- Abstract:
- 1 Introduction▲
- 2 Materials and methods▲
- 3 Results▲
- 4 Discussion▲
- References
- Figure
- Fig.1 CD spectra of samples treated in microwave irradiation and water bath for 10 min
- Fig.2 CD spectra of samples treated in microwave irradiation and water bath for 20 min
- Fig.3 CD spectra of samples treated in microwave irradiation and water bath for 30 min
- Fig.4 CD spectra of samples treated in microwave irradiation and water bath for 40 min
- Fig.5 CD spectra of samples treated in microwave irradiation and water bath for 50 min
- Fig.6 CD spectra of samples treated in microwave irradiation and water bath for 60 min
- Fig.7 Varying contents of regular α-helix (Hr)
- Fig.8 Varying contents of distorted α-helix (Hd)
- Fig.9 Varying contents of regular β-strand (Sr)
- Fig.10 Varying contents of distorted β-strand (Sd)
- Fig.11 Varying contents of β-turn (T)
- Fig.12 Varying contents of random coil
J. Cent. South Univ. Technol. (2011) 18: 1029-1033
DOI: 10.1007/s11771-011-0799-y
Effect of microwave irradiation on secondary structure of α-amylase by circular dichroism
ZHANG Xiao-yun(张小云)1, 2, QIN Wen-qing(覃文庆)1, TIAN Xue-da(田学达)2, HUANG Meng(黄猛)2
1. School of Mineral Processing and Bioengineering, Central South University, Changsha 410083, China;
2. College of Chemical Engineering, Xiangtan University, Xiangtan 411105, China
? Central South University Press and Springer-Verlag Berlin Heidelberg 2011
Abstract:
Based on the starch hydrolysis reaction accelerated by microwave irradiation with α-amylase, the circular dichroism (CD) and secondary structure changes of α-amylase under the condition of microwave irradiation and water bath were studied by circular dichroism spectra. The results showed that, both the peak heights (at λ=193 nm) of the CD spectra of the samples treated by microwave irradiation and water bath reduced. The reduced rate by microwave irradiation ranged from 140% to 220%, while the reduced rate by water bath ranged from 60% to 140%. The peak of the sample treated by microwave irradiation for 60 min disappeared at λ=193 nm, while the sample showed a wake peak by water bath. The peak position by microwave irradiation emerged a blue shift in the range of 5-8 nm at λ=204 nm and λ=220 nm, while it emerged in the range of 3-5 nm by water bath. With time going on, the microwave irradiation and water bath have prompted the secondary structure of α-helix, β-sheet, β-turn and the mutual transformations of random coil, but the trends were different.
Key words:
microwave irradiation; α-amylase; secondary structure; circular dichroism spectra;
1 Introduction
Alpha-amylase, i.e. 1,4-α-D-glucosidase, can hydrolyze the α-1,4-glycosidic linkages in the starch to quickly liquefy the starch to produce low-molecular- mass dextrin and reduce sugar. Widely used in various industries including glucose production, beer brewing, and alcohol, fermentation and textile industries, α- amylase is one of the most active study areas in the field of enzyme research [1-4]. Our preliminary study showed that, compared with water bath, microwave irradiation can accelerate the hydrolysis of corn starch catalyzed by α-amylase [5-6]. Relevant studies [7-9] indicated that microwave irradiation improves the “microenvironment” of enzyme, so as to enhance the catalytic specificity of enzyme. Microwave irradiation enhances the orientation effect between the spatial structure of active sites and relevant substrate groups in enzyme catalytic system, and results in a closer distance and strict orientation between the reactive groups in substrate molecules and active center of enzyme, leading to higher efficiency and specificity of enzyme catalytic reaction.
Within the ultraviolet region (190-240 nm) of circular dichroism (CD), the main chromophoric groups are peptide chains. As the CD spectrum within the ultraviolet region contains the conformational information on main chain of biopolymers, it is the most effective method for studying the secondary structure of protein solution [10-15]. In this work, the circular dichroism spectra were used to study the influence of microwave irradiation and water bath on the secondary structure of α-amylase to provide theoretical support for the catalytic mechanism of α-amylase in the starch hydrolysis reaction.
2 Materials and methods
2.1 Materials
Alpha-amylase, bought in Japan, Product No. DA 4251, and enzyme activity of 4 000 U/mg was used.
XH-10 microwave reactor, supplied by Beijing Xianghao Tech. Co., Ltd., was temperature controllable.
KW series thermometer was supplied by Shanghai Bierde Industrial Co., Ltd.
JASCO-815 circular dichroism spectroscopy was supplied by Zhejiang University of Technology, China.
2.2 Sample preparation
Accurately weigh 2 g of α-amylase, dissolve it in distilled water, transfer it to a 1 000 mL volumetric flask and dilute it to obtain 2 mg/mL α-amylase solution (non-buffer system). Measure 6 aliquots of 50 mL α-amylase solution. The microwave power was set at 800 W and irradiation temperature was set at 62 °C. Incubate α-amylase solution in the microwave reactor for 10, 20, 30, 40, 50 and 60 min, respectively, and then place the samples into 2 °C refrigerator before measurement. Pipet 6 aliquots of 50 mL α-amylase solution, and incubate in 62 °C water bath for 10, 20, 30, 40, 50 and 60 min, respectively, then place the samples into 2 °C refrigerator before measurement.
2.3 Determination of CD spectra
Before determination of CD spectra, dilute samples at a rate of 1:4 and 0.5 cm quartz cell were used for CD spectra determination. Experimental conditions were room temperature (25 °C), scanning wavelength 190- 250 nm, determination sensitivity 5×10-3 (°)/cm, slit width 10 nm, and scanning rate 100 nm/min. Average molecular mass of amino acid residue is 109, and the mean value of three scanning values was taken as the result of CD data. Circular dichroism is represented by mean residue ellipticity [θ]. After CD spectra had been obtained, Selcon3 program of CDpro [13] package was used to calculate the variation of the content of secondary structure in α-amylase within the wavelength range from 190 to 250 nm under microwave irradiation and water bath.
3 Results
3.1 Influence of microwave irradiation and water bath on CD spectra of α-amylase
The characteristic absorption bands of the secondary structures of protein within the range from 190 to 250 nm are listed in Table 1 [11]. The CD spectra of α-amylase treated with microwave irradiation and water bath are shown in Figs.1-6. The untreated α-amylase shows a positive absorption peak at the wavelength of 193 nm, and double negative absorption peaks at the wavelengths of 204 nm and 220 nm, which are characteristics of the α-helical secondary structure. The negative peak at 204 nm represents π – π* transition of peptide bonds in α-helical structure, and the negative peak at 220 nm derives from n – π* transition [16]. The CD spectra ranging from 205 nm to 225 nm are the result of superposition of α-helical with β-sheet structures. The weak positive peak of β-sheet offsets the indent between double negative peaks of α-helix to form a wide negative peak [17].
Compared with the untreated samples, the microwave irradiation and water bath caused different changes in peak height and peak position on CD spectra. As shown in Figs.1-6, at wavelength λ=193 nm, all peak heights of the treated group decrease. The microwave irradiation causes the decrease of 140%-220% and the greatest decrease is observed at 60 min of microwave treatment. The water bath results in the decrease of 60%-140% and the greatest decrease is also observed at 60 min of water bath. As shown in Fig.6, 60 min of microwave irradiation results in the disappearance of the spectrum peak at wavelength λ=193 nm, while a weak peak presents at the same wavelength for 60 min treatment of water bath.
Table 1 Characteristic absorption of secondary structure
Fig.1 CD spectra of samples treated in microwave irradiation and water bath for 10 min
Fig.2 CD spectra of samples treated in microwave irradiation and water bath for 20 min
Fig.3 CD spectra of samples treated in microwave irradiation and water bath for 30 min
Fig.4 CD spectra of samples treated in microwave irradiation and water bath for 40 min
Fig.5 CD spectra of samples treated in microwave irradiation and water bath for 50 min
As shown in Figs.1-6, blue shifts of double negative peaks at λ=204 nm and λ=220 nm are observed for all treated groups. The microwave irradiation causes a blue shift ranging from 5 to 8 nm, while the water bath results in a blue shift ranging from 3 to 5 nm. The microwave irradiation causes larger blue shift than the water bath does. Under the microwave irradiation, the shape of double negative peaks gradually transit to a single negative peak as the treatment time is increased.
Fig.6 CD spectra of samples treated in microwave irradiation and water bath for 60 min
3.2 Influence of microwave irradiation and water bath on secondary structure of α-amylase
After determination of sample CD spectra (190- 250 nm), Selcon3 program of CDpro package was used to calculate the content (mass fraction) of secondary structures after different treatment times of microwave irradiation and water bath, as shown in Figs.7-12.
Fig.7 Varying contents of regular α-helix (Hr)
As shown in Fig.7, under the microwave irradiation and water bath, the content of regular α-helix gradually decreases with the increase of treatment time. The microwave irradiation results in faster decrease rate than the water bath does. Under the microwave irradiation, the decrease range is 36.29%-77.42% with the maximum drop at 60 min of microwave treatment. Under the water bath, the decrease range is 8.47%-28.23% with the maximum drop at 50 min of water bath. As shown in Fig.8, under the microwave irradiation, the content of non-regular α-helix also drops with the increase of treatment time, while the water bath causes slight increase in content. The microwave irradiation results in a decrease ranging from 0 to 38.86% with the maximum drop at 60 min. The water bath causes increase in content of non-regular α-helix, ranging from 9.33% to 12.44% and the maximum increase is observed at 10 min of treatment.
Fig.8 Varying contents of distorted α-helix (Hd)
Fig.9 Varying contents of regular β-strand (Sr)
Fig.10 Varying contents of distorted β-strand (Sd)
As shown in Figs.9 and 10, regular and non-regularβ-sheets present similar change modes in content under the two treatment methods. Under the microwave irradiation, the content increases with time; while under the water bath, it decreases with time. During the microwave treatment, the content of regular β-sheet increases by 133.33%-480% with the maximum increase at 50 min; the content of non-regular β-sheet increases by 4.84%-35.48% with the maximum increase at 50 min. During the water bath treatment, the content of regular β-sheet decreases by 46.67%-186.67% with the maximum drop at 30 min; the content of non-regular β-sheet decreases by 35.48%-54.84% with the maximum drop at 60 min.
Fig.11 Varying contents of β-turn (T)
Fig.12 Varying contents of random coil
As shown in Figs.11 and 12, β-turn and non-regular coil also present similar change mode in content under the two treatment methods. Under the microwave irradiation and water bath, the contents of β-turn and non-regular coil increase with time. During the microwave irradiation, the content increase of β-turn ranges from 15.53% to 29.61% and the maximum growth is observed at 50 min; the content increase of non-regular coil ranges from 20.21% to 47.74% and the maximum growth is observed at 60 min. During the water bath treatment, the content of β-turn increases by 6.31%-13.11% with the maximum growth at 60 min; the content of non-regular turn increases by 7.67%-24.04% with the maximum growth at 50 min.
4 Discussion
Preliminary studies [18-20] on the influence of microwave irradiation on the structure of enzyme protein show that, the microwave irradiation can change the secondary structure of protein, and result in the transition of ordered structure to disordered structure, so as to influence the activity of enzyme protein.
This study shows that, microwave irradiation and water bath present different influences on the content change of secondary structure of α-amylase. The influence of water bath on the secondary structure of α-amylase is only a result of the thermal effect. However, the microwave irradiation can produce thermal effect and other effects on the secondary structure of α-amylase. Under the microwave irradiation, the α-helix content of α-amylase decreases sharply, while the contents of β-sheet, β-turn and non-regular coil increase gradually. The microwave irradiation results in disordered secondary structure of α-amylase, similar to the result reported by VUKOVA et al [20]. The secondary structures of enzyme protein are maintained by the hydrogen bonds between carbonyl and amide groups on the peptide. Hydrogen bond is one of the main forces that stabilize the secondary structure of protein in addition to hydrophobic bond and van der Waals force. The results suggest that the microwave irradiation can affect the hydrogen bond (including hydrogen bond between peptide chains and between peptide chain and water molecule), hydrophobic bond and van der Waals force, leading to loosening, breakage and restructuring of hydrogen bond to change the orientation of the original hydrogen bonds that maintain the stable secondary structure of α-amylase protein. As a result, the hydrogen bonds are redistributed to change the conformation and activity of enzyme protein, so as to decrease the structure stability of α-amylase and increase its flexibility. This study provides theoretical support for the reaction mechanism of microwave irradiation in accelerating the starch hydrolysis catalyzed by α-amylase.
References
[1] MOU Ke-jia, WANG Zheng-xiang. The progress on starch-binding domain of amylases [J]. Science and Technology of Food Industry, 2007, 28(3): 242-245.
[2] SUN Lian-hai. Study on the relation of the function and α-amylase structure [D]. Henan: Henan Agricultural University, 2006: 5-10. (in Chinese)
[3] MARCO J L, BATAUS L A, VALENCIA F F. Purification and characterization of a truncated Bacillus subtilis α-amylase produced by Escherichia coli [J]. Applied Microbiology and Biotechnology, 1996, 44(6): 746-752.
[4] ROBERT T C, KIRSTEN M V, LU Y C. Salivary α-amylase as a measure of endogenous adrenergic activity [J]. Clinical Physiology and Functional Imaging, 1996, 16(4): 433-488.
[5] ZHANG Xiao-yun, TIAN Xue-da, CHEN Hua-rong. A preparation method of starch hydrolysate by microwave induced catalysis. CN: ZL200610136955.5 [P]. 2008-10-22.
[6] LIU Hong, ZHANG Xiao-yun, ZENG Mei-xia. Kinetics of corn starch hydrolysis by enzyme catalysis under microwave irradiation [J]. Fine Chemicals, 2010, 27(1): 82-86.
[7] HAO Bo-lin, LIU Ji-xing. Theoretics physics and life science [M]. Shanghai: Shanghai Science and Technology Press, 1997. (in Chinese)
[8] KARMEE S K. Application of microwave irradiation in biocatalysis [J]. Research Journal of Biotechnology, 2006, 1(2): 1-4.
[9] CHEN Xiao-bing, LI Li, WANG Zhao. Progress on research and application in microwave catalysis [J]. Journal of Liaoning University of Petroleum & Chemical Technology, 2006, 26(4): 15-17. (in Chinese)
[10] YAO Zhan-quan, AODUN G, XU Qiang. Study on the effect of electric field on the secondary structure of α-amylase by circular dichroism [J]. Journal of Instrumental Analysis, 2006, 26(12): 2311-2314. (in Chinese)
[11] HUANG Han-chang, JIANG Zhao-feng, ZHU Hong-ji. The methods of protein conformation predicted by uv-circular dichroism [J]. Chemistry, 2007, (7): 501-506. (in Chinese)
[12] WHITMORE L, WALLACE B A. Protein secondary structure analyses from circular dichroism spectroscopy: Methods and reference databases [J]. Biopolymers, 2007, 89(5): 392-400.
[13] SREERAMA N, VENYAMINOV SY, WOODY R W. Estimation of protein secondary structure from circular dichroism spectra: inclusion of denatured proteinswith native proteins in the analysis [J]. Analytical Biochemistry, 2000, 287: 243-251.
[14] SREERAMA N, WOODY R W. Estimation of protein secondary structure from circular dichroism spectra: Comparison of CONTIN, SELCON, and CDSSTR methods with an expanded reference set [J]. Analytical Biochemistry, 2000, 287(2): 252-260.
[15] JOHNSON W C. Protein secondary structure and circular dichroism: A practical guide [J]. Proteins: Structure, Function, and Bioinformatics, 1989, 7(3): 205-214.
[16] FENG Yong-jun, ZHANG Chang-kai, CHEN Ya-li. Circular dichroism spectra and secondary structures in solution of flammulin [J]. Acta Chimica Sinica, 2000, (7): 816-820. (in Chinese)
[17] OOBATAKE M, TAKAHASHI S, TATSUO O. Conformational stability of ribonuclease T1 [J]. Biochem, 1979, 86(1): 55-63.
[18] XIA Yong-mei, SUN Shi-yu, FANG Yun. Microwave irradiation- enzyme coupling catalysis [J]. Process in Chemistry, 2007, 19(2/3): 250-255. (in Chinese)
[19] PROCELLI M, CACCIAPUOTI G, FUSCO S. Non-thermal effects of microwaves on proteins: Thermophilic enzymes as model system [J]. FEBS Letters, 1997, 402(2/3): 102-106.
[20] VUKOVA T, ATANASSOV A, IVANOV R. Intensity-dependent effects of microwave electromagnetic fields on acetylcholinesterase activity and protein conformation in frog skeletal muscles [J]. Medical Science Monitor, 2005, 11(2): 50-56.
(Edited by PENG Chao-qun)
Foundation item: Project(08A080) supported by the Scientific Research Fund of Hunan Provincial Education Department, China
Received date: 2010-04-30; Accepted date: 2010-12-08
Corresponding author: TIAN Xue-da, Professor, PhD; Tel: +86-731-58292469; E-mail: snowy@xtu.edu.cn
- Effect of microwave irradiation on secondary structure of α-amylase by circular dichroism
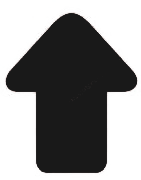