] KAISER Arno, LOBERT Markus, TELLE Rainer. Thermal stability of zircon (ZrSiO4) [J]. Journal of the European Ceramic Society, 2008, 28(11): 2199-2211.
[42] LANG Maik, ZHANG Fu-xiang, LIAN Jie, TRAUTMANN Christina, NEUMANN Reinhard, EWING Rodney C. Irradiation-induced stabilization of zircon (ZrSiO4) at high pressure [J]. Earth and Planetary Science Letters, 2008, 269(1, 2): 291-295.
[43] POURASAD Jalil, EHSANI Naser, VALEFI Zia KHALIFESOLTANI Sayed Ali. Preparation of a nanostructured SiC-ZrO2 coating to improve the oxidation resistance of graphite [J]. Surface and Coatings Technology, 2017, 323: 58-64.
[44] DONG Z J, LIU S X, LI X K, WESTWOOD A, YUAN G M, CUI Z W, CONG Y. Influence of infiltration temperature on the microstructure and oxidation behavior of SiC-ZrC ceramic coating on C/C composites prepared by reactive melt infiltration [J]. Ceramics International, 2015, 41(1): 797-811.
[45] WANG Shao-long, LI Ke-zhi, LI He-jun, ZHANG Yu-lei. Microstructure and ablation resistance of ZrC nanostructured coating for carbon/carbon composites [J]. Materials Letters, 2013, 107: 99-102.
[46] WANG Shao-long, LIN Ke-zhi, LI He-jun, ZHANG Yu-lei, FENG Tao. Structure evolution and ablation behavior of ZrC coating on C/C composites under single and cyclic oxyacetylene torch environment [J]. Ceramics International, 2014, 40(10): 16003-16014.
[47] WANG Peng, ZHOU Shan-bao, ZHANG Xing-hong, GUI Kai-xuan, LI Yong-xia, AN Jia-dong, HAN Wen-bo. Thermal cycling and oxidation resistance of B modified ZrB2-SiC coatings on SiC coated graphite [J]. Surface and Coatings Technology, 2015, 280: 330-337.
[48] JALIL Pourasad, NASER Ehsani. In-situ synthesis of SiC-ZrB2 coating by a novel pack cementation technique to protect graphite against oxidation [J]. Journal of Alloys and Compounds, 2017, 690: 692-698.
[49] YAO Xi-yuan, LI He-jun, ZHANG Yu-lei, WU Heng, QIANG Xin-fa. A SiC-Si-ZrB2 multiphase oxidation protective ceramic coating for SiC-coated carbon/carbon composites [J]. Ceramics International, 2012, 38(3): 2095-2100.
[50] WANG Peng, HAN Wen-bo, ZHANG Xing-hong, LI Ning, ZHAO Guang-dong, ZHOU Shan-bao. (ZrB2-SiC)/SiC oxidation protective coatings for graphite materials [J]. Ceramics International, 2015, 41(5): 6941-6949.
[51] LI Shi-bin, LU Zhen-lin, GAO Ji-qiang, JIN Zhi-hao. A study on the cycling oxidation behavior of mullite-coated silicon carbide [J]. Materials Chemistry and Physics, 2003, 78(3): 655-659.
[52] LIU Wen-zhong, XIAO Peng, LI Zhuan, HONG Wen, LUO Heng, YU Xiao-yu, LI Yang, CHEN Wen-bo. Microstructure and oxidation behavior of sol-gel mullite coating on SiC-coated carbon/carbon composites [J]. Journal of the European Ceramic Society, 2015, 35(14): 3789-3796.
[53] YANG Xin, SU Zhe-an, HUANG Qi-zhong, CHAI Li-yuan. Preparation and oxidation resistance of mullite/SiC coating for carbon materials at 1150 °C [J]. Transactions of Nonferrous Metals Society of China, 2012, 22(12): 2997-3002.
[54] JACOBSON N S. Corrosion of silicon-based ceramics in combustion environments [J]. Journal of the American Ceramic Society, 1993, 76(1): 3-28.
[55] WANG Kai-tong, CAO Li-yun, HUANG Jian-yeng, FEI Jie. A mullite/SiC oxidation protective coating for carbon/carbon composites [J]. Journal of the European Ceramic Society, 2013, 33(1): 191-198.
[56] FU Qian-gang, LI He-jun, LI Ke-zhi, SHI Xiao-hong, HUANG Min. A SiC/glass oxidation protective coating for carbon/carbon composites for application at 1173 K [J]. Carbon, 2007, 45(4): 892-894.
[57] HUANG Jian-feng, LI He-jun, ZENG Xie-rong, LI Ke-zhi, XIONG Xin-bo, HUANG Min, ZHANG Xiu-lian, LIU Ying-lou. A new SiC/yttrium silicate/glass multi-layer oxidation protective coating for carbon/carbon composites [J]. Carbon, 2004, 42(11): 2356-2359.
[58] WAN Feng, LUO Fa, ZHOU Ying-ying, ZHOU Wan-cheng, ZHU Dong-mei. A glass coating for SiC fiber reinforced aluminum phosphate matrix (SiCf/AlPO4) composites for high-temperature absorbing wave applications [J]. Surface and Coatings Technology, 2015, 264: 9-16.
[59] HUANG Jian-feng, ZHANG Yong-liang, ZHU Kong-jun, LI Cui-yan, CAO Li-yun, ZHOU Lei, OUYANG Hai-bo, ZHANG Bo-ye, HAO Wei, YAO Chun-yan. Microstructure and oxidation protection of borosilicate glass coating prepared by pulse arc discharge deposition for C/C composites [J]. Ceramics International, 2015, 41(3): 4662-4667.
[60] FU Qian-gang, LI He-jun, SHI Xiao-hong, LI Ke-zhi, WEI Jian, HUANG Min. Oxidation protective glass coating for SiC coated carbon/carbon composites for application at 1773 K [J]. Materials Letters, 2006, 60(3): 431-434.
[61] FU Qian-gang, LI He-jun, SHI Xiao-hong, LI Ke-zhi, WANG Chuang, HUANG Min. Double-layer oxidation protective SiC/glass coatings for carbon/carbon composites [J]. Surface and Coatings Technology, 2006, 200(11): 3473-3477.
[62] WANG Shu-qiu, ZENG Fan-hao, LI Yi, GU Yi, ZHANG Fu-qin, XIONG Xiang. Oxidation mechanism of SiC-zirconia-glass ceramic coated carbon/carbon composites at 1123-1273 K [J]. Materials Research Bulletin, 2017, 91: 189-196.
[63] BRANDL W, GRABKE H J, TOMA D, KRUGER J. The oxidation behaviour of sprayed MCrAlY coatings [J]. Surface and Coatings Technology, 1996, 86-87: 41-47.
[64] TIMOTHY J Foley, CURTIS E Johnson, KELVIN T Higa. Inhibition of oxide formation on aluminum nanoparticles by transition metal coating [J]. Chem Mater, 2005, 17(16): 4086-4091.
[65] ZHANG Y, FRANKLIN N W, CHEN R J, DAI H J. Metal coating on suspended carbon nanotubes and its implication to metal-tube interaction [J]. Chemical Physics Letters, 2000, 331(1): 35-41.
[66] HUANG Min, LI Ke-zhi, LI He-jun, FU Qian-gang, SUN Guo-dong. Double-layer oxidation protective SiC/Cr-Al-Si coating for carbon-carbon composites [J]. Surface and Coatings Technology, 2007, 201(18): 7842-7846.
[67] SHI Xiao-hong, WANG Chang-cong, LIN Hong-jiao, HUO Cai-xia, JIN Xiu-xiu, SHI Guo-ge, DONG Kai-yuan. Oxidation resistance of a La-Mo-Si-O-C coating prepared by supersonic atmosphere plasma spraying on the surface of SiC-coated C/C composites [J]. Surface and Coatings Technology, 2016, 300: 10-18.
[68] LI Ke-zhi, HOU Dang-she, LI He-jun, FU Qian-gang, JIAO Geng-sheng. Si-W-Mo coating for SiC coated carbon/carbon composites against oxidation [J]. Surface and Coatings Technology, 2007, 201(24): 9598-9602.
[69] LIU Rong-zheng, LIU Bing, ZHANG Kai-hong, LIU Ma-lin, SHAO You-lin, TANG Chun-he. High temperature oxidation behavior of SiC coating in TRISO coated particles [J]. Journal of Nuclear Materials, 2014, 453(1-3): 107-114.
[70] ZHAO Hong-sheng, FU Zhi-qiang, TANG Chun-he, LIU Xiao-xue, LI Zi-qiang, ZHANG Kai-hong. Study of SiC/SiO2 oxidation-resistant coatings on matrix graphite for HTR fuel element [J]. Nuclear Engineering and Design, 2014, 271: 217-220.
[71] FU Zhi-qiang, TANG Chun-he, LIANG Tong-xiang, ROBIN Jean-Charles. Oxidation of SiC and decomposition of SiO2 at low partial pressure of oxygen in He-O2 system [J]. Nuclear Engineering and Design, 2004, 234(1-3): 45-49.
[72] FU Zhi-qiang, WANG Cheng-biao, TANG Chun-he, ZHAO Hong-sheng, ROBIN Jean-Charles. Oxidation behaviors of SiO2/SiC coated matrix graphite of high temperature gas-cooled reactor fuel element [J]. Nuclear Engineering and Design, 2013, 265: 867-871.
[73] LI Guo-dong, XIONG Xiang, HUANG Ke-long. Ablation mechanism of TaC coating fabricated by chemical vapor deposition on carbon-carbon composites [J]. Transactions of Nonferrous Metals Society of China, 2009, 19: s689-s695.
[74] WANG Shao-long, KE Zhi-li, HE Jun-li, YU Lei-zhang, WEI Yang-zhang. Ablation behavior of CVD-ZrC coating under oxyacetylene torch environment with different heat fluxes [J]. International Journal of Refractory Metals and Hard Materials, 2015, 48: 108-114.
[75] ZHANG Bo, HUANG Jian-feng, OUYANG Hai-bo, CAO Li-yun, LI Cui-yan. A mullite oxidation protective coating on SiC coated carbon/carbon composites by hot dipping [J]. Ceramics International, 2016, 42(15): 17932-17935.
[76] HUANG Jian-feng, ZENG Xie-rong, LI He-jun, XIONG Xin-bo, HUANG Min. Mullite-Al2O3-SiC oxidation protective coating for carbon/carbon composites [J]. Carbon, 2003, 41(14): 2825-2829.
[77] ZHOU Ping, LI Zi-qiang, ZHAO Hong-sheng, ZHANG Kai-hong, LIU Xiao-xue, LIU Bing. Sintering of SiC coating layer on graphite spheres prepared by pack cementation [J]. Key Engineering Materials, 2016, 697: 807-813.
[78] CHENG L F, XU Yong-dong, ZHANG Li-tong, YIN Xiao-wei. Preparation of an oxidation protection coating for C/C composites by low pressure chemical vapor deposition [J]. Carbon, 2000, 38: 1493-1498.
[79] CHOY K L. Chemical vapour deposition of coatings [J]. Progress in Materials Science, 2003, 48(2): 57-170.
[80] NARUSHIMA T, GOTO T, IGUCHI Y, HIRAI T. High-temperature oxidation of chemically vapor-deposited silicon-carbide in wet oxygen at 1823 to 1923 K [J]. Journal of the American Ceramic Society, 1990, 73: 3580-3584.
[81] LUO Zheng, ZHOU Xin-gui, YU Jin-shan. High-tomperature mechanical properties of thermal barrier coated SiC/SiC composites by PIP process with a new precursor polymer [J]. Surface and Coating Technology, 2014, 258: 146-153.
[82] YANG Xin, HUANG Qi-zhong, ZOU Yan-hong, CHANG Xin, SU Zhe-an, ZHANG Ming-yu, XIE Zhi-yong. Anti-oxidation behavior of chemical vapor reaction SiC coatings on different carbon materials at high temperatures [J]. Transactions of Nonferrous Metals Society of China, 2009, 19(5): 1044-1050.
[83] LIU Rong-zheng, LIU Ma-lin, CHANG Jia-xing, SHAO You-lin, LIU Bing. An improved design of TRISO particle with porous SiC inner layer by fluidized bed-chemical vapor deposition [J]. Journal of Nuclear Materials, 2015, 467: 917-926.
[84] LIU Rong-zheng, LIU Ma-lin, WANG Zi-liang, SHAO You-lin, CHANG Jia-xing, LIU Bing, WANG Yong-xin. Preparation of fine grained SiC layer by fluidized bed chemical vapor deposition with pulsed propylene [J]. Journal of the American Ceramic Society, 2016, 99(6): 1870-1873.
[85] NIU Fang-xu, WANG Yan-xiang, ABBAS Imran, FU Shan-long, WANG Cheng-guo. A MoSi2-SiOC-Si3N4/SiC anti-oxidation coating for C/C composites prepared at relatively low temperature [J]. Ceramics International, 2017, 43(3): 3238-3245.
[86] CHEN Zhou, WU Wang-ping, CHEN Zhao-feng, CONG Xiang-na, QIU Jin-lian. Microstructural characterization on ZrC doped carbon/carbon composites [J]. Ceramics International, 2012, 38(1): 761-767.
[87] YANG Yang, LI Ke-zhi, ZHAO Zhi-gang, LI He-jun. Ablation resistance of HfC-SiC coating prepared by supersonic atmospheric plasma spraying for SiC-coated C/C composites [J]. Ceramics International, 2016, 42(4): 4768-4774.
[88] HU Cui, NIU Ya-ran, HUANG San-song, LI Hong, REN Mu-su, ZENG Yi, ZHENG Xue-bin, SUN Jin-liang. In-situ fabrication of ZrB2-SiC/SiC gradient coating on C/C composites [J]. Journal of Alloys and Compounds, 2015, 646: 916-923.
[89] NIU Ya-ran, ZHENG Xue-bin, DING Chuan-xian, LI Hong, HU Cui, REN Mu-su, SUN Jin-liang. Microstructure characteristics of silicon carbide coatings fabricated on C/C composites by plasma spraying technology [J]. Ceramics International, 2011, 37(5): 1675-1680.
[90] WU Wang-ping, CHEN Zhao-feng, CHENG Han, WANG Liang-bing, ZHANG Ying. Tungsten and iridium multilayered structure by DGP as ablation-resistance coatings for graphite [J]. Applied Surface Science, 2011, 257(16): 7295-7304.
[91] FILIPESCU M, VELISA G, ION V, ANDREI A, SCINTEE N, IONESCU P, STANCIU S G, PANTELICA D, DINESCU M. Silicon carbide thin films as nuclear ceramics grown by laser ablation [J]. Journal of Nuclear Materials, 2011, 416(1, 2): 18-21.
(Edited by YANG Hua)
中文导读
HTR球形燃料元件基体石墨抗氧化涂层的研究进展
摘要:石墨是一种优良的固体慢化材料和结构材料,具有良好的辐照性能,是高温超导材料的核心成分。然而,石墨在高温下易被氧化,提高基体石墨的抗氧化性能是保证高温气冷堆(HTR)安全性的重要途径。研究表明涂层法可以有效地提高基体石墨的抗氧化性能。涂层体系的选择包括材料体系和制备方法,并且涂层的抗氧化机理也是十分重要的。碳化硅基涂层是HTR中应用最广的涂层体系,其他涂层体系如锆化合物涂层、贵金属涂层应用也比较广泛。但是由于一些涂层中所含元素不能用于高辐照环境,一些涂层体系不适合应用在HTR中。本文重点综述了一些常用涂层体系的抗氧化机理,并且对常见的涂层制备方法进行了总结,如包埋(PC)、化学气相沉积(CVD)、等离子喷涂(PS)、浆料涂覆法(slurry)等。最后,提出了一些现有抗氧化涂层体系中存在的问题,并且对核用中石墨基体表面抗氧化涂层的研究进行了展望。
关键词:石墨;涂层;核;氧化;机理
Foundation item: Project(ZX06901) supported by National S&T Major Project of China; Project(21671116) supported by the National Natural Science Foundation of China
Received date: 2018-06-08; Accepted date: 2019-06-14
Corresponding author: ZHAO Hong-sheng, PhD, Professor; Tel: +86-10-89796090; E-mail: hshzhao@tsinghua.edu.cn; ORCID: 0000- 0002-6829-3204
Abstract: The core components of HTR mainly consist of graphite, which is an excellent solid moderating material and structure material with good irradiation performance. However, graphite is easier to be oxidized at high temperature. It is important to improve the anti-oxidation performance of graphite substrate to enhance the safety of HTR. Anti-oxidation coating has been proved that it can effectively improve the oxidation resistance of graphite substrate. The selection of coating includes the coating materials system and the preparation techniques. In addition, attention also should be paid to the anti-oxidation mechanism. SiC based coating system is most commonly used in HTR and some other coating systems such as zirconium compounds coating, noble metal coating also can be used in nuclear. However, some coating systems are not suitable in HTR because the elements contained cannot be used in high irradiation environment. The emphasis is on the antioxidant mechanism of different coating systems. Some anti-oxidation coating techniques for graphite substrate are also reviewed in this paper, such as PC, CVD, PS and slurry. Finally, the existing problems of the oxidation resistance coating are proposed and an outlook is made for the development of the protection coating system for graphite substrate used in nuclear.
- Review of oxidant resistant coating on graphite substrate of HTR fuel element
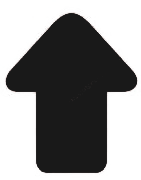