J. Cent. South Univ. Technol. (2008) 15: 768-773
DOI: 10.1007/s11771-008-0142-4
Preparation of fluorescence starch-nanoparticle and
its application as plant transgenic vehicle
LIU Jun(刘 俊)1, 2, WANG Feng-hua(王凤华)2, WANG Ling-ling(王玲玲)3, XIAO Su-yao(肖苏尧)2,
TONG Chun-yi(童春意)2, TANG Dong-ying(唐冬英)2, LIU Xuan-ming(刘选眀)2
(1. College of Materials Science and Engineering, Hunan University, Changsha 410082, China;
2. College of Life Science and Biotechnology, Hunan University, Changsha 410082, China;
3. School of Physics and Microelectronics Science, Hunan University, Changsha 410082, China)
Abstract:
Starch-nanoparticles were synthesized in water-in-oil microemusion at room temperature, and the starch-nanoparticles were coated with poly-L-lysine. The surface of the starch-nanoparticles was combined with fluorescence material Ru(bpy)32+?6H2O, and then the particles were characterized via transmission electron microscope. The fluorescence nanoparticles were conjugated with plasmid DNA to form complexes, and then treated with ultrasound and
Key words:
fluorescence nanoparticle; plant; gene vehicle; ultrasound-mediation; transformation;
1 Introduction
Nanoparticle biomaterial has been applied to genetic transformation of mammalian cells[1-3]. Carried by nanoparticles, exterior genes penetrate mammalian cells usually by two ways: one way is that target group on nanoparticles combines with the specific receptor of cell membrane surface, and the receptor cells endocytose the complex, and then DNA escapes from the composites to cytoplasm and nucleus[4-5]; the other way is that the receptor cells randomly uptake DNA composites without target groups on nanoparticles [6-7]. As the plant cell wall is not the same as the mammalian cell membrane which can absorb DNA composite by intracellular endocytosis, nanoparticle gene vehicle has not been applied to plant genetic transformation. Nowadays, two major methods are applied to plant genetic transformation: microprojectile bombardment and agrobacterium. Microprojectile bombardment yields low transformation efficiency (mostly 2%-20%) with high cost[8-9]. Agrobacterium transformation is mostly applied to dicotyledon. When it is used in few monocotyledon plants, the efficiency gets much lower (the transformation efficiency is 0.01%-20%), for it is hard for the monocotyledon to produce much more impressible cells to accept exogenous genes[10-11]. In this work, nanoparticle biomaterial was designed to transport exterior genes through plant cell wall with the aid of ultrasound because ultrasonic wave could induce instantaneous channel in cell wall, cell membrane and nucleus membrane[12-13].
2 Experimental
2.1 Preparation of fluorescence nanoparticle
Starch-nanoparticles (StNPs) were synthesized in water-in-oil microemusion[14-16], suspended in the solution of phosphoric acid buffer (pH=7.4), and then poly-L-lysine and Ru(bpy)32+?6H2O (RuBPY) solution were added. The mixed solution was shaken at room temperature for 6 h and then centrifuged. The deposition was freeze-dried.
2.2 Transfer of nanoparticle into cell mediated by ultrasound
The calli of Dioscrea Zigiberensis G H Wright were chosen to mortar and ground to cell suspension. The suspension was added into tubes of 1 mL and placed for 5 min. The residue deposited while separated cells were in the superstratum. 950 μL of the top suspension was sucked and dripped into five Eppendorf tubes averagely, and then 50 μL of nanoparticle solution was added. The solution was completely mixed under mini- pulsator, and then the tubes were put into the water in ultrasonic generators (three types of 120 W-40 kHz, 180 W-40 kHz and 300 W-40 kHz, Shanghai SCIENTZ Research Institute of Biotechnology, China) and treated with ultrasound for 3, 5, 7, 9 and 11 min, respectively. The cells were washed with Murashige-Skoog (MS) liquid culture medium and centrifuged to remove the nonspecifically attached nanoparticles on the cells. The cell suspension was dipped on slides for observing the trace of nanoparticles after the cells were cultured for 12, 24, 48 and 72 h, respectively. The cells incubated with the same nanoparticles that were not disposed with ultrasound were treated as controls.
2.3 Analysis of nanoparticle combined with DNA
R1601 and pEGAD plasmid DNA were dripped into nanoparticle solution at the same time, mixed for 1 min on mini-pulsator, placed under room temperature for 30 min, then dotted in the gel poles, and finally electrophoresed for 1 h at 80 V. Photos were taken with UVPCDS 8000PC white/ultrsviolet transilluminator. To analyze whether RuBPY-PLL-StNPs could protect DNA from ultrasound damage, the similar method was used[17]. Nanoparticles were mixed with pEGAD plasmid DNA, and then treated with ultrasound for 5, 10 and 15 min, respectively. The naked pEGAD plasmid DNA was treated as control. To analyze whether RuBPY-PLL- StNPs could protect DNA from DNase I cleavage, the ultrasoundated complexes were incubated with 1 U DNase I at 37 ℃ for 1 h[18], and finally digested with Trypsin and dotted in the gel well. The naked pEGAD plasmid DNA was treated as control.
2.4 Transformation of nanoparticle gene vehicle
Firstly, gene vectors were constructed. Five tubes of 500 μL were prepared. 10 μL nanoparticle solution of 1 μg/μL was dripped in each tube, then 1, 3, 5, 7 and 9 μL pEGAD plasmid DNA of 0.864 μg/μL were added respectively and mixed for 1 min on mini-pulsator. All the tubes were placed at room temperature for 30 min, and different vectors carrying diverse copies of genes were constructed. Secondly, susceptive cells were prepared. The calli of Dioscrea Zigiberensis G H Wright were cultured on MS solid medium with 2 mg/L of 2,4-D for a week. Those calli growing vigorously were selected to mortar and ground to cells suspension, and then the suspension was transferred to MS liquid medium with 2 mg/L of 2,4-D and 0.5 mg/L of 6-BA, cultivated at 28 ℃ and 110 r/min under dim light. A week later, most of the suspension cells divided rapidly. Thirdly, the appropriate intensity and charged time of ultrasound were selected. Based on the former research of RuBPY-PLL-StNPs trafficing the cells of Dioscrea Zigiberensis G H Wright, the mixed solution of 1 mL cell and nanoparticle was treated with ultrasound 120 W, 40 kHz for 5 min. At last, gene transformation was accomplished. The cell suspension was diverted to high penetrability MS medium with 0.75 mol/L sugar, and 4 h later, it was centrifuged at 1 000 r/min for 1 min to pour out the superstratum, and then gene vehicle solution was mixed with the cells to form hypotonic solution. After being mixed for 5 min on mini-pulsator, the suspension was treated with ultrasound for 5 min. The treated cell suspension was placed for 30 min before the mixture was centrifuged for 1 min, and then the cells were cultivated in MS liquid medium with 2 mg/L of 2,4-D and 0.5 mg/L of 6-BA. The similar cells incubating with nanoparticle gene vehicles and without treating with ultrasound acted as controls.
3 Results and discussion
RuBPY-PLL-StNPs with positive charge and diameter of 50-100 nm were synthesized (Fig.1). When observed under fluorescence microscope, those nanoparticles exhibit intense fluorescence (Fig.2). Therefore, those RuBPY-modified nanoparticles are visible with eyes, while those without labeling molecules of RuBPY are not imaged under fluorescence microscope. So the distribution and movement of those nanoparticles in cells will be traced if they are transferred into cells.
Fig.1 Atomic force microscope (AFM) image of nanoparticles (50±10 nm)
Fig.2 Image of nanoparticles under inverted fluorescence microscope
When treated with ultrasound under the condition of 180 W-40 kHz and 300 W-40 kHz for 3 min, 90% of the cells are broken. While under the condition of 120 W-40 kHz for 3-5 min, the cells keep normal shape and RuBPY-PLL-StNPs are observed in the cells, the nanoparticles not only locate in cytoplasm, but also enter into nucleus (Fig.3). When treated with ultrasound for 7-11 min, more than 90% of the cells are broken, and at the same time, more nanoparticles are visible in those broken cells. But no nanoparticles can be observed in the controlled cells. The results show that nanoparticles can pierce the cell wall, cell membrane and even cell nucleus membrane when they are mediated by ultrasound; varying the treated time and the intensity of ultrasound can control the number of the entered nanoparticles and affect the viability of the cells. When the treated suspension cells are cultured for 24-72 h, the fluorescence intensity decreases in 90% of those cells embodying fluorescence nanoparticles while the other cells embodying the unmodified nanoparticles emit unchanged fluorescence. This indicates that most cells keep biological activity; the nanoparticles are distributed to offspring during the cells division; at the same time, parts of the nanoparticles are degraded by enzymes in the cells.
Fig.3 Transverse section images under confocal laser microscope: (a) Fluorescence image; (b) Optical image; (c) Overlay of (a) and (b)
The above results show that these kinds of nanoparticles can be transferred into the cells of Dioscrea Zigiberensis G H Wright mediated by ultrasound, and DNA conjugated on these nanoparticles may also be transited into the cells. Therefore, this kind of nanoparticles can be used as plant transgenic vehicles if they can protect DNA from ultrasound damage and DNase I cleavage.
As shown in Fig.4, when the nanoparticles combine with DNA to form complexes and are dotted in the poles, the complexes resort in poles (Fig.4, Lane 4), and no DNA stains can be observed. This demonstrates that those nanoparticles can combine with DNA efficiently. At the same time, the DNA copies can be adjusted by adding diverse amount of DNA. The complexes are digested with Trypsin to degrade poly-L-lysine and release
Fig.4 Electrophoresis of DNA-nanoparticle complexes: Lane 1—DNA control; Lane 2—R1601 plasmid DNA; Lane 3—pEGAD plasmid DNA; Lane 4—R1601 and pEGAD plasmid DNA-PLL-StNP complexes; Lane 5—Complexes as lane 4 digested with Trypsin; Lanes 6–8—pEGAD plasmid DNA-PLL-StNP complexes charged with ultrasound for 5, 10 and 15 min, respectively; Lane 9—Naked pEGAD plasmid DNA charged with ultrasound for 5 min (dotted sample after other lanes electrophoresed for 20 min); Lane 10— Untrasoundated complexes as lane 6–8 digested with Trypsin; Lane 11—Untrasoundated complexes digested with DNase I; Lane 12—Complexes as lane 11 digested with Trypsin; Lane 13—Naked pEGAD plasmid DNA digested with DNaseI
R1601 and pEGAD plasmid DNA (Fig.4, lane 5), which suggests that the nanoparticles can integrate different genes (DNA) at the same time.
When the DNA-nanoparticle complexes were treated with ultrasound, digested with Trypsin, and then dotted in the gel poles. The DNA bands (Fig.4, lane 10) keep the same as those of naked pEGAD plasmid (Fig.4, lane 3). The fact that the DNA bands remain unchanged illustrates that the property of DNA does not change. While the naked pEGAD plasmid DNA, after being disposed by the ultrasound, leaves no band in the gel but a cluster of shining points dragging tails in the front of the lane(Fig.4, lane 9). All these facts imply that even though the naked DNA is broken up by the ultrasound, nanoparticles can effectively protect DNA from the destruction of the ultrasound.
Fig.4 also shows that the DNA-nanoparticle complexes are still left in the gel poles after being digested by DNase I (Fig.4, lane 11). The bands of the DNA released from nanoparticles (Fig.4, lane 12) are the same as those of the normal pEGAD plasmid. Meanwhile, no bands of naked DNA leave in the gel lane after being digested by DNase I. The results demonstrate that DNA-nanoparticle complexes can protect DNA not only from ultrasound damage, but also from DNase I cleavage.
The reason may be explained as follows: in the process of the combination of DNA with the nanoparticles, the surface effect of the latter and small-size effect may change the pattern of DNA and merge with nanoparticles into holistic one, without any spatial sites for the ultrasound or DNase I to touch. The above fact provides a theoretical base for the genetic transformation of nanoparticles using the ultrasound as the medium.
As illustrated in Fig.5, 24 h later, parts of the transformed cells exhibit intensive green fluorescence when they are observed under inverted fluorescence microscope; 48 h later, the fluorescence intensity decreases; 72 h later, few cells emit intensive fluorescence all the time. While the controlled cells show dim background fluorescence. The results demonstrate that the pEGAD GFP genes are transferred into the cells of Dioscrea Zigiberensis G H Wright and express GFP enjoying a frequency over 5%. The change of the fluorescence intensity suggests that the most exterior genes transitorily express in cytoplasm except few exterior genes integrate into nucleus genome and express GFP genes invariably. In a word, nanoparticles can be used as plant transgenic vehicles.
Fig.5 Transverse section images under confocal laser microscope: (a) Fluorescence image; (b) Fluorescence image showing rudimental undegraded nanoparticles; (c) Overlay of (a) and (b)
There are a few major factors affecting the transfor- mation efficiency of nanoparticles as plant transgenic vehicles. The ultrasound should be taken into account at first. If the intensity is too powerful or the cells are disposed for long time, most of the plant cells will break up; on the contrary, faint and short-time ultrasound will pierce few instantaneous channels on the cell wall, cell membrane and nucleus membrane, which is not helpful for the introduction of exterior genes by the nanoparticle gene carriers. In conclusion, the strength and the time of the disposed ultrasound should change with specific plant cells and the number of cells. The DNA copy on the nanoparticle gene carrier is the second factor. If a nanoparticle gene vehicle carries too many gene copies, the probability of exterior genes inserting the same location of the plant genome will increase, and the silence chance of those genes will also increase; by contraries, the probability of exterior genes integrating the plant cells genome will decrease. However, this issue should be lucubrated. The third important factor is the state of responsive cells. It is found that the cells which are disposed in high penetrability condition can accept exterior genes easily because the osmosis helps nanoparticle gene vehicles to diffuse through the instantaneous channels produced by ultrasound. Appropriate concentration of cytokinin will stimulate more susceptive cells, as those cells cultivated in the MS medium with 2 mg/L 2,4-D and 0.5 mg/L 6-BA express GFP with higher efficiency than those in the medium with 2 mg/L 2,4-D. The reason may be that more cells are in state of vigorous division after being stimulated with cytokinin, and during the division cycle, nucleus membrane breaks up, exposing more locations in the chromosome for exterior genes to insert. In addition, the shape and length of DNA (gene) affect the cell’s transformation. Two types of DNA fragments are prepared: one is the DNA extracted directly from plasmid, including circular fragments, super-circular fragments and linear fragments; the other is the linear segment recycled from the plasmid DNA digested by restricted enzyme EcoR1471 and EcoRI, which contains 35 S promoter and GFP gene. The two types of DNA band with composites respectively, and then are transferred into suspended cells mediated by ultrasound. The pEGAD plasmid is approximately 12 kb, while the linear DNA fragment cut by the double enzymes is about 2 kb. The experiment reveals that the transformation efficiency of the linear DNA is higher than that of circular DNA. The possible reason is that the shorter linear segments can combine with the plant cells’ genome more easily with a better expression while the steric effect caused by the circular or super-circular DNA segments reduces the integrating.
4 Conclusions
1) Fluorescence starch-nanoparticles are synthesized in water-in-oil microemusion. DNA is effectively protected from ultrasound damage by nanoparticle, while the naked DNA is destroyed in the same condition. Furthermore, the DNA-nanoparticle complexes treated with ultrasound can also protect DNA from the digestion of DNase I.
2) Nanoparticles are successfully used as plant transgenic vehicle. After being mediated by ultrasound, this vector availably overcomes the difficulty that exterior genes can hardly pass through the cell wall.
3) Nanoparticle gene vehicles are not restricted by the types of cells. The vehicles mediated by ultrasound transfer exogenous genes to insert cells’ genome, directly reducing the interim processes made by Agrobacterium. So they will be applied in all sorts of plants to get higher transfer efficiency. At the same time, this new method can omit elaborative processes such as eliminating bacterium.
4) Fluorescence starch-nanoparticle can not only carry genes (DNA) but also be imaged under fluorescence microscope, so the course of exterior genes’ movement can be investigated by observing the nanoparticles’ trace and the expressed GFP.
5) Those methods which can inspire instantaneous channels in the cell wall, cell membrane and even nucleolus membrane such as electroporation and laser impulsion, will also aid nanoparticle gene vehicles to enter plant cells as ultrasound does. Nanoparticle gene vehicles can be widely applied in suspension cells, tender buds, vigorous calli, developing embryos, pollen tubes and other tissues.
References
[1] Boussif O, Lezoualc'h F, ZANTA M A, Mergny M D, Scherman D, Demeneix B, Behr J. A versatile vector for gene and oligonucleotide transfer into cells in culture and in vivo: Polyethylenimine [J]. Proceedings of the National Academy Sciences USA, 1995, 92(16): 7297-7301.
[2] Luo D, Saltzman W M. Synthetic DNA delivery systems [J]. Nature Biotechnology, 2000, 18(1): 33-37.
[3] Roy I, Ohulchanskyy T Y, Bharali D J, Pudavar H E, Mistretta R A, Kaur N, Prasad P N. Optical tracking of organically modified silica nanoparticles as DNA carriers: A nonviral, nanomedicine approach for gene delivery [J]. Proceedings of the National Academy Sciences USA, 2005, 102(2): 279-284.
[4] HOOD J D, Bednarski M, Frausto R, Guccione S, Reisfeld R A, Xiang R, Cheresh D A. Tumor regression by targeted gene delivery to the neovasculature [J]. Science, 2002, 296(5577): 2404-2407.
[5] Thomas M, Klibanov A M. Conjugation to gold nanoparticles enhances polyethylenimine’s transfer of plasmid DNA into mammalian cells [J]. Proceedings of the National Academy Sciences USA, 2003, 100(16): 9138-9143.
[6] Chowdhury E H, Kunou M, Nagaoka M, Kundu A K, Hoshiba T, Akaike T. High-efficiency gene delivery for expression in mammalian cells by nanoprecipitates of Ca-Mg phosphate [J]. Gene, 2004, 341: 77-82.
[7] LIU Jun, LIU Xuan-ming, XIAO Su-yao, TONG Chun-yi, TANG Dong-ying, ZHAO Li-jian. Starch nanoparticle as transgenic vehicle mediated by ultrasound [J]. Chemical Journal of Chinese Universities, 2005, 26(4): 634-637. (in Chinese)
[8] Taylor N J, Fauquet C M. Microparticle bombardment as a tool in plant science and agricultural biotechnology [J]. DNA and Cell Biology, 2002, 21(12): 963-977.
[9] Men S, Ming X, Wang Y, Liu R, Wei C, Li Y. Genetic transformation of two species of orchid by biolistic bombardment [J]. Plant Cell Reports, 2003, 21(6): 592-598.
[10] Gelvin S B. Agrobacterium-mediated plant transformation: The biology behind the “Gene-Jockeying” tool [J]. Microbiology and Molecular Biology Reviews, 2003, 67(1): 16-37.
[11] Stachel S E, Messens E, Montagu M V, Zambryski P M, Zambryski P. Identification of the signal molecules produced by wounded plant cells that activate T-DNA transfer in Agrobacterium tumefaciens [J]. Nature, 1985, 318(6047): 624-629.
[12] Miller D L, Thomas R M. Ultrasonic gas body activation in Elodea leaves and the mechanical index [J]. Ultrasound in Medicine and Biology, 1993, 19(4): 343-351.
[13] Deng C X, Sieling F, Pan H, Cui J. Ultrasound-induced cell membrane porosity[J]. Ultrasound in Medicine and Biology, 2004, 30(4): 519-526.
[14] CHAI Li-yuan, SU Wei-feng, PENG Bing, WANG Yun-yan, WEI Shun-wen, YUAN Chun, YU Yan-fen, HAO Yun. Modification of nano-TiO2 by Al2O3 in-situ coating [J]. Journal of Central South University of Technology, 2006, 13(1): 17-21.
[15] XIAO Su-yao, LIU Xuan-ming, TONG Chun-yi, LIU Jun, TANG Dong-ying, ZHAO Li-jian. Studies of poly-L-lysine-starch nanoparticle preparation and its application as gene carrier [J]. Science in China Series B: Chemistry, 2005, 48(2): 162-166.
[16] YU Dan-mi, XIAO Su-yao, TONG Chun-yi, CHIN Lin, LIU Xuan-ming. Dialdehyde starch nanoparticles: Preparation and application in drug carrier [J]. Chinese Science Bulletin, 2007, 52(21):2913-2918.
[17] LIU Jun, LIU Xuan-ming, XIAO Su-yao, TONG Chun-yi, TANG Dong-ying, ZHAO Li-jian. Bioconjugated nanoparticle for DNA protection from ultrasound damage [J]. Analytical Sciences, 2005, 21(3): 193-195.
[18] HE Xiao-xiao, WANG Ke-min, TAN Wei-hong, LIU Bin, LIN Xia, HE Chun-mei, LI Du, HUANG Sha-sheng, LI Jun. Bioconjugated nanoparticles for DNA protection from cleavage [J]. Journal of the American Chemical Society, 2003, 125(24): 7168-7269.
Foundation item: Project(200501) supported the “985” Program of China
Received date: 2008-06-08; Accepted date: 2008-07-11
Corresponding author: LIU Xuan-ming, Professor; Tel: +86-731-8821721; E-mail: sw_xml@hnu.cn
(Edited by CHEN Wei-ping)
Abstract: Starch-nanoparticles were synthesized in water-in-oil microemusion at room temperature, and the starch-nanoparticles were coated with poly-L-lysine. The surface of the starch-nanoparticles was combined with fluorescence material Ru(bpy)32+?6H2O, and then the particles were characterized via transmission electron microscope. The fluorescence nanoparticles were conjugated with plasmid DNA to form complexes, and then treated with ultrasound and
- Preparation of fluorescence starch-nanoparticle andits application as plant transgenic vehicle
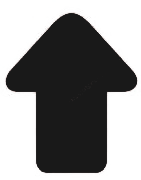