- Abstract:
- 1 Introduction▲
- 2 Experimental▲
- 3 Results and discus...▲
- 4 Conclusions▲
- References
- Figure
- Fig. 1 Variations of mechanical properties with annealing temperature in annealing treatment for 1 h
- Fig. 2 Variations of mechanical properties with annealing time in annealing treatment at 300 ℃
- Fig. 3 Microstructures of alloy at various annealing temperatures: (a) Cold rolling; (b) Annealed at 250 ℃ for 1 h; (c) Annealed at 400 ℃ for 1 h; (d) Annealed at 450 ℃ for 1 h; (e) Annealed at 500 ℃ for 1 h; (f) Annealed at 550 ℃ for 1 h
- Fig. 4 Fractograph (a) and EDS pattern (b) of alloy annealed at 350 ℃ for 1 h
- Fig. 5 TEM images of alloy at various annealing temperatures: (a) Cold rolling; (b) Annealed at 200 ℃ for 1 h; (c) Annealed at 300 ℃ for 1 h; (d-f) Annealed at 350 ℃ for 1 h; (g, h) Annealed at 450 ℃ for 1 h
J. Cent. South Univ. (2012) 19: 1785-1790
DOI: 10.1007/s11771-012-1208-x
Microstructure and mechanical properties of Al-5.8Mg-Mn-Sc-Zr alloy after annealing treatment
CHEN Qin(陈琴)1, PAN Qing-lin(潘清林)1, 2, WANG Ying(王迎)1,
ZAHNG Zhi-ye(张志野)1, ZHOU Jian(周坚)1, LIU Chang(刘畅)1
1. School of Materials Science and Engineering, Central South University, Changsha 410083, China;
2. Key Laboratory of Nonferrous Materials Science and Engineering of Ministry of Education
(Central South University), Changsha 410083, China
? Central South University Press and Springer-Verlag Berlin Heidelberg 2012
Abstract:
An Al-5.8Mg-0.4Mn-0.35(Sc+Zr) (mass fraction, %) alloy sheet was prepared using water chilling copper mould ingot metallurgy processing which was protected by active flux. The influence of stabilizing annealing on mechanical properties and microstructure of the cold rolling sheet was studied. The results show that the strength and hardness of the alloy decrease, while the elongation increases with increasing the stabilizing annealing temperature. With the increase of stabilizing annealing time, the strength and hardness of the alloy drop slightly but its ductility exhibits no change. Partial recovery and recrystallization orderly occur with the increase of annealing temperature during stabilizing treatment. Only different degrees of recovery occur in the alloys annealed below 400 ℃ for 1 h. Partial recrystallization occurs after annealed at 450 ℃ for 1 h. By annealing at 300 ℃ for 1 h, the alloy can obtain the optimum application values of σb, σ0.2 and δ, which are 436 MPa, 327 MPa and 16.7%, respectively.
Key words:
1 Introduction
As lightweight structural material with a good combination of mechanical properties and cast ability, Al-Mg-Mn alloy has been applied extensively in the field of microelectronic, automotive and aerospace industries. However, applications of Al-Mg-Mn alloy are still restrained due to the limited mechanical properties. In order to further improve the structure and properties of Al-Mg-Mn alloys, many experiments have been performed extensively. Experimental investigations [1-5] showed that addition of alloying elements especially rare earth elements could significantly improve the mechanical properties as well as thermal stability and corrosion behavior.
The microstructure and properties of A1-Mg-Mn alloy are strongly affected by the simultaneous addition of small quantities of scandium and zirconium. Sc and Zr additions to Al alloys result in a significant refinement of as-cast grain size, an increase in mechanical properties and improvement of corrosion resistance and weldability [6-8]. This is because of the precipitates of the complex phase Al3(Sc, Zr) during alloy solidification from the melt and during homogenization of as-cast alloy. Experimental investigations [1-3] showed that the increase in strength mainly came from grain refining strengthening, substructure strengthening and precipitation strengthening caused by Al3(Sc, Zr) particles [9-10]. Because of the presence of Al3(Sc,Zr) precipitates, Al-Mg-Mn-Sc-Zr alloy retains its deformed microstructure even when annealed at very high temperature. Deformation energy proceeds by recovery and it is very difficult to obtain a fully recrystallized microstructure.
After cold rolling, dislocation density in the alloy increases dramatically; as a result, the strength, hardness and corrosion resistance increase while ductility declines. Due to cold deformation, the free energy of Al-Mg-Mn-Sc-Zr alloy rises, so that the properties and microstructure become unstable. For the aluminum alloys containing high magnesium content, when stored at room temperature for long term, they are easy to arouse aging softening phenomenon; therefore, it is indispensable to conduct stabilizing treatments to cold-deformed alloys. The alloys can obtain stable mechanical properties and large corrosion resistance after stabilizing treatments [11-12].
Recent studies on the Al-Mg-Mn alloys are mainly concentrated on low-magnesium alloys, and primarily on the effect of rare earth elements on the properties of these alloys, while researches on the actual application performance and the industrial production are seldom. So, in this work, the effect of stabilizing treatment on the hardness, tensile properties and microstructure of cold-deformed Al-Mg-Mn-Sc-Zr alloy was studied, and then the optimal stabilizing treatment of this alloy was obtained.
2 Experimental
The material used in this work is Al-5.8%Mg-0.4%Mn-0.25%Sc-0.1%Zr (mass fraction). The alloy was prepared with pure Al, Mg and Al-Mn, Al-Sc, Al-Zr master alloys by ingot metallurgy in a crucible and then pouring into a copper book-shaped mould (260 mm×150 mm×30 mm). The ingots were homogenized at 460 ℃ for 24 h, milled to 25 mm in thickness, and hot-rolled to 5.7 mm (77% rolling reduction). The hot rolling temperature was 470 ℃. Hot rolling was followed by intermediate annealing at 400 ℃ for 2 h. And then, the hot-rolled sheets were cold-rolled to a thickness of 2.0 mm (65% reduction). The cold-rolled sheets were annealed at 100, 150, 200, 250, 300, 325, 350, 400, 450 and 500 ℃ for 1 h, and 300 ℃ for 1, 8, 16 and 24 h, respectively.
All the tensile specimens were prepared according to GB/T 228—2002. The mechanical properties were measured on a MTS-858 tensile machine with a crosshead speed of 2 mm/min and the tensile axis parallel to the rolling direction. The hardness was tested on a 401MVD MICRO-VICKEERS. The optical microscopy of the specimens was observed by polar light after electro-polishing using an electrolyte solution consisting of 10% HClO3 and 90% ethanol (volume fraction). The micrographs of fractures were observed by scanning electronic microscope (SEM, FEI QUANTA- 200). Thin foils for transmission electron microscopy (TEM) analysis were prepared by twin-jet polishing with an electrolyte solution consisting of 25% HNO3 and 75% methanol (volume fraction) below -25 ℃. The foils were observed on Tecnai G2 20 at 200 kV.
3 Results and discussion
3.1 Mechanical properties
Figure 1 shows the results of longitudinal mechanical properties through stabilizing treatment at different stabilizing annealing temperatures for 1 h. A proper annealing temperature can be concluded from the curves. Figure 1(a) shows the variations of hardness with annealing temperature. From Fig. 1(a), it may be seen that the hardness of Al-Mg-Mn-Sc-Zr alloy declines slowly with increasing the annealing temperature while that of Al-Mg-Mn alloy declines obviously from 250 ℃ to 350 ℃. Figure 1(b) shows the variations of mechanical properties with annealing temperature. It can be seen that tensile strength and yield strength of the alloy decrease, while the elongation increases with increasing the annealing temperature. Besides, the strength of the alloy decreases significantly from 300 ℃ to 350 ℃ and then has a slight variation until 500 ℃, so after 500 ℃ high-temperature annealing, the alloy still maintain a high strength, with σb≥380 MPa and σ0.2≥225 MPa. Research [13] showed that in a certain temperature range, the corrosion resistance of Al-Mg alloys becomes better with elevating the annealing temperature. In order to make a compromise for achieving high strength, good plasticity and large corrosion resistance, the desirable temperature for stabilizing treatment is recommended to be 300 ℃.
Figure 2 reveals the relations between longitudinal mechanical properties and annealing time of the alloy in stabilizing treatment at 300 ℃. With the increase of stabilizing annealing time, the strength drops slightly but plasticity of the alloy exhibits no change.
3.2 Microstructure
3.2.1 Optical microscopy
The optical micrographs of the alloy are shown in Fig. 3. The grains of cold-rolled alloy are elongated along the rolling direction, presenting obvious fibrous rolling deformation organization. Due to the anisotropism of the alloy, the corrosion occurring on the surface of the alloy is nonuniform during electrolytic polishing, so the surface of the deformation organization is undulating, as shown in Fig. 3(a). After annealed at 400 ℃ for 1 h, the alloy still keeps fibrous rolling deformation organization, as shown in Fig. 3(c). When the temperature increases to 450 ℃, partial recrystallization with very fine recrystallization grains are observed in Fig. 3(d). After annealed at 550 ℃ for 1 h, the cold rolled alloy has not yet fully recrystallized, and the alloy still keeps fibrous rolling deformation organization, as shown in Fig. 3(f). Referring to the research of CHEN et al [14], it can be seen the recrystallization temperature of Al-5.8Mg-0.4Mn-0.35 (Sc+Zr) alloy is much higher than the Sc and Zr-free Al-Mg-Mn alloy, which is fully recrystallized even after annealed at 360 ℃ for 1 h.
Fig. 1 Variations of mechanical properties with annealing temperature in annealing treatment for 1 h
Fig. 2 Variations of mechanical properties with annealing time in annealing treatment at 300 ℃
Fig. 3 Microstructures of alloy at various annealing temperatures: (a) Cold rolling; (b) Annealed at 250 ℃ for 1 h; (c) Annealed at 400 ℃ for 1 h; (d) Annealed at 450 ℃ for 1 h; (e) Annealed at 500 ℃ for 1 h; (f) Annealed at 550 ℃ for 1 h
Besides, it can be observed that there are many black particles in the optical micrographs. According to the fractograph and EDS pattern of the alloy, the black particles are complex compounds containing Sc and Zr elements, as shown in Fig. 4. The evolution of the microstructure is found to correlate with the tensile strength of the alloys. From Fig. 4, we can also see that the fractograph exhibits typical ductile dimple fracture pattern, which indicates that the alloy has good ductility when annealed at 350 ℃ for 1 h.
Fig. 4 Fractograph (a) and EDS pattern (b) of alloy annealed at 350 ℃ for 1 h
3.2.2 Transmission electron microscopy (TEM)
The TEM micrographs (TEM) of the alloy through stabilizing treatment at different stabilizing annealing temperatures for 1 h are presented in Fig. 5. It is quite visible that the grains of the cold-rolled alloy display a fibrous structure along the rolling deformation direction, with high-density dislocations and intense stress field in the alloy, as shown in Fig. 5(a). The cold-rolled alloy has high-density dislocations and a large number of dislocation tangles, which makes it difficult for dislocations to slip. And there are strong interaction of the dispersed Al3(Sc, Zr) particles with dislocations in the matrix of the alloy [15-19], so the cold-rolled alloy has high hardness, strength and low ductility.
After annealed at 200 ℃ for 1 h, some areas of the alloy still keep a large number of dislocation tangles, while the dislocation density in the grain and the entwining degree of dislocation decrease in most areas of the alloy. Meanwhile, the dislocation rearranges and sparsely distributes near grain boundaries, forming clear cellular substructure, as shown in Fig. 5(b). From Fig. 5(c), it can be seen that some low-angle sub-grains are formed in some areas of the alloy after annealed at 300 ℃ for 1 h, and there are spherical second phase particles existing in the alloy. Besides, it can be observed that the second phase particles strongly pin sub-grain boundaries and dislocations. As a result of partial recovery, the dislocation density decreases, thus, the hardness and strength of the alloy decrease slightly.
Further increasing the annealing temperature to 350 ℃, the recovery process in the matrix of the alloy is very obvious. Owing to the bow-out of grain boundary migration, many low-angle sub-boundaries and sub-grains are formed, and the size of the sub-grains is inhomogeneous. It can also be observed clearly that there are many dispersed Al3(Sc, Zr) particles in the alloy, which are petal-shaped and coherent with the matrix of the alloy. These Al3(Sc, Zr) particles can still strongly pin sub-grain boundaries, as shown in Figs. 5(d)-(f). Because of obvious recovery, the dislocation density decreases obviously, and many low-angle sub- boundaries and sub-grains are formed; therefore, the hardness and strength of the alloy decrease sharply.
After annealed at 450 ℃ for 1 h, the matrix has been completely recovered and formed high-angle boundaries and grains. A large number of Al3(Sc, Zr) particles dispersedly distribute in the matrix, and the particles are still coherent with the matrix without coarsening, as shown in Figs. 5(g) and (h). At this moment, both the number and the size of sub-grains increase and partial recrystallization occurs; thereby, the hardness and strength of the alloy continue to decrease and the ductility continues to increase.
When adding Sc and Zr into aluminum alloys simultaneously, part of Sc in the Al3Sc (or Al3Zr) phase is substituted by Zr (or Sc). Thus, Al3(Sc, Zr) complex particles are formed. These particles have higher thermal stability and are susceptible to coagulation to a less extent during heating [20]. The high thermal stability of Al3(Sc, Zr) particles makes some benefits for inhibiting recrystallization and reserving work-hardening, so hardness and strength of alloy basically maintain unchanged with the increase of stabilizing annealing time.
TEM observation indicates that Al3(Sc, Zr) particles strongly pin dislocations and sub-grain boundaries, which explains why the recrystallization of the alloy containing Sc and Zr is restrained. The strong drag effect of these precipitates is due to the fact that they are coherent with Al matrix and very thermally stable against loss of coherency and coarsening. Recrystallization occurs only after these precipitates have lost their coherency [21-22]. The results indicate that even a temperature as high as 450 ℃ is not sufficient for the elimination of the inhibiting effect of Al3(Sc, Zr) precipitates on recrystallization.
Fig. 5 TEM images of alloy at various annealing temperatures: (a) Cold rolling; (b) Annealed at 200 ℃ for 1 h; (c) Annealed at 300 ℃ for 1 h; (d-f) Annealed at 350 ℃ for 1 h; (g, h) Annealed at 450 ℃ for 1 h
Besides, micro-alloying elements dissolved in the metals can significantly increase the recrystallization temperature. Especially, when the difference between adding elements and solute atoms is very great or the critical solid solubility of the adding elements in the solvent is small, the effect on improving the recrystallization temperature is significant. This is because the interaction of solute atoms and crystal defects has close relationship with the size and valence number of solute atoms. The high binding energy of the solute atoms to these defects makes it more effective to impede the movement of crystal defects, and it will contribute to the formation and growth of the sub-grain in the heating process; thus, it can significantly increase the recrystallization temperature. Consequently, the alloy is strengthened.
4 Conclusions
1) With increasing the stabilizing annealing temperature, the strength and hardness of Al-5.8Mg-0.4Mn-0.25Sc-0.1Zr (mass fraction, %) alloy decrease, while the elongation increases; with the increase of stabilizing annealing time, the strength and hardness drop slightly but plasticity of the alloy exhibits no change. By annealing at 300 ℃ for 1 h, the alloy can obtain the optimum application values of σb, σ0.2 and δ, which are 436 MPa, 327 MPa and 16.7%, respectively.
2) Partial recovery and recrystallization orderly occur with the increase of annealing temperature during stabilizing treatment when annealed below 400 ℃ for 1 h, and only different degrees of recovery occurs in the alloy; when annealed at 450 ℃ for 1 h, partial recrystallization occurs, and this process does not finish even annealed at 550 ℃ for 1 h.
3) Sc and Zr elements in Al-Mg-Mn alloys mainly exist as Al3(Sc, Zr) complex particles. These particles have obvious effect on improving strength and inhibiting recrystallization.
References
[1] LATHABAI S, LLOYD P G. The effect of scandium on the microstructure, mechanical properties and weldability of a cast Al-Mg alloy [J]. Acta Materialia, 2002, 50(17): 4275-4292.
[2] KUMAR N, MISHRA R S, HUSKAMP CS, SANKARAN K K. Critical grain size for change in deformation behavior in ultrafine grained Al-Mg-Sc alloy [J]. Scripta Materialia, 2011, 64(6): 576-579.
[3] LEE S, UTSUNOMIYA A, AKAMATSU H, NEISHI K, FURUKAWA M, HORITA Z, LANGDON T G. Influence of scandium and zirconium on grain stability and superplastic ductilities in ultrafine-grained Al-Mg alloys [J]. Acta Materialia, 2002, 50(3): 553-564.
[4] MAENG D Y, LEE J H, HONG S I. The effect of transition elements on the superplastic behavior of Al-Mg alloys [J]. Materials Science and Engineering A, 2003, 357(1/2): 188-195.
[5] DU Gang, YAN De-sheng, RONG Li-jian. Influence of intermediate annealing temperatures on mechanical properties of repeatedly cold-rolled Al-Mg-Sc-Zr alloy [J]. Acta Metallurgica Sinica, 2008, 44(10): 1209-1212.
[6] HE Zhen-bo. Research on the structure and properties of welding joint for alloy Al-Mg-(Sc, Zr) [J]. Light Alloy Fabricaton Technology, 2006,34(8): 44-47.
[7] CHEN Su-li, JIANG Feng, YIN Zhi-min, LEI Xue-feng, NIE Bo. Microstructure and properties of Al-Mg-Sc alloy weld joints filled with Al-Mg-Zr and Al-Mg-Zr-Sc weld wires [J]. The Chinese Journal of Nonferrous Metals, 2006,16(5): 835-840. (in Chinese)
[8] PENG Yong-yi, YIN Zhi-min, LEI Xue-feng, PAN Qing-lin, HE Zhen-bo. Microstructure and properties of friction stir welded joints of Al-Mg-Sc alloy plates [J]. Rare Metal Materials and Engineering, 2011,40(2): 201-205. (in Chinese)
[9] ROUMINA R, SINCLAIR C W. Recovery kinetics in the presence of precipitates: The softening response of an Al-Mg-Sc alloy [J]. Acta Materialia, 2010, 58(1): 111-121.
[10] MARQUIS E A, SEIDMAN D N. Coarsening kinetics of nanoscale Al3Sc precipitates in an Al-Mg-Sc alloy [J]. Acta Materialia, 2005, 53(15): 4259-4268.
[11] NIE Bo, YIN Zhi-min, JIANG Feng, JIANG Chun-li, CONG Fu-guan. Influence of stabilizing annealing on tensile property and exfoliation corrosion resistance of Al-Mg-Sc alloy [J]. Transactions of Materials and Heat Treatment, 2008, 29(3): 58-61.
[12] SMOLA B, STULIKOVA I, OCENASEK V, PELCOVA J, NEUBERT V. Annealing effects in Al-Sc alloys [J]. Materials Science and Engineering A, 2007, 462(1/2): 370-374.
[13] PENG Yong-yi, YIN Zhi-min, YANG Jin, DU Yu-xuan. In-plane anisotropy of 1545 aluminum alloy sheet [J]. Transactions of Nonferrous Metals Society of China, 2005, 15(1): 87-92.
[14] CHEN Xian-ming, PAN Qing-lin, ZHOU Chang-rong, YIN Zhi-min. Effects of minor scandium addition on microstructure and tensile properties of Al-Mg-Mn alloy [J]. Chinese Journal of Rare Metals, 2003, 27(3): 403-405.
[15] CLOUET E, BARBU A, LAE L, MARTIN G. Precipitation kinetics of Al3Zr and Al3Sc in aluminum alloys modeled with cluster dynamics [J]. Acta Materialia, 2005, 53(8): 2313-2325.
[16] FAZELI F, POOLE W J, SINCLAIR C W. Modeling the effect of Al3Sc precipitates on the yield stress and work hardening of an Al-Mg-Sc alloy [J]. Acta Materialia, 2008, 56(9): 1909-1918.
[17] DU Gang, YANG Wen, YAN De-sheng, RONG Li-jian. Precipitation behaviors of primary phases in Al-Mg-Sc- Zr aIloy [J]. The Chinese Journal of Nonferrous Metals, 2010, 20(6): 1083-1087. (in Chinese)
[18] MARQUIES E A, SEIDMAN D N. Nanoscale structural evolution of Al3Sc precipitates in Al-Sc alloys [J]. Acta Materialia, 2001, 49(11): 1909-1919.
[19] ZHAO Wei-tao, YAN De-sheng, RONG Li-jian. TEM observation of annealing microstructure of deformed Al-Mg-Sc-Zr alloy [J]. Acta Metallurgica Sinica, 2005, 41(11l): 1150-1154. (in Chinese)
[20] JIANG Feng, WEN Kang, JIAN Hai-gen, JIANG Chun-li, ZHAO Juan, JIANG Long. Existing form and action mechanism of minor scandium and zirconium in Al-Cu-Mg alloy [J]. Journal of Central South University of Technology, 2010, 17(1): 19-23.
[21] IWAMUR S, NAKAYAMA M, MIURS Y. Coherency between Al3Sc precipitate and the matrix in Al alloys containing Sc [J]. Materials Science and Forum, 2002, 396(402): 1151-1156.
[22] TANG Bi-yu, LI Dong-lin, CHEN Ping, YI Jian-xiong, WEN Li, PENG Li-ming, DING Wen-jiang. The thermal properties of Al-Mg-TM (TM=Sc, Zr): Ab initio study [J]. Solid State Sciences, 2010, 12(5): 845-850.
(Edited by YANG Bing)
Foundation item: Project(2006AA03Z523) supported by the National High Technology Research and Development Program of China
Received date: 2011-05-17; Accepted date: 2011-07-22
Corresponding author: PAN Qing-lin, Professor, PhD; Tel: +86-731-88830933; E-mail: pql@csu.edu.cn
Abstract: An Al-5.8Mg-0.4Mn-0.35(Sc+Zr) (mass fraction, %) alloy sheet was prepared using water chilling copper mould ingot metallurgy processing which was protected by active flux. The influence of stabilizing annealing on mechanical properties and microstructure of the cold rolling sheet was studied. The results show that the strength and hardness of the alloy decrease, while the elongation increases with increasing the stabilizing annealing temperature. With the increase of stabilizing annealing time, the strength and hardness of the alloy drop slightly but its ductility exhibits no change. Partial recovery and recrystallization orderly occur with the increase of annealing temperature during stabilizing treatment. Only different degrees of recovery occur in the alloys annealed below 400 ℃ for 1 h. Partial recrystallization occurs after annealed at 450 ℃ for 1 h. By annealing at 300 ℃ for 1 h, the alloy can obtain the optimum application values of σb, σ0.2 and δ, which are 436 MPa, 327 MPa and 16.7%, respectively.
- Microstructure and mechanical properties of Al-5.8Mg-Mn-Sc-Zr alloy after annealing treatment
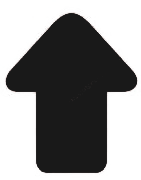