- Abstract:
- 1 Introduction▲
- 2 Experimental▲
- 3 Results and discussion▲
- 4 Conclusions▲
- References
- Figure
- Fig.1 XRD patterns of CuO/ZrO2 composite photocatalyst prepared via three different routes
- Fig.2 XRD patterns of CuO/ZrO2 composite photocatalysts calcined at different temperatures for 12 h
- Fig.3
- Fig.4 TG-DTA curves of precursor sample of CuO/ZrO2 dried gel
- Fig.5 Maximal amount of H2 evolution on CuO/ZrO2 composite photocatalysts obtained via solid state reaction in different molar ratios of CuO to ZrO2 (Reaction conditions: concentration of photocatalyst 1 g/L; concentration of oxalic acid 0.05 mol/L; volume of distilled water 600 mL)
- Fig.6 Effect of photocatalyst preparation method on H2 evolution activity (Reaction conditions: concentration of photocatalyst 1 g/L; concentration of oxalic acid 0.05 mol/L; volume of distilled water 600 mL)
- Fig.7 Effect of calcination temperature on H2 evolution activity over CuO/ZrO2 composite photocatalyst synthesized via sol-gel technique (Reaction conditions: concentration of photocatalyst 1 g/L; concentration of oxalic acid 0.05 mol/L; volume of distilled water 600 mL)
- Fig.8 Durability of as-obtained CuO/ZrO2 composite photocatalyst prepared by sol-gel technique (Reaction conditions: concentration of photocatalyst 1 g/L; initial concentration of oxalic acid 0.05 mol/L; volume of distilled water 600 mL; irradiation time 12 h)
J. Cent. South Univ. Technol. (2011) 18: 56-62
DOI: 10.1007/s11771-011-0658-x
Photocatalytic H2 evolution activity of CuO/ZrO2 composite catalyst under simulated sunlight irradiation
YAN Jian-hui(阎建辉)1, 2, YAO Mao-hai(姚茂海)1, 2, ZHANG Li(张丽)1, 2,
TANG You-gen(唐有根)1, 2, YANG Hai-hua(杨海华)1
1. Department of Chemistry and Chemical Engineering, Hunan Institute of Science and Technology,
Yueyang 414000, China;
2. College of Chemistry and Chemical Engineering, Central South University, Changsha 410083, China
? Central South University Press and Springer-Verlag Berlin Heidelberg 2011
Abstract:
Zirconia-supported CuO (CuO/ZrO2) composite photocatalysts were successfully synthesized via citric acid-assisted sol-gel technique. For comparison, CuO/ZrO2 materials were also prepared by solid state reaction and co-precipitation method. The as-prepared powders were characterized by X-ray diffractometry (XRD), transmission electron microscopy (TEM), and thermogravimetric-differential thermal analysis (TG-DTA). The photocatalytic activity of CuO/ZrO2 catalyst was investigated based on the H2 evolution from oxalic acid solution under simulated sunlight irradiation. The effects of molar ratio of CuO to ZrO2, preparation method, phase change with the calcination temperature and the durability on the photocatalytic activity of the photocatalyst were investigated in detail. It is found that the optimal activity of photocatalytic H2 evolution (2.41 mmol·h-1·g-1) can be obtained when CuO/ZrO2 composite photocatalyst is synthesized by sol-gel technique and the mole ratio of CuO to ZrO2 is 40%. The activity of copper oxide supported on monoclinic ZrO2 calcined at higher temperature is much higher than that on tetragonal ZrO2 calcined at lower temperature, and the best calcination temperature is 900 ?C.
Key words:
CuO/ZrO2; photocatalytic activity; hydrogen evolution; simulated sunlight;
1 Introduction
Because of the prominent advantages, such as clean, renewable and environment friendly, hydrogen has been considered to be an effective energy carrier in future. Owing to the fact that water and light are naturally abundant and inexhaustible, photocatalytic H2 production from water splitting using semiconductor photocatalysts under UV and/or visible light irradiation has become a hot topic after the report by HONDA and FUJISHIMA for photoelectrochemical water splitting using a TiO2 semiconductor electrode in 1972 [1]. Although TiO2 is stable, innocuous, environmentally friendly and abundant, and has low cost [2], a great obstacle still exists that the band gap of TiO2 is 3.2 eV and TiO2 only responses to UV light irradiation. However, the energy of the UV light just occupies about 5% of the total solar energy [3]. Hence, in the past three decades, a series of photocatalysts have been developed for water splitting under visible light irradiation, such as tantalite [4-5] and niobate photocatalysts [6-7], metal sulfide [8], solid- solution photocatalysts [9-10], and doped photocatalysts [11-12]. Nevertheless, the variety of visible light- induced photocatalysts is still limited. Under such a background, searching new photocatalysts with the ability to absorb visible light is a key factor to photocatalytic H2 production from water splitting.
Zirconia-supported CuO system has been demonstrated to have interesting catalytic properties in many reactions, such as water gas shift reaction [13], NO abatement reactions [14], CO/CO2 hydrogenation [15- 16], and generation of H2 from steam methane reforming [17-18]. However, there are few reports that CuO/ZrO2 catalyst can be applied for photocatalytic H2 production from water splitting under simulated sunlight irradiation. At present, in the aspect of the CuO composite photocatalysts, most of the investigations have been still focused on the composite of CuO/TiO2. BANDARA et al [19] investigated the photocatalytic H2 production activity of CuO/TiO2 from methanol solution under UV light irradiation. Also, JIN et al [20] reported CuO/TiO2 photocatalytic H2 generation eosin-sensitized under visible light irradiation. Nevertheless, the photocatalytic H2 evolution activity of CuO/TiO2 is still limited. For CuO/TiO2 composite photocatalyst, TiO2 plays an essential role for reaction, while CuO has a supportive role and H2 production reaction starts with the excitation of TiO2 particles. Compared with the CuO/TiO2 composite photocatalyst, in the system of CuO/ZrO2 composite photocatalyst, CuO plays an essential role for the reaction because the band gap of CuO is 1.7 eV [21]. Therefore, it not only is suitable for visible light-induced photocatalysis, but also generates more photo-excited electrons and holes than CuO/TiO2; while ZrO2 has a supportive role. ARDIZZONE and ORLANDI [22] indicated that ZrO2 exhibited excellent adsorption ability to organic compounds. In the present study, oxalic acid, a kind of organism, acted as sacrificial reagent and was adsorbed by ZrO2 in order to increase the separation of the photo-excited electrons and holes and improve the photocatalytic activity.
In this work, CuO/ZrO2 catalyst was synthesized via three approaches: solid state reaction, co-precipitation method, as well as sol-gel technique. More speci?cally, the photocatalytic activity of the as-prepared CuO/ZrO2 composite photocatalysts was investigated for photocatalytic H2 generation with oxalic acid as sacrificial reagent under simulated sunlight irradiation.
2 Experimental
2.1 Photocatalysts preparation
All the chemicals used in experiments were of analytical grade with no further treatment. The photocatalyst CuO/ZrO2 was synthesized by the following three approaches.
1) Solid state reaction. Mixture of CuO and ZrO2 with different molar ratios was milled for 30 min in an agate mortar, then calcined at 900 ?C for 12 h in air at a heating rate of 10 ?C/min and slowly cooled to room temperature. In the end, it was milled for 10 min again to get the final product.
2) Sol-gel technique. A stoichiometric quantity of Cu(NO3)2?3H2O, Zr(NO3)4?5H2O and anhydrous citric acid were dissolved together in 100 mL anhydrous ethanol to get a mixed solution. Then, the solution was stirred vigorously and heated on a hot plate set at 80 °C by using a water bath, until a transparent and polymeric gel was obtained. The as-prepared gel was subsequently transferred into an oven and kept at 120 °C to dry to form a powder precursor of CuO/ZrO2. The dried powder precursor was finally calcined at various temperatures (673-1 273 K) for 6 h in air.
3) Co-precipitation method. A stoichiometric quantity of Cu(NO3)2?3H2O and ZrOCl2?8H2O were dissolved together in 100 mL distilled water to produce a blue solution. Then, 1 mol/L NaHCO3 was added into the solution drop by drop under vigorous stirring until the pH became around 9-10, and viscous precipitates were produced. The precipitates were filtrated and washed by distilled water several times until no Cl- was detected, then dried at 120 °C overnight, and subsequently calcined at 900 °C for 6 h in air.
2.2 Photocatalysts characterization
The crystal structure of the as-prepared photocatalyst was identi?ed by powder X-ray diffraction method (XRD, Bruker D8) using Cu Kα radiation (λ=1.541 8 ?) with a scanning angle (2θ) of 10?-85? at a scan speed of 4 (?)/min, a voltage of 40 kV and a current of 300 mA. The grain size and morphology of the photocatalyst were characterized with a Hitachi H-800 transmission electron microscope (TEM) operated at 200 kV. The temperatures of possible decomposition and phase changes were determined with a thermogravimetric-differential thermal analyzer at a rate of 10 ?C/min from room temperature to 600 ?C.
2.3 Photocatalytic activity measurements
The photocatalytic activity of the as-prepared photocatalyst was evaluated by measuring the amount of H2 evolution from 0.05 mol/L oxalic acid solution under simulated sunlight irradiation. The H2 production experiment was carried out in an inner irradiation-type reaction vessel made of pyrex, and a Xe (250 W) lamp was used as the simulated sunlight source to offer photons. In all experiments, to keep the reaction temperature at room temperature, water was used as the external circulation cooling, electric fan and vacuum pump as the internal cooling. Prior to irradiation, 0.6 g of the as-prepared photocatalyst was dispersed with 100 mL distilled water in an ultrasonic bath and put in the reactor, subsequently the reactor was left in dark and nitrogen flux was introduced into the system for 30 min to remove O2. Afterwards, the photocatalytic reaction system was closed and exposed to the light irradiation. During the irradiation, the mixture was suspended by using a magnetic stirrer within the quartz cell. The as-produced H2 was collected in a water manometer and was analyzed by gas chromatography (TCD, N2 as gas carrier, zeolite NaX column). The volume of H2 was taken as the volume of the displaced water at different time intervals of irradiation. Some experiments were repeated three times and the results were reproducible within the experimental errors (±4%).
3 Results and discussion
3.1 Characterization
Fig.1 depicts the XRD patterns of CuO/ZrO2 composite photocatalyst prepared via solid state reaction, co-precipitation method, as well as sol-gel technique. All the samples were calcined at 900 ?C in air. It is obvious that the crystal structures of ZrO2-supporter prepared via different approaches all are composed of monoclinic zirconia (M-ZrO2). The diffraction intensities of sample prepared by sol-gel technique are lower than those of the sample prepared via solid state reaction. LI et al [23] consider this is probably due to the small particle size and/or the discrepancies in crystallinities.
Fig.1 XRD patterns of CuO/ZrO2 composite photocatalyst prepared via three different routes
Fig.2 presents the XRD patterns of the CuO/ZrO2 photocatalysts by sol-gel method calcined at different temperatures. At 400 ?C, the tetragonal zirconia (T-ZrO2) structure is dominant with a small amount of the monoclinic zirconia (M-ZrO2) and some amorphous phase. At 500 ?C and 600 ?C, the peak intensities of M-ZrO2, T-ZrO2 and copper oxide all get stronger, and the full-width at half-maximum (FWHM) of T-ZrO2 and copper oxide all become narrower. Whereas, after being calcined at 700 ?C, drastic crystallization of M-ZrO2 has occurred and M-ZrO2 is in a dominant position. When the calcination temperature is raised from 800 ?C to 1 000 ?C, the diffraction peaks of T-ZrO2 disappear, only M-ZrO2 structure exists, and stronger integral peaks intensity and narrower FWHM are observed, indicating
Fig.2 XRD patterns of CuO/ZrO2 composite photocatalysts calcined at different temperatures for 12 h
good crystallinity. Other studies have also indicated that the calcination temperature changes the crystal structure of ZrO2 [24]
Fig.3 presents TEM images of the CuO/ZrO2 photocatalyst fabricated via solid state reaction, co-precipitation method, as well as sol-gel technique. It can be seen that CuO and ZrO2 particles are successfully synthesized by any of three methods. The particle size of ZrO2-supporter synthesized by sol-gel technique is about 90 nm which is smaller than that prepared by co-precipitation method or solid state reaction. In addition, some particles prepared by solid state reaction
Fig.3 TEM images of CuO/ZrO2 composite photocatalysts synthesized via three different approaches: (a) Sol-gel technique; (b) Co-precipitation method; (c) Solid state reaction
are found to be aggregated badly.
Fig.4 shows the TG-DTA curves of the precursor sample of CuO/ZrO2 dried gel. From the thermo- gravimetric curve, there is three obvious mass losses in the temperature range of 30-350 ?C. The first mass loss before 200 ?C may be attributed to the release of H2O in the existence of physical absorption and the volatilization of organic solvent such as ethanol. The second mass loss at 200-300 ?C can be ascribed to the decomposition of the citric acid. The third mass loss at 300-350 ?C is due to the decomposition of nitrate and synthesis of the CuO/ZrO2 compounds. Meanwhile, the corresponding strong endothermic peak at about 340 ?C is observed from differential thermal curves.
Fig.4 TG-DTA curves of precursor sample of CuO/ZrO2 dried gel
3.2 Photocatalytic activity
3.2.1 Effect of molar ratio of CuO to ZrO2 on photo- catalytic H2 evolution activity
Fig.5 summarizes the rate of H2 evolution on CuO/ZrO2 composite photocatalysts containing different amounts of CuO obtained via solid state reaction. No H2 is detected when pure ZrO2 is used as photocatalyst, suggesting that ZrO2 is not active for photocatalytic H2 evolution under simulated sunlight irradiation. But after CuO is mixed with ZrO2, the activity for H2 evolution is increased greatly. It can be concluded that the composite of CuO with ZrO2 is necessary for photocatalytic H2 evolution under simulated sunlight irradiation. In the system of CuO/ZrO2 composite photocatalysts, H2 evolves on the CuO particles, and ZrO2 particles act as supporter to adsorb the reactive species such as sacrificial reagent so as to improve the separation of the photo-excited electrons and holes. Hence, with the increase of the molar ratio of CuO to ZrO2, the amount of H2 evolution on CuO/ZrO2 photocatalyst is further increased and achieves a maximum when the molar ratio of CuO to ZrO2 is 40%. However, if the molar ratio exceeds 40%, the quantity of ZrO2 will be decreased and influence the adsorption to the reactive species, which leads to the decrease of the consumption of the photo-generated holes, and further influences the separation of the photo-excited electrons and holes. Consequently, the photocatalytic activity is decreased. The appearance of a maximum activity with an optimum molar ratio of CuO to ZrO2 has also been observed for other preparation methods.
Fig.5 Maximal amount of H2 evolution on CuO/ZrO2 composite photocatalysts obtained via solid state reaction in different molar ratios of CuO to ZrO2 (Reaction conditions: concentration of photocatalyst 1 g/L; concentration of oxalic acid 0.05 mol/L; volume of distilled water 600 mL)
3.2.2 Effect of preparation method on photocatalytic H2 evolution activity
Fig.6 illustrates the effect of photocatalyst preparation method on the photocatalytic H2 evolution activity. It reveals that the CuO/ZrO2 composite photocatalysts synthesized by the sol-gel technique show the highest photocatalytic activity for H2 evolution. The TEM and XRD results demonstrate that the synthesis approach has great effects on the size and crystallinity of the product. The particle size and crystallinity strongly affect the photocatalytic activity for H2 evolution [25]. The higher the degree of crystallinity is, the smaller the amount of defects is. The defects operate as trapping and recombination centers between photoexcited electrons and holes, leading to a decrease in the photocatalytic activity. And the smaller the particle size is, the shorter the distance that photoexcited electrons and holes have to migrate to reaction sites on the surface become, resulting in a decrease in the recombination probability. The resultant photocatalytic activity is dominated by the balance among these factors.
In the present work, the grinding and calcination processes in the solid state reaction result in the inhomogeneous mixture, great mass change and bad dispersion of CuO particles on the surface of ZrO2-supporter, going against the photocatalytic activity. In the co-precipitation method, the homogeneity and dispersion are better than those of the samples prepared via solid state reaction, but the particle size and crystallinity of photocatalysts are compared unfavorably to the samples prepared via sol-gel technique. The sol-gel technique is superior to the other two preparation methods. Here, the using of citric acid as chelating agent, an important aspect of the sol-gel technique, ensures the homogeneity and dispersion of CuO on the surface of the ZrO2-supporter, the smaller size and higher crystallinity of the photocatalysts.
Fig.6 Effect of photocatalyst preparation method on H2 evolution activity (Reaction conditions: concentration of photocatalyst 1 g/L; concentration of oxalic acid 0.05 mol/L; volume of distilled water 600 mL)
3.2.3 Effect of calcination temperature on photocatalytic H2 evolution activity
Fig.7 reveals the H2 evolution activity of the CuO/ZrO2 composite photocatalyst synthesized via the sol-gel technique as a function of the calcination temperature. It is pointed out that, when the calcination temperature is increased from 400 ?C to 600 ?C, the amount of H2 evolution on the CuO/ZrO2 composite photocatalyst is not improved obviously. With further increasing the calcination temperature, however, the amount of H2 evolution is increased greatly, and reaches the maximum (2.41 mmol·h-1·g-1) at 900 ?C. As shown in the XRD results (Fig.2), with the change of the crystal structure from tetragonal at low temperature to monoclinic at high temperature, the photocatalytic activity is improved gradually, indicating that the photocatalytic activity of copper oxide supported on M-ZrO2 is much higher than that supported on T-ZrO2. The crystal structure of ZrO2-supporter has great effect on the activity of CuO/ZrO2 catalyst.
RHODES and BELL [26] demonstrated that the activity of copper supported on M-ZrO2 is considerably higher than that on T-ZrO2. This difference in activities has been attributed to the different adsorption capacities of both ZrO2 structures to adsorb reactive species. In this
Fig.7 Effect of calcination temperature on H2 evolution activity over CuO/ZrO2 composite photocatalyst synthesized via sol-gel technique (Reaction conditions: concentration of photocatalyst 1 g/L; concentration of oxalic acid 0.05 mol/L; volume of distilled water 600 mL)
experiment, oxalic acid has been used as sacrificial reagent in order to consume the photo-generated holes, and it exists in the form of HC2O4-. Therefore, when using M-ZrO2 as supporter, more reactive species of HC2O4- are adsorbed on the surface of CuO/ZrO2 composite photocatalyst than that of CuO supported on T-ZrO2, leading to quick consumption of the photo-excited holes. Accordingly, the photo-excited electrons and holes in CuO/M-ZrO2 can be separated more efficiently than those in CuO/T-ZrO2, which results in the higher photocatalytic activity of copper oxide supported on M-ZrO2 than that supported on T-ZrO2.
3.2.4 Durability of photocatalyst
The durability of the CuO/ZrO2 composite photocatalyst was studied by using the same catalyst for H2 evolution several times. Fig.8 depicts the amount of
Fig.8 Durability of as-obtained CuO/ZrO2 composite photocatalyst prepared by sol-gel technique (Reaction conditions: concentration of photocatalyst 1 g/L; initial concentration of oxalic acid 0.05 mol/L; volume of distilled water 600 mL; irradiation time 12 h)
H2 evolution with repeated use of the catalyst. After being irradiated for 12 h, the suspensions were kept in dark for 12 h, purged with N2 for 30 min and added with fresh oxalic acid, then irradiated again. In each run, the continuous consumption of oxalic acid and the adsorption of by-products on the photocatalyst surface result in slight decrease of photocatalytic activity. However, the addition of fresh oxalic acid in the second or third run restores photocatalytic activity. There is just a little decrease of the photocatalytic H2 evolution activity in repeated runs for 36 h. So, CuO/ZrO2 composite photocatalyst is considered to be a stable photocatalyst for photocatalytic H2 evolution under simulated sunlight irradiation.
4 Conclusions
1) The photocatalytic H2 evolution activity of CuO/ZrO2 catalyst is affected by the molar ratio of CuO to ZrO2. With increasing the molar ratio of CuO to ZrO2, the amount of H2 evolution on CuO/ZrO2 photocatalyst is increased and achieves a maximum when the molar ratio is 40%.
2) The CuO/ZrO2 photocatalysts synthesized by three different methods exhibit different photocatalytic H2 evolution activity. Sol-gel technique is superior to the other two preparation methods. Because of the introduction of the citric acid as chelating agent, the CuO/ZrO2 composite photocatalysts own the best homogeneity and dispersion, as well as the smaller size and higher crystallinity.
3) With increasing calcination temperature, the crystal structure of ZrO2-supporter is changed from T-ZrO2 at low calcination temperature to M-ZrO2 at high calcination temperature, and M-ZrO2 can adsorb more reactive species than T-ZrO2. Therefore, The photocatalytic H2 evolution activity of the CuO/ZrO2 composite photocatalyst is improved with increasing the calcination temperature, and achieves the maximal activity (2.41 mmol·h-1·g-1) at 900 ?C.
4) The CuO/ZrO2 catalyst is a stable photocatalyst for H2 evolution from aqueous oxalic acid solution under simulated sunlight irradiation. However, the band structure and the photocatalytic mechanism of the as-prepared CuO/ZrO2 composite photocatalyst are still unclear and further research needs to be performed.
References
[1] FUJISHIMA A, HONDA K. Electrochemical photolysis of water at a semiconductor electrode [J]. Nature, 1972, 238: 37-38.
[2] SI Shi-hui, YAN Chang-li, LIU Guo-cong, LIU Di, WANG Yue-long, WANG Xiao-guang. Controllable photodegradation of pesticide complex with nanocrystalline TiO2 photocatalyst [J]. Journal of Central South University: Science and Technology, 2004, 35(4): 591-594. (in Chinese)
[3] RAKESH R Y, XU H F. Incorporating strong polarity minerals of tourmaline with semiconductor titania to improve the photosplitting of water [J]. Journal of Physics Chemistry C, 2008, 112(2): 532-539.
[4] KATO H, KUDO A. New tantalate photocatalysts for water decomposition into H2 and O2 [J]. Chemical Physics Letters, 1998, 295: 487-492.
[5] KATO H, KUDO A. Photocatalytic water splitting into H2 and O2 over various tantalate photocatalysts [J]. Catalysis Today, 2003, 78: 561-569.
[6] OWEN C C, FRANK E O. Niobate nanosheets as catalysts for photochemical water splitting into hydrogen and hydrogen peroxide [J]. Journal of Physics Chemistry C, 2009, 113(1): 479-485.
[7] BEATA Z, EWA B P, RYSZARD J K. Preparation and characterization of lithium niobate as a novel photocatalyst in hydrogen generation [J]. Journal of Physics and Chemistry of Solids, 2008, 69: 236-242.
[8] ZONG Xu, YAN Hong-jian, WU Guo-peng, MA Gui-jun, WEN Fu-yu, WANG Lu, LI Can. Enhancement of photocatalytic H2 evolution on CdS by loading MoS2 as cocatalyst under visible light irradiation [J]. Journal of American Chemical Society, 2008, 130(23): 7176-7177.
[9] TSUJI I, KATO H, KOBAYASHI H, KUDO A. Photocatalytic H2 evolution reaction from aqueous solutions over band structure- controlled (AgIn)xZn2(1-x)S2 solid solution photocatalysts with visible-light response and their surface nanostructures [J]. Journal of American Chemical Society, 2004, 126(41): 13406-13413.
[10] LEE Y, TERAMURA K, HARA M, DOMEN K. Modification of (Zn1+xGe)(N2Ox) solid solution as a visible light driven photocatalyst for overall water splitting [J]. Chemistry of Materials, 2007, 19(8): 2120-2127.
[11] THAMMANOON S, SIRIPORN L, SUMAETH C. Use of Pt/N-doped mesoporous-assembled nanocrystalline TiO2 for photocatalytic H2 production under visible light irradiation [J]. Catalysis Communications, 2009, 10: 538-543.
[12] LUO Nian-jun, JIANG Zheng, SHI Hua-hong, CAO Fa-hai, XIAO Tian-cun, EDWARDS P P. Photo-catalytic conversion of oxygenated hydrocarbons to hydrogen over heteroatom-doped TiO2 catalysts [J]. International Journal of Hydrogen Energy, 2009, 34: 125-129.
[13] GONZALO A, SICHEM G, PAULO A. Influence of the crystalline structure of ZrO2 on the activity of Cu/ZrO2 catalysts on the water gas shift reaction [J]. Catalysis Communications, 2008, 9: 2550-2554.
[14] DANIELA P, ALESSANDRO M, DIANA S, MARIA C C, PAOLO C, VALERIO I. In situ sulphated CuOx/ZrO2 and CuOx/sulphated-ZrO2 as catalysts for the reduction of NOx with NH3 in the presence of excess O2 [J]. Applied Catalysis B: Environmental, 2005, 60: 83-92.
[15] MA Zhong-yi, YANG Cheng, WEI Wei, LI Wen-huai, SUN Yu-han. Catalytic performance of copper supported on zirconia polymorphs for CO hydrogenation [J]. Journal of Molecular Catalysis A: Chemical, 2005, 231: 75-81.
[16] LIU Jin-yao, SHI Jiang-liu, HE De-hua, ZHANG Qi-jian, WU Xiao-hui, LIANG Yu, ZHU Qi-ming. Surface active structure of ultra-fine Cu/ZrO2 catalysts used for the CO2 + H2 to methanol reaction [J]. Applied Catalysis A: General, 2001, 218: 113-119.
[17] YAO Cheng-zhang, WANG Lu-cun, LIU Yong-mei, WU Gui-sheng, CAO Yong, DAI Wei-lin, HE He-yong, FAN Kang-nian. Effect of preparation method on the hydrogen production from methanol steam reforming over binary Cu/ZrO2 catalysts [J]. Applied Catalysis A: General, 2006, 297: 151-158.
[18] SZIZYBALSKI A, GIRGSDIES F, GABIS A, WANG Y, NIEDERBERGER M, RESSLER T. In situ investigations of structure-activity relationships of a Cu/ZrO2 catalyst for the steam reforming of methanol [J]. Journal of Catalysis, 2005, 233: 297-307.
[19] BANDARA J, UDAWATTA C P K, RAJAPAKSE C S K. Highly stable CuO incorporated TiO2 catalyst for photocatalytic hydrogen production from H2O [J]. Photochemical & Photobiological Sciences, 2005, 4: 857-861.
[20] JIN Zhi-liang, ZHANG Xiao-jie, LI Yue-xiang, LI Shu-ben, LU Gong-xuan. 5.1% apparent quantum efficiency for stable hydrogen generation over eosin-sensitized CuO/TiO2 photocatalyst under visible light irradiation [J]. Catalysis Communications, 2007, 8: 1267-1273.
[21] XU Y, MARTIN A A S. The absolute energy positions of conduction and valence bands of selected semiconducting minerals [J]. American Mineralogist, 2000, 85: 543-556.
[22] ARDIZZONE S, ORLANDI M. Adsorption of alkyl benzenesulfonates at the ZrO2 solution interface [J]. Journal of Electroanalytical Chemistry, 1995, 391: 181-185.
[23] LI X K, KAKO T, YE J H. 2-propanol photodegradation over lead niobates under visible light irradiation [J]. Applied Catalysis A: General, 2007, 326: 1-7.
[24] VAHIDSHAD Y, ABDIZADEH H, BAHARVANDI H R, AKBARIBASERI M. Effects of calcinations temperature on the structure of CuO-ZrO2 nanoparticles [J]. International Journal of Modern Physics B, 2008, 22(18/19): 3201-3209.
[25] KUDO A, MISEKI Y. Heterogeneous photocatalyst materials for water splitting [J]. Chemical Society Reviews, 2009, 38: 253-278.
[26] RHODES M D, BELL A T. The effects of zirconia morphology on methanol synthesis from CO and H2 over Cu/ZrO2 catalysts (Part Ⅰ): Steady-state studies [J]. Journal of Catalysis, 2005, 233: 198-209.
Foundation item: Project(20876039) supported by the National Natural Science Foundation of China; Project(09JJ3023) supported by the Natural Science Foundation of Hunan Province, China
Received date: 2009-12-17; Accepted date: 2010-04-30
Corresponding author: YAN Jian-hui, Professor, PhD; Tel: +86-730-8640436; E-mail: yanjh58@163.com
- Photocatalytic H2 evolution activity of CuO/ZrO2 composite catalyst under simulated sunlight irradiation
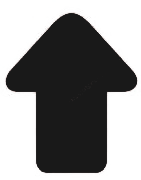