- Abstract:
- 1 Introduction▲
- 2 Department of Appl...▲
- 3 Results and discus...▲
- 4 Conclusions▲
- References
- Figure
- Fig. 1 Light microscopy image of an aqueous ethanol electrolyte including ZnCl2 and CH3(CH2)12COOH
- Fig. 2 SEM images of anodic surface under conditions of 0.1 mol/L myristic acid, 0.0487 mol/L ZnCl2 and applied DC voltage of 30 V with electrodeposition time of 10 min and different water contents in 150 mL electrolyte:
- Fig. 3 Variation rule of contact angle and deposition time of anodic surface under conditions of 0.1 mol/L myristic acid, 0.0487 mol/L ZnCl2 and applied DC voltage of 30 V with various contents of water in 150 mL electrolyte
- Fig. 4 SEM images of anodic surface under conditions of 0.1 mol/L myristic acid, 0.0487 mol/L ZnCl2, 10 mL water and applied DC voltage of 30 V with different electrodeposition time: (inset is corresponding image of water droplet and enlargement image)
- Fig. 5 Variation rule of contact angle and deposition time of anodic surface under conditions of 0.1 mol/L myristic acid, 0.0487 mol/L ZnCl2, 10 mL water and applied DC voltage of 30 V
- Fig. 6 SEM images of anodic surface under conditions of 0.1 mol/L myristic acid, 10 mL water, 20 min for DC voltage of 30 V with different concentrations of ZnCl2: (inset is corresponding enlargement image)
- Fig. 7 SEM images of cathodic surface under conditions of 0.1 mol/L myristic acid, 0.0487 mol/L ZnCl2, 10 mL water and applied DC voltage of 30 V with different electrodeposition time:(inset is corresponding image of water droplet and enlargement image)
- Fig. 8 Variation rule of contact angle and deposition time of cathodic surface under conditions of 0.1 mol/L myristic acid, 0.0487 mol/L ZnCl2, 10 mL water and applied DC voltage of 30 V
- Fig. 9 FTIR patterns of electrolyte including zinc chloride and ethanol, and powders scraped from anodic and cathodic superhydrophobic surfaces (0.1 mol/L myristic acid, 0.0487 mol/L ZnCl2, 10 mL water and applied DC voltage of 30 V for 20 min)
- Fig. 10 XRD spectra of anodic and cathodic powders corresponding to Fig. 9
J. Cent. South Univ. (2016) 23: 1576-1583
DOI: 10.1007/s11771-016-3211-0
A rapid one-step electrodeposition process for fabrication of superhydrobic surfaces on anode and cathode
HAO Li-mei(郝丽梅)1, YAN Xiao-le(闫小乐)1, XIE You(解忧)1, ZHANG Tao(张涛)1, CHEN Zhi(陈志)2
1. Department of Applied Physics, Xi’an University of Science & Technology, Xi’an 710054, China;
2 Department of Applied Physics, Northwestern Polytechnical University, Xi’an, 710129, China
Central South University Press and Springer-Verlag Berlin Heidelberg 2016
Abstract:
This work presents a method to solve the weak solubility of zinc chloride (ZnCl2) in the ethanol by adding some reasonable water into an ethanol electrolyte containing ZnCl2 and myristic acid (CH3(CH2)12COOH). A rapid one-step electrodeposition process was developed to fabricate anodic (2.5 min) and cathodic (40 s) superhydrophobic surfaces of copper substrate (contact angle more than 150°) in an aqueous ethanol electrolyte. Morphology, composition, chemical structure and superhydrophobicity of these superhydrophobic surfaces were investigated by SEM, FTIR, XRD, and contact angle measurement, respectively. The results indicate that water ratio of the electrolyte can reduce the required deposition time, superhydrophobic surface needs over 30 min with anhydrous electrolyte, while it needs only 2.5 min with electrolyte including 10 mL water, and the maximum contact angle of anodic surface is 166° and that of the cathodic surface is 168°. Two copper electrode surfaces have different reactions in the process of electrodeposition time, and the anodic copper surface covers copper myristate (Cu[CH3(CH2)12COO]2) and cupric chloride (CuCl); while, zinc myristate (Zn[CH3(CH2)12COO]2) and pure zinc (Zn) appear on the cathodic surface.
Key words:
one-step electrodeposition process; superhydrophobicity; contact angle; aqueous; anode; cathode;
1 Introduction
Wettability of materials surface is one of the most important properties, and it depends on both geometrical microstructures and chemical composition. Superhydrophobic surface has attracted much attention owing to its great importance not only in fundamental research but also in industrial application [1-2], including separation of oil/water dispersions, anti-snow, stain-resistant textiles, drag reduction, corrosion resistance, stain-resistant surfaces, etc.
To mimic the lotus leaf effect, enormous contributions have been made to design and fabricate the superhydrophobic surfaces by many methods including wet chemical etching, solution-immersion, sol–gel method, chemical vapor deposition, anodic oxidation, microfabrication, electrodeposition, selfassembly, layer-by-layer, plasma etching, and so on [3-6]. All reported methods emphasize the importance of the coexistence of both surface roughness and the low surface energy in order for the surface to exhibit superhydrophobicity. One-step method has made great progress, and it can simultaneously prepare both surface roughness and low surface energy in the surface. At present, one-step method for fabrication of superhydrophobic surface has much research. For example, XI et al [7] used one-step solution-immersion process and electrodeposition method to achieve superhydrophobic surface with flowerlike particles of copper stearate, and it was also proved that cheap fatty acid can fabricate superhydrophobic surface by simple one-step method. SALEEMA et al [8-10] did much research work following XI et al [7] by one-step method. Recently, articles appear about one-step methods, such as XU et al [11] using one-step solution-immersion process to fabricate copper foam superhydrophobic surface, CONTRERAS et al [12] using simple one-step dipping process to obtain superhydrophobic polypropylene nanocomposite coatings, and WATANABE and FUJIHARA [13] using one-step synthesis of layered yttrium hydroxides in immiscible liquid–liquid systems. Our group had also prepared various superhydrophobic cathodic surfaces with different salt chlorides (manganese chloride, nickel chloride, etc.) by fast one-step electroposition method [14-15]. Furthermore, they mostly focus on cathodic or anodic superhydrophobic surface in the previous preparation method. However, research on the synthesis of both anodic and cathodic superhydrophobic surfaces at one time by electrodeposition method is limited.
Since ZnCl2 has a weak solubility in the ethanol, and CH3(CH2)12COOH has a weak solubility in the water, a method was presented to solve solution question by adding some reasonable water into an ethanol electrolyte in this work. One-step electrodeposition method was developed to simultaneously prepare anodic and cathodic superhydrophobic surfaces with an aqueous ethanol electrolyte including ZnCl2 and CH3(CH2)12COOH. The effect of ratio of water and ethanol, electrodeposition time, concentration of zinc chloride on the structure and physical properties of anodic surface were investigated in detail. Furthermore, the effect of electrodeposition time on cathodic superhydrophobic surface was studied. In addition, the formation mechanisms of both the anodic and the cathodic surfaces were also discussed.
2 Experimental section
2.1 Surface preparation
In a typical synthesis [14], 0.1 mol/L myristic acid was dissolved in the mixture of water and ethanol to form 150 mL electrolyte, and the electrolytes with ZnCl2 concentration of 0.0047, 0.0247, 0.0487, 0.0978 and 0.1467 mol/L were used for the electrodeposition process, respectively. Figure 1 shows the light microscopy image of mixing uniform electrolyte. As shown, there exists a lot of suspended flocculence in the electrolyte. Two pieces of copper plates (25 mm×50 mm×15 mm) were abraded with silicon carbide papers (from 400 to 800 grades), washed with distilled water, and dried by electric blower under an atmosphere condition for the working electrodes. The as-prepared two copper plates were taken as the cathode and anode in an electrolyte cell, and a direct current (DC) voltage of 30 V was applied to two electrodes with a distance of 2 cm. After a certain electrolysis time, the working electrodes were rinsed thoroughly several times with ethanol and dried under an air condition. Consequently, both of the as-prepared anodic and cathodic surfaces were obtained. In separate experiments, the effects of different electrodeposition time were also studied. All chemicals were of analytical grade and without further purification. All solutions were prepared with distilled water.
Fig. 1 Light microscopy image of an aqueous ethanol electrolyte including ZnCl2 and CH3(CH2)12COOH
2.2 Characterization
The obtained samples were tested by field-emission scanning electron microscopy (FE-SEM, JSM-6390A). The crystalline phases were characterized by X-ray diffraction techniques (XRD, D/max2400) with monochromatic Cu Kα radiation. Infrared transmission spectrum was recorded at room temperature on a Fourier transform infrared spectrophotometer (FTIR, BRUKER- Tensor27). A contact angle analysis was performed with an optical CCD (CNB-GP300 CGG1) at ambient temperature. Rolling angles were measured by a self- made device. The average contact angle was determined by measuring the sample at five different positions. Water droplets of about 5 μL were dropped onto the superhydrophobic surface from a distance of 0.2 cm by a vibrating burette.
3 Results and discussion
3.1 Effect of ratio of water and ethanol mixture
Since zinc chloride has a low solubility in ethanol solution and myristic acid has a poor solubility in the water, this work presents one-step fast process by adding a small amount of water into ethanol to improve the low solubility. The effect of water content from 0 to 30 mL in 150 mL electrolyte was investigated on the superhydrophobic property of anodic surface.
Figure 2 shows the SEM image of anodic surface under conditions of 0.1 mol/L myristic acid,0.0487 mol/L ZnCl2 and applied voltage of 30 V with electrodeposition time of 10 min and different water contents in 150 mL electrolyte. When there is no water in the electrolyte, several microstructures with some natural flake appear on the anodic surface, and the fastest growth is along the arrow direction (see Fig. 2(a)), and the value of the contact angle is only 86° (see Fig. 3). When water of 5 mL is added to the electrolyte, the anisotropic growths of microstructures are weakened, and many flower-like structures occur along different direction, and its contact angle is increased to 155°. When water content increases to 10 mL, the flower becomes bigger and more and the contact angle improves to 160°.
Figure 3 shows the variation rule of contact angle and deposition time of anodic surface with different contents of water in 150 mL electrolyte. It can be seen from the Fig. 3, when electrodeposition time is less than 30 min, anodic surface is not hydrophobic in anhydrous electrolyte. When electrodeposition time is equal andmore than 30 min, its contact angle has only 151°. However, when the water of 5 mL is added to the electrolyte, the contact angle is significantly higher than that of anhydrous electrolyte. When the added water is further increased to 10 mL, anodic surface with only 2.5 min has the good contact angle of 152°, and its contact angle arrives at a relatively steady value of 166° with the increasing electrodeposition time, which is similar to the fact that the certain water in the electrolyte can promote the reaction [16]. When the added water increases to 20 mL, superhydrophobicity of the anodic surface can be obtained in 1 min. When the water of 30 mL is added, the anodic surface with 0.5 min can achieve superhydrophobicity. It is noted that with the electrodeposition time of greater than 30 min, the contact angle of the anodic surface with 20 mL or 30 mL water slowly decreased, which is different from that with the water content of 0, 5, or 10 mL. Therefore, a reasonable amount of water has an important effect on superhydrophobic properties of the anodic surface. Importantly, the anodic superhydrophobic surface needs a less electrodeposition time with increasing the water content. However, over the 30 mL water will affect the solubility of myristic acid in the electrolyte, and then more than 30 mL water was not studied in this work. So, a small amount of water can improve the low solubility of zinc chloride in ethanol and has no obvious effect on the solubility of myristic acid, and then improves superhydrophobicity of the anodic surface. In the later section, the water of 10 mL is fixed.
Fig. 2 SEM images of anodic surface under conditions of 0.1 mol/L myristic acid, 0.0487 mol/L ZnCl2 and applied DC voltage of 30 V with electrodeposition time of 10 min and different water contents in 150 mL electrolyte:
Fig. 3 Variation rule of contact angle and deposition time of anodic surface under conditions of 0.1 mol/L myristic acid, 0.0487 mol/L ZnCl2 and applied DC voltage of 30 V with various contents of water in 150 mL electrolyte
3.2 Effect of electrodeposition time
Figure 4 shows the surface morphology of anodic surface under conditions of 0.1 mol/L myristic acid, 0.0487 mol/L ZnCl2 and applied voltage of 30 V with the different electrodeposition time. As shown in Fig. 4(a), when electrodeposition time is 2.5 min, there are some random sheet structures on the substrates surface, but these sheets do not cover the entire surface and part of the surface is bare. When time increases to 3.5 min, the ordered sheet structures are observed on the surface, and their sizes become larger, and then they completely cover the surface.
As the time further enlarges to 5 min, some flower structures with an average diameter about 10-15 μm appear on the surface, and they are composed of these ordered sheets. However, the anodic surface is not completely covered by these flowers. When the time enhances to 10 min, these flowers structures cover the entire surface and the distance of flowers is 1-2 μm. When the deposition time extends to 20 min, the regular flower becomes denser, and it has an average diameter of 15-20 μm. The inset of Fig. 4(e) is the corresponding photo of water drop on the surface, and the anodic surface shows an excellent superhydrophobicity property. When the time prolongs to 60 min, three bloomy flowers appear with diameter of about 25 μm above the first layer of flower, and the flower with double layer enhances the dimension and roughness. When the time evolves to 90 min, there exists the bigger flower, and the density of flowers becomes weak. Furthermore, a high magnification SEM images of Figs. 4(h) and (i) reveal that the flower structures with 60 min have a more significant uniform and higher dense than that with 90 min. The main reason is that the anodic copper involves oxidation-reduction reaction at the electro- depositing process, and the amount of the new reaction product in this copper increases with evolution of time; So, it is hard for the later product to tightly adhere to the anodic surface, which makes the part coatings drop off from the surface, and the structures become worse.
Fig. 4 SEM images of anodic surface under conditions of 0.1 mol/L myristic acid, 0.0487 mol/L ZnCl2, 10 mL water and applied DC voltage of 30 V with different electrodeposition time: (inset is corresponding image of water droplet and enlargement image)
Figure 5 shows the detailed variation rule of contact angle and deposition time corresponding to curve of 10 mL in Fig. 3. As shown, the contact angle of anodic surface is about 60° when the electrodeposition is 1 min. However, when the time is increased to 2.5 min, the contact angle improves to 152°, which results from some random sheet structures on the anodic surface (see Fig. 4(a)), and these hierarchical structures significantly increase the surface roughness. When the electro- deposition time is further increased, the contact angle gradually increases. When time increases to 10 min, the anodic surface has a good contact angle of 160° and a rolling angle of less than 2°. This is because the flower structures completely cover the surface. While the electrodeposition time is further prolonged, the contact angle is slowly increased, and the value with 60 min improves to 166°, which is greater than 161.3° in Ref. [7] and 162° in Ref. [9]. However, when time is 90 min, the contact angle decreased slightly since the part of the coatings falls off from the anodic surface.
Fig. 5 Variation rule of contact angle and deposition time of anodic surface under conditions of 0.1 mol/L myristic acid, 0.0487 mol/L ZnCl2, 10 mL water and applied DC voltage of 30 V
3.3 Effect of ZnCl2 concentration
Effect of the concentration of zinc chloride on the morphology and wettability of the surface was also studied in detail.
Figure 6 depicts SEM images of anodic surface with different concentrations of ZnCl2, 0.1 mol/L myristic acid, and 20 min for 30 V electrodeposition voltage. When the concentration of zinc chloride is 0.0047 mol/L, several flowers with the diameter of 5-10 μm are observed on the anodic surface, and the contact angle is about 158°. When the concentration of zinc chloride is increased to 0.0247 mol/L, the flower becomes bigger and more, and the corresponding contact angle improves to 160°. With the concentration of zinc chloride increases, reaction product increases and roughness improves slowly, and so the contact angle slightly is enhanced. When the concentration further enhances to 0.0978 mol/L, the morphology of the surface is almost no change, and the contact angle still keeps about 160°. This is because zinc chloride has reached saturation point in a certain content of myristic acid, and so it has no obvious effect on the contact angle of anodic surface. However, when the concentration of zinc chloride is greater than 0.1467 mol/L, it is difficult to study its influence on the morphology and wettability due to its bad solubility in the aqueous ethanol electrolyte.
3.4 Effect of electrodeposition time on cathodic surface
In Ref. [7-15], the anodic or cathodic superhydrophobic surface was prepared by various methods. However, there is only limited published literature [21] concerning about fabrication of anodic and cathodic superhydrophobic coatings at one time. Thus, superhydrophobicity of the cathodic surface corresponding to anodic surface in section 3.2 was also investigated.
Figure 7 shows SEM images of the cathodic surfaces for different electrodeposition time under the same condition of the corresponding anodic surface. As shown in Fig. 7, when the deposition time is 2.5 min, another flower-like structure, different from the flower on the anodic surface, is entirely covered on the cathodic surface. It can be found that the size of flower is bigger than that of the corresponding anodic surface, and there exist several larger flowers with the diameter of about 50 μm above these dense flower structures. With increasing the deposition time, the number of the big flowers becomes more, and the small flowers gradually disappear. The reason is mainly that the big flower is dominant according with the Ostwald competition mechanism [17]. Furthermore, it can be seen from the inset of Fig. 7(b) that the bigger flower has the smaller secondary branches in the trunk, and then the flower results in an isotropic growth. When the deposition time prolongs to 60 min, only one flower is observed from Fig. 7(d). Furthermore, the secondary branches and the third branches appear in this flower, which will greatly increase the roughness of the cathode surface.
Fig. 6 SEM images of anodic surface under conditions of 0.1 mol/L myristic acid, 10 mL water, 20 min for DC voltage of 30 V with different concentrations of ZnCl2: (inset is corresponding enlargement image)
Fig. 7 SEM images of cathodic surface under conditions of 0.1 mol/L myristic acid, 0.0487 mol/L ZnCl2, 10 mL water and applied DC voltage of 30 V with different electrodeposition time:(inset is corresponding image of water droplet and enlargement image)
Figure 8 depicts the water contact angles of the cathodic surfaces with different deposition time. The cathodic surface with only 40 s has the good contact angle of 151°. With increasing the time, the contact angle increases, and the value with 20 min improves to 168°, which is shorter than 1 h in Ref. [7] and has a similar rule with the improving roughness. However, when the time is more than 20 min, the superhydrophobicity is slightly weakened. It mainly results from the too thicker coating (1-2 cm) in the cathodic surface with the more deposition time, so that water drop can be easily trapped into the rough structures.
Fig. 8 Variation rule of contact angle and deposition time of cathodic surface under conditions of 0.1 mol/L myristic acid, 0.0487 mol/L ZnCl2, 10 mL water and applied DC voltage of 30 V
3.5 Mechanic formation
Figure 9 shows FTIR patterns of an aqueous ethanol electrolyte including ZnCl2 and CH3(CH2)12COOH, and the powders scraped from the anodic and cathodic superhydrophobic surfaces at 0.0487 mol/L ZnCl2 in 0.1 mol/L myristic acid solution for 20 min. It can be seen from curve “1” that there are the adsorption peaks at 2916 cm-1 and 2849 cm-1 corresponding to the asymmetric and symmetric —CH stretching vibrations in the high-frequency region, respectively, and the adsorption peaks at 1699 cm-1 in the low-frequency region, which is different from the myristic acid of 1701 cm-1 [7, 14]. It can be deduced that the uniform stirred electrolyte may generate new complex ingredient. Furthermore, when the electrodeposition process is over, two adsorption peaks at 2916 cm-1 and 2849 cm-1 in the high-frequency region still exist, but the peak of 1699 cm-1 in the low-frequency region becomes 1585 cm-1 and 1442 cm-1 of the anodic surface and 1540 cm-1 and 1462 cm-1 of the cathodic surface corresponding to the symmetric and asymmetric COO— stretching vibrations, respectively. It is indicated to generate salt myristate [7, 18-20] in both of anodic and cathodic surfaces, and myristic acid had reacted completely with ethanol and the solutions products did not include any of free myristic acid.
Fig. 9 FTIR patterns of electrolyte including zinc chloride and ethanol, and powders scraped from anodic and cathodic superhydrophobic surfaces (0.1 mol/L myristic acid, 0.0487 mol/L ZnCl2, 10 mL water and applied DC voltage of 30 V for 20 min)
Furthermore, Fig. 10 depicts the corresponding XRD spectra of the powders in Fig. 9. It is found that there are five defined diffraction peaks with “*” in the small angle region of 1.5°-20°, which reveals that the as-prepared anodic surface includes copper myristate. Whereas, six diffraction peaks 111, 200, 220, 311, 400 and 331 in the high angle region of 20°-80° are indexed to cupric chloride (CuCl) (JCPDS card No.06-0344). Therefore, we can deduce that there is copper myristate (Cu[CH3(CH2)12COO]2) with low surface energy and cupric chloride (CuCl) on the anodic copper surface. Similar, it is found that there are three well defined diffraction peaks with “%” in the small angle region of less than 20°, which reveals that the as-prepared cathodic surface includes zinc myristate. Whereas, four diffraction peaks 002, 100, 101, 102 in the big angle region of30°-80° are indexed to pure zinc (Zn) (JCPDS card No.01-1238). Therefore, we can deduce that the flower structure with multi-level branch is composed of zinc myristate (Zn[CH3(CH2)12COO]2) and pure zinc (Zn).
Fig. 10 XRD spectra of anodic and cathodic powders corresponding to Fig. 9
Based on the XRD and FTIR technique, the reaction equations [7, 21] of the anodic surface can be written as follows:
Cu-2e→Cu2+ (1)
Cu2++2CH3(CH2)12COOH→Cu[CH3(CH2)12COO] 2+2H+ (2)
Cu+Cl-→CuCl+e (3)
The reaction equations of cathodic surface are described as follows.
Zn2++2e→Zn (4)
Zn2++2CH3(CH2)12COOH→Zn[CH3(CH2)12COO]2+2H+ (5)
2H++2e→H2 (6)
The reaction mechanism is explained that the anodic copper involves in reaction at the electrodepositing process. The growth of copper myristate crystals from aqueous ethanol electrolyte is controlled by nucleation and growth process. These processes are affected by the precursor concentration and the applied potentials. While, the pure zinc was rapidly deposited on the cathodic copper substrate at the beginning of electrodeposition process, and then the high flux of Zn nucleus provides a good basis for zinc myristate with flower-like structures. Furthermore, it was observed that hydrogen was gradually released around the cathode surface during the reaction process.
4 Conclusions
A method was developed to solve a low solution of ZnCl2 in the ethanol and has a low solution of CH3(CH2)12COOH in the water by adding some reasonable water into an ethanol electrolyte. One-step electrodeposition method was presented to simultaneously prepare both anodic and cathodic superhydrophobic surfaces with this novel aqueous ethanol electrolyte including ZnCl2 and CH3(CH2)12COOH. A water ratio of the electrolyte has a great effect on superhydrophobic property of the anodic surface, and water can reduce the required deposition time. Anodic and cathodic superhydrophobic surfaces of copper substrate were fabricated with 2.5 min and 40 s by the rapid one-step electrodeposition method, respectively. With an electrodeposition time increases, the superhydrophobic surfaces improve, and the maximum contact angle of anodic surface is 166°, and that of the cathodic surface is 168°. Two copper electrode surfaces have different reactions in the process of electrodeposition time, and the anodic copper surface covers copper myristate (Cu[CH3(CH2)12COO]2) and cupric chloride (CuCl); while, zinc myristate (Zn[CH3(CH2)12COO]2) and pure zinc (Zn) appear on the cathodic surface.
References
[1] Barthlott W, Neinhuis C. Purity of the sacred lotus, or escape from contamination in biological surfaces [J]. Planta, 1997, 202(1): 1-8.
[2] Celia E, DARMANIN T, GIVENCHY E T D, AmigonI S, Guittard F. Recent advances in designing superhydrophobic surfaces [J]. Journal of Colloid and Interface Science, 2013, 402: 1-18.
[3] Liu Ke-song, Tian Ye, Jiang Lei. Bio-inspired superoleophobic and smart materials: Design, fabrication, and application [J]. Progress inMaterialsScience, 2013, 58(4): 503-564.
[4] Sun J Y, Bhushan B. Structure and mechanical properties of beetle wings: A review [J]. RSC Advances, 2012, 2(33): 12606- 12623.
[5] Zhu H, Guo Z, Liu W. Adhesion behaviors on superhydrophobic surfaces [J]. Chemical Communications, 2014, 50(30): 3900-3913.
[6] Papadopoulos P, Mammen L, Deng X, Vollmer D, Butt H J. How superhydrophobicity breaks down [J]. Proceedings of the National Academy of Sciences, 2013, 110: 3254-3258.
[7] XI Jin-ming, LIN Feng, JIANG Lei. A general approach for fabrication of superhydrophobic and superamphiphobic surfaces [J]. Applied Physics Letters, 2008, 92(5): 053102.
[8] Saleema N, Sarkar D K, Gallant D, Paynter R W, Chen X G. Chemical nature of superhydrophobic aluminum alloy surfaces produced via a one-step process using fluoroalkyl-silane in a base medium [J]. ACS Applied Materials & Interfaces, 2011, 3(12): 4775-4781.
[9] Sarkar D K, Saleema N. One-step fabrication process of superhydrophobic green coatings [J]. Surface and Coatings Technology, 2010, 204(15): 2483-2486.
[10] Huang Y, Sarkar D K, Gallant D, Chen X. Corrosion resistance properties of superhydrophobic copper surfaces fabricated by one-step electrochemical modification process [J]. Applied Surface Science, 2013, 282: 689-694.
[11] Xu Jia, XU Jin-liang, Cao Yang, Ji Xian-bing, Yan Yu-ying. Fabrication of non-flaking, superhydrophobic surfaces using a one-step solution-immersion process on copper foams [J]. Applied Surface Science, 2013, 286: 220-227.
[12] Contreras C B, Chagas G, Strumia M C, Weibel D E. Permanent superhydrophobic polypropylene nanocomposite coatings by a simple one-step dipping process [J]. Applied Surface Science, 2014, 307: 234-240.
[13] Watanabe M, Fujihara S. One-step synthesis of layered yttrium hydroxides in immiscible liquid–liquid systems: Intercalation of sterically-bulky hydrophobic organic anions and doping of europium ions [J]. Journal of Solid State Chemistry, 2014, 210(1): 130-137.
[14] Chen Zhi, Hao Li-mei, Chen An-qi, Song Qing-jun, Chen Chang-le. A rapid one-step process for fabrication of superhydrophobic surface by electrodeposition method [J]. Electrochimica Acta, 2012, 59: 168-171.
[15] Chen Zhi, Li Feng, Hao Li-mei, Chen An-qi, Kong You-chao. One-step electrodeposition process to fabricate cathodic superhydrophobic surface [J]. Applied Surface Science, 2011, 258: 1395-1398.
[16] Merte L R, Peng G, Bechstein R, Rieboldt F, Farberow C A, Grabow L C, Besenbacher F. Water-mediated proton hopping on an iron oxide surface [J]. Science, 2012, 336(6083): 889-893.
[17] Lim B, Xia Y. Metal nanocrystals with highly branched morphologies [J]. Angewandte Chemie-International Edition, 2011, 50(1): 76-85.
[18] Liang S H, Yu T, Liu D P, Wang W X, Wang Y P, Han X F. Characterization of stearic acid adsorption on Ni (111) surface by experimental and first-principles study approach [J]. Journal of Applied Physics, 2011, 109(7): 07C115.
[19] Wang Ming, Li Hai-xia, Huang Xing-liang, Yi Li. Zinc maleate and calcium stearate as a complex thermal stabilizer for poly (vinyl chloride) [J]. Journal of Vinyl & Additive Technology, 2014, 20(1): 1-9.
[20] Mukherjee S, Datta A. Langmuir-Blodgett deposition selects carboxylate headgroup coordination [J]. Physical Review E, 2011, 84(4): 041601.
[21] Chen Zhi, Hao Li-mei, Chen Chang-le. Simultaneous fabrication of superhydrophobic coatings on cathodic and anodic copper surfaces with micro/nano-structures [J]. ECS Electrochemistry Letters, 2012, 1(4): D21-D23.
(Edited by YANG Hua)
Foundation item: Projects(11304243, 11102164) supported by the National Natural Science Foundation of China; Project(2014JQ1039) supported by the Natural Science Foundation of Shannxi Province, China; Project(12JK0966) supported by the Shaanxi Provincial Education Department, China; Project(2013QDJ037) supported by the Xi’an University of Science and Technology Dr Scientific Research Fund, China; Project(3102016ZY027) supported by the Fundamental Research Funds for the Central Universities of China; Project(13GH014602) supported by the Program of New Staff and Research Area Project of NPU, China
Received date: 2015-04-28; Accepted date: 2015-06-25
Corresponding author: CHEN Zhi, Associate Professor, PhD; Tel: +86-29-88431664; E-mail: c2002z@nwpu.edu.cn
Abstract: This work presents a method to solve the weak solubility of zinc chloride (ZnCl2) in the ethanol by adding some reasonable water into an ethanol electrolyte containing ZnCl2 and myristic acid (CH3(CH2)12COOH). A rapid one-step electrodeposition process was developed to fabricate anodic (2.5 min) and cathodic (40 s) superhydrophobic surfaces of copper substrate (contact angle more than 150°) in an aqueous ethanol electrolyte. Morphology, composition, chemical structure and superhydrophobicity of these superhydrophobic surfaces were investigated by SEM, FTIR, XRD, and contact angle measurement, respectively. The results indicate that water ratio of the electrolyte can reduce the required deposition time, superhydrophobic surface needs over 30 min with anhydrous electrolyte, while it needs only 2.5 min with electrolyte including 10 mL water, and the maximum contact angle of anodic surface is 166° and that of the cathodic surface is 168°. Two copper electrode surfaces have different reactions in the process of electrodeposition time, and the anodic copper surface covers copper myristate (Cu[CH3(CH2)12COO]2) and cupric chloride (CuCl); while, zinc myristate (Zn[CH3(CH2)12COO]2) and pure zinc (Zn) appear on the cathodic surface.
- A rapid one-step electrodeposition process for fabrication of superhydrobic surfaces on anode and cathode
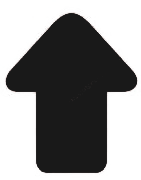