- Abstract:
- 1 Introduction▲
- 2 Experimental▲
- 3 Results and discussion▲
- 4 Conclusions▲
- References
- Figure
- Fig.1 SEM images of spherical Ni(OH)2 particles at different reaction times
- Fig.2 TEM image of Ni(OH)2 particle at 60 h
- Fig.3 XRD patterns for samples obtained at different reaction time
- Fig.4 Schematic illustration of template growth mechanism for spherical Ni(OH)2 particle
- Fig.5 Typical network microstructures of spherical Ni(OH)2 particle
- Fig.6 Formation processes of network microstructure for spherical Ni(OH)2 particle
J. Cent. South Univ. Technol. (2007)03-0310-05
DOI: 10.1007/s11771-007-0061-9
Template growth mechanism of spherical Ni(OH)2
PENG Mei-xun(彭美勋)1,2, SHEN Xiang-qian(沈湘黔)2,3
(1. School of Geoscience and Environmental Engineering, Central South University, Changsha 410083, China;
2. Changsha Research Institute of Mineral and Metallurgy, Changsha 410012, China;
3. College of Materials Science and Engineering, Jiangsu University, Zhenjiang 212013, China)
Abstract:
The microstructures and growth process characteristics of spherical Ni(OH)2 particles synthesized by the aqueous precipitation-crystallization method were investigated by SEM, TEM and XRD, and their growth mechanism was discussed. With the reaction beginning and continuing, amorphous Ni(OH)2 nano-crystallites grow up to spherical micron-particles with radially arranged crystallites. The nucleation, crystallization and re-crystallization led by Ostwald ripening simultaneously take place through the whole growth processes. With the course from reversible aggregation to irreversible agglomeration, the Ni(OH)2 particles tend to grow according to the template growth model: the growth on the crystallite templates stretching in the radius directions is free and quick, while the growth rate for crystallites in other directions is confined due to lower monomers concentration and tends to dissolve. So it is only the radially arranged crystallites that predominate in the particle and lead to characteristic microstructures.
Key words:
spherical Ni(OH)2; microstructure; Ostwald ripening; growth mechanism; crystallization;
1 Introduction
Spherical Ni(OH)2 have been widely used as the active materials for the nickel electrodes in Ni-MH batteries due to an appropriate packing density and high electrochemical performances. In the last two decades, a lot of investigations have been carried out to explain electrochemical processes for Ni-MH batteries by modeling Ni(OH)2 active materials’ microstructures and the electrode processes[1-2], because the microstructures of spherical Ni(OH)2 particles work on the electrochemical performance for the nickel electrodes in Ni-MH batteries. Many sophisticated methods therefore have been advanced to correlate the chemical composition-processing-microstructure-electrochemical performance of the materials[3-5]. Although the precipitation-crystallization processes of spherical Ni(OH)2 has been used in industry for many years[6-7], it is hard to produce spherical Ni(OH)2 with an appropriate particle size distribution, required precise chemical compositions and microstructures to meet the demand of high performances from the development of advanced mobile power technologies as the formation processing of spherical Ni(OH)2 is not completely understood, and it results in the product quality instable and the electrochemical performance disparity.
In this paper the formation of the microstructures and the growth mechanism of the spherical Ni(OH)2 synthesized with aqueous precipitation-crystallization method in a continuous stirring-tank reactor(CSTR) was studied.
2 Experimental
2.1 Synthesis of spherical Ni(OH)2
The required amounts of NiSO4, NaOH and NH4?H2O solutions were continuously fed into the CSTR at temperature of 50-55 ℃ and pH value of 11.0-11.5. The temperature and pH value were digitally responded and automatically controlled by adjusting the heater and reagents pumping speed automatically. The precipitate was sampled from the reactor at different times from the reaction beginning to the equilibrium state for the system to examine the particle characteristics with reaction time. The nickel hydroxide precipitates were washed with distilled water, then filtered and dried in an oven at 105 ℃.
2.2 Structural characterization
The interior section of a spherical Ni(OH)2 particle for the morphological observation was obtained with a method as follows[8]. One layer of spherical Ni(OH)2 powders was dispersed between two pieces of glasses, which were impressed carefully by hand without sliding. Some of Ni(OH)2 spheres could be crazed into two hemispheres and the interior sections can be observed by scanning electron microscope(SEM) with model of
JSM-5600LV instrument.
The sample of Ni(OH)2 particles for transmission electron microscopy(TEM) observation were prepared as follows[9]. The Ni(OH)2 particles were dispersed on a piece of metal and embedded when copper was plated. Then the flake embedded the Ni(OH)2 particles was thinned down to such a thickness that the Ni(OH)2 particles could be observed from either side. In the end, ion beams were applied to thin the sample to proper thickness from two sides. TEM investigation was done with a model of JEM-2000FX instrument.
X-ray diffraction(XRD) tests were done by a D/max-rA system employing Cu Ka radiation at 50 kV, 100 mA, with a graphite monochromator. Continuous scan was performed with scanning rate 4 (?)/min and scanning 2θ scope 10?-70?.
3 Results and discussion
3.1 Growth processes of Ni(OH)2 particle
The SEM images of Ni(OH)2 particle morphologies with different reaction times are shown in Fig.1. After 1 h of reaction, the precipitates are amorphous nano- particles in shape and loosely aggregated. The crystallites are like spindles with vague interfaces on the surfaces of the aggregated particles after 5 h. After 10 h growth, the crystallites become a little larger with much clear interfaces and the particles aggregate closely and become larger and more spherical. The particles become round and closely agglomerated spheres after 60 h growth, which are obviously the largest in size among the samples, and the crystallites in which keep the same spindle-like shape. The interior morphologies for typical Ni(OH)2 particles with 60 h growth time is shown in Fig.2. The particle consists of crystal flakes, which are mostly arranged in the radial direction and some of them are nearly as long as the radius of the sphere.
3.2 Growth mechanism of spherical Ni(OH)2
The Gibbs-Thomson effect, i.e. the equilibrium concentration at the surface of a cluster with curvature radius r is used in a truncated Taylor’s series form[10] as
(1)
where the index GT stands for Gibbs-Thomson, R and T are respectively the gas constant and thermodynamic temperature, c∞ is the equilibrium solubility at infinite cluster radius r, V is the mole volume of the solid, and γ is the solid-liquid interface energy. Eqn.(1) implies that the small particles or the surface with small curvature radius in solution will dissolve and the crystallizing monomers will diffuse to re-crystallize at large particles or on the surface with large curvature radius. This is called Ostwald ripening. It is easy to know that Ostwald ripening only occurs under the situation that the crystal monomer concentration is near to equilibrium.
Supersaturation S here is defined as follows:
S=c/c∞-1 (2)
The concentration of the reactant c is easy to decrease to c∞ since the precipitation reaction of Ni(OH)2 is a rapid course, that is to say, S→0 and Ostwald ripening occurs often. Besides the large particles grow up at the expense of small particles, the monomers diffuse on the particle surface from the bulge position to crater position, and make the particle become more spherical and smoother. KAMINSKI et al[11] gained the smooth
Fig.1 SEM images of spherical Ni(OH)2 particles at different reaction times
(a) 1 h; (b) 5 h; (c) 10 h; (d) 60 h
surface through growth interruption which leads to solid/liquid quasi-equilibrium condition(S→0) and the adatoms diffusion with Ostwald ripening rule.
Fig.2 TEM image of Ni(OH)2 particle at 60 h
According to boundary layer theory[12], there is a boundary layer on the surface of the crystallizing cluster, across which fluid moving rate, monomer concentration and fluid temperature alter most drastically, and they correspond to rate boundary layer, mass boundary layer and temperature boundary layer respectively. The rate boundary layer does not affect the crystallization, and the influence of the temperature boundary layer can be omitted since there is almost no heat effect in the Ni(OH)2 precipitation processes. Only the mass boundary layer affects the crystallization of Ni(OH)2 heavily. The mass transports by diffusion and convection in the boundary layer despite the movement state of the matrix fluid. The thickness of the mass boundary layer is negatively correlated with the moving rate of the fluid, so in the drastically stirring fluid the mass boundary layer δ→0, and the mass growth rate of crystal G is
G=ASn (3)
where A and n are both constants[12] .
The precipitation of Ni(OH)2 from alkaline Ni2+ fluid is a quick reaction, so the reaction rate depends on the slowest course-monomer diffusion, and the fluid on the surface of the cluster keeps in equilibrium despite the high supersaturation of the matrix fluid. The cluster and its near neighbors can compose a system in equilibrium. Within the system, Ostwald ripening also occurs despite the high reactants supersaturation.
The XRD patterns for samples at different reaction time in Fig.1 show similar characteristics of β-Ni(OH)2 phase as in Fig.3. The hexagonal crystal structure has been formed after 1 h growth in spite of its amorphous shape. The outside and inside microstructural differences for the Ni(OH)2 particles can be attributed to different thermodynamic and kinetic circumstances. It is supposed that some of the inner crystallites grow up by re-crystallization at the expense of outer crystallites. The dissolution of tiny Ni(OH)2 crystallites on the surface of the sphere and the recrystallization of the larger inner crystallites simultaneously take place. This leads to larger and better arranged crystallites[13].
Fig.3 XRD patterns for samples obtained at different reaction time
JIA et al[14] correlated supersaturation(S) and homogeneous nucleation or heterogeneous nucleation rate. He introduced the critical supersaturation for the homogeneous nucleation (Sc,homo) and heterogeneous nucleation(Sc,heter) to prcecipitation process. When Sc,heter<><>c,homo, the heterogeneous nucleation predominates; and when S>Sc,homo, the homogeneous nucleation occurs. When S>>Sc,homo, the homogeneous nucleation predominates and take places very quickly thereby the crystallization(interface growth) is prohibited. This is the case for normal Ni(OH)2. The growth processes for spherical Ni(OH)2 is schematically illustrated in Fig.4. Since the reactants(including ammonia which drastically decreases the supersaturation of Ni2+ concentration) are continuously fed into the reactor with acutely compulsive convection, it is supposed that the supersaturation S>Sc,homo, the homogeneous nucleation begins. But S not >>Sc,homo, so as time goes on, the increase in seed concentration facilitates the heterogeneous nucleation, and the controlled supersaturation permits mild crystal growth that leads to reasonable crystallinity as excellent electrochemistry performance asks.
The crystal structure of β-Ni(OH)2 is described as a hexagonal close-packed structure of hydroxyl ions (ABAB) with Ni2+ occupying octahedral interstices between two hydroxyl ions layers. A monolayer (001) comprises two hydroxyl ions and one Ni2+ cation, and the chemical bond between two monolayers is supposed to be hydrogen bond which is very weak so there is (001) complete cleavage which divides the crystal into many thin hexagonal platelets[15]. According to the periodic
Fig.4 Schematic illustration of template growth mechanism for spherical Ni(OH)2 particle
(Growth rate of crystal C, B and A decreases according to the sequence of SC>SB>SA, and the smallest crystal A dissolves through Ostwald ripening)
bond chain (PBC) theory[16], the crystal direction with strong chemical bond energy grows more quickly than that with weak chemical bond energy and the quick growth face tends to disappear during growth for β-Ni(OH)2, and the crystallites turn into platelet-like because of the crystallization habit. The platelet-like crystallites tend to “stand” with the sides of the platelets directing to the outsides on the surfaces of the particles due to template growth mechanism. Fig.4 schematically shows the template growth processes, in which the slow growth of the thickness for the crystallites is omitted. The mass boundary layer thickness δ→0 in the fully stirred fluid, because crystallites A, B and C are very near, it can be considered that B(B1B2B3) is in the boundary layer of C(C1C2C3) and A(A1A2) in that of B. The supersaturation for crystallites A, B and C is in the order: SA<>B<>C, so the growth rate will be in the sequence: A<>
Fig.5 Typical network microstructures of spherical Ni(OH)2 particle
(a) Intersection; (b) Surface
Fig.6 Formation processes of network microstructure for spherical Ni(OH)2 particle
growth stage, the grains are very tiny with high specific surface energy, so the aggregation occurs and the aggregated particle is composed of tiny crystallites irregularly littered, which forms the center of the sphere shown in Fig.5(a) unlike the out part of the sphere which grows up in radial direction following the template growth mechanism from the central crystallites. Among these platelet-like crystallites there are full of pores and crannies. This special radial microstructure forms a network frame and will benefit improvements for electrochemical performances of nickel hydroxides as the active positive materials in Ni-MH batteries due to the enhancement of proton diffusion and electronic conductivity in the materials[17].
4 Conclusions
1) During the reaction-crystallization precipitation process in aqueous solution, the Ni(OH)2 particles grow up from basically amorphous to spherical particles in shape with the sizes from nano-scale to several microns.
2) With the Ni(OH)2 particle growth, the nucleation, crystallization and Ostwald ripening almost simultaneously take place in the whole processes.
3) The Ni(OH)2 crystals tend to grow abiding by the template growth mechanism. The “Template Growth Model” suggests: during the growth processes beyond aggregation, the growth on the crystallite templates stretching in the radius directions is free and quick, while the growth rate for crystallites in other directions are confined due to short supply of monomers and tend to dissolve. So it is only the radially arranged crystallites that grow up quickly, stretch out directly to the surface of the particle, and leads to macro-spherical nickel hydroxide particles characterized with large radially arranged platelet-like crystallites. This special microstructure will benefit improvements for electrochemical performances of nickel hydroxides.
References
[1] SRINIVASAN V, WEIDNER J W, WHITE R E. Mathematical models of the nickel hydroxide active materials[J]. J Solid State Electrochem, 2000, 4(2): 367-382.
[2] WU B, WHITE R E. Modeling of a nickel hydrogen cell: phase reactions in the nickel active material[J]. J Electrochem Soc, 2001, 148(6): A595-A609.
[3] WEIDNER J W, TIMMERMANN P. Effect of proton diffusion, electron conductivity and charge transfer resistance on nickel hydroxide discharge curves[J]. J Electrochem Soc, 1994, 141(3): 346-351.
[4] MAO Z, VIDTS D P, WHITE R E. Theoretical analysis of the discharge performance of a NiOOH/H2 cell[J ]. J Electrochem Soc, 1994, 141(1): 54-64.
[5] SONG Quan-sheng, TANG Zhi-yuan, GUO He-tong, et al. Structural characteristics of nickel hydroxide synthesized by a chemical precipitation route under different pH values[J]. J Power Sources, 2002, 112(2): 428-434.
[6] WATANABE K, KIKUOKA T. Physical and electrochemical characteristics of nickel hydroxide as a positive material for rechargeable alkaline batteries[J]. J Appl Electrochem, 1995, 25(2): 219-226.
[7] ACHARYA R, SUBBAIAH T, ANAND S, et al. Effect of precipitating agents on the physicochemical and electrolytic characteristics of nickel hydroxide[J]. Materials Letter, 2003, 57(20): 3089-3095.
[8] PENG Mei-Xun, WANG Ling-sen, SHEN Xiang-qian, et al. Microstructures and formation mechanism of spherical β-Ni(OH)2[J]. The Chinese Journal of Nonferrous Metals, 2003,13(5): 1130-1135. (in Chinese)
[9] FANG Ke-ming. Study on microstructure of powder particles by TEM[J]. China Powder Science and Technology, 1999, 10(5): 8-10. (in Chinese)
[10] XIA H, ALLMANG M Z. Rate equation approach to the late stages of cluster ripening[J]. Physica A: Statistical and Theoretical Physics, 1998, 261(4): 176-187.
[11] KAMINSKI A Y, SURIS R A. Smoothing of crystal surfaces during growth interruption[J]. Applied Surface Science, 1996, 104/105: 312-316.
[12] ZHANG Ke-cong, ZHANG Le-hui. Crystal Growth Science and Technology(Vol.1)[M]. Beijing: Science Press, 1997. (in Chinese)
[13] SHEN Xiang-qian, PENG Mei-xun, JING Mao-xiang, et al. Formation of microstructures for spherical Ni(OH)2 particles[J]. J Functional Materials, 2005, 36(10): 1629-1633
[14] JIA Zi-quan, LIU Zhong-zhou. Process characteristics and principles of synthesis of nanosized particles by liquid precipitation methods[J]. Chinese Journal of Chemical Engineering, 2002, 30(1): 15-41. (in Chinese)
[15] DELAHAYE-VIDAL A, BEAUDOIN B, SAC-EPEE N, et al. Structural and textural investigations of the nickel hydroxide electrode[J]. Solid State Ionics, 1996, 84(4): 239-248.
[16] HORST J H T, KRAMER H J M, ROSMALEN G M V, et al. Molecular modeling of the crystallization of polymorphs. Part I: The morphology of HMX polymorphs[J]. J Crystal Growth, 2002, 237/239: 2215-2220.
[17] PENG Mei-xun, SHEN Xiang-qian, WANG Ling-sen, et al. Structural characteristics of spherical Ni(OH)2 and its charge/discharge process[J]. Journal of Central South University of Technology, 2005, 12(1): 5-8.
Foundation item: Project(50134020) supported by the National Natural Science Foundation of China; Project supported by Postdoctoral Fund of Central South University
Received date: 2006-10-24; Accepted date: 2006-12-27
Corresponding author: PENG Mei-xun, PhD; Tel: +86-731-8716990; E-mail: orally@163.com
- Template growth mechanism of spherical Ni(OH)2
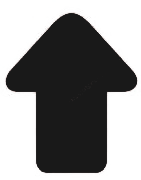