- Abstract:
- 1 Introduction▲
- 2 Magnetocaloric effect ▲
- 3 Criteria for selecting ...▲
- 4 Giant magnetocaloric ef...▲
- 5 New research progress o...▲
- 6 Conclusions ▲
- References
- Figure
- Fig.1 Comparison between GMCE materials and conventional MCE materials at μ0ΔH=5 T [8]
- Fig.2 The maximum MCE of Gd at its ordering temperature (Fitted equation of MCE for Gd at TC: ?Tad=3.675×(μ0H)0.7) [8]
- Fig.3 ΔSM at ?H=5 T for RCo2, RAl2, Gd5(Si1-xGex)4, Mn(As1-xSbx), MnFe(P1-xAsx) and La(Fe13-x Six) families plus a number of individual compounds versus Curie temperature [4]: 1─ErAl2; 2─(Dy0.7Er0.3)Al2; 3─DyAl2; 4─TbAl2; 5─(Tb0.4Gd0.6)Al2; 6─GdAl2; 7─Er(Co0.85Si0.15)2; 8─TbCo2; 9─Gd4Bi3; 10─Gd4(Bi2.25Sb0.75); 11─Gd4(Bi1.5Sb1.5); 12─Gd4(Bi0.75Sb2.25); 13─Gd4Sb3; 14─ErCo2; 15─HoCo2; 16─DyCo2; 17─Gd; 18─Gd5Si2.3Ge1.7; 19─Gd5Si3Ge; 20─Gd5Si4; 21─Gd5Si0.5Ge3.5; 22─Gd5SiGe3; 23─Gd5Si1.2Ge2.8; 24─Gd5Si1.3Ge2.7; 25─Gd5Si1.5Ge2.5; 26─Gd5Si1.6Ge2.4; 27─Gd5Si1.8Ge2.2; 28─Gd5Si1.95Ge2.05; 29─Gd5Si1.98Ge2.02; 30─Gd5Si2.02Ge1.98; 31─Gd5Si2.1Ge1.9; 32─HoCoAl; 33─DyCoAl; 34─TbCoAl; 35─GdCoAl; 36─MnAs; 37─MnFeP0.45As0.55; 38─TbN; 39─HoN; 40─Tb5Si2Ge2; 41─Dy5Si3Ge; 42─La(Fe11.7Si1.3); 43─La(Fe11.5Si1.5)H1.8; 44─La1.4Ca1.6Mn2O7; 45─Gd5Sn4; 46─Ni55.2Mn18.6Ga26.2
- Fig.4 Magnetic phase diagram of Gd5Si4-Ge5Ge4 pseudo-binary system [4]: ○—Monolinic Gd5(SiGe2)-type phase (Heat treated); ●—Monolinic Gd5(SiGe2)-type phase (As cast); ■—Orthorhombic Gd5Si4-type phase; ▲, ◆—Orthorhombic Gd5Ge4-type phase
- Fig.5 Dependence of isothermal magnetic entropy change ΔSM on temperature for La(Fe0.88Si0.12)13Hy alloys [46]
J. Cent. South Univ. Technol. (2009) 16: 0001-0012
DOI: 10.1007/s11771-009-0001-y
Development of magnetocaloric materials in room temperature magnetic refrigeration application in recent six years
LIU Min(刘 敏) , YU Bing-feng(俞炳丰)
(School of Energy and Power Engineering, Xi’an Jiaotong University, Xi’an 710049, China)
Abstract:
Some magnetocaloric materials were used successfully in magnetic refrigeration application and became one of the critical parts of magnetic refrigeration technology whose delightful progresses were made worldwide in the past 30 years. At the same time, the research on giant magnetocaloric materials will accelerate the development of room temperature magnetic refrigeration. In this paper, the new theoretical and experimental investigations on magnetic materials in room temperature application were described, including Gd and its binary and ternary intermetallic compounds, Mn-based compounds, La(Fe13-xMx)-based compounds and manganites. Based on the analysis of hysteresis, corrosion, cost and heat process, the comparison between different families of magnetic materials was discussed. Further research of room temperature magnetic refrigerant was suggested.
Key words:
magnetic material; refrigeration; magnetocaloric effect; magnetic resistance;
1 Introduction
Some magnetic materials, such as lanthanide metal Gd, MnAs, and lanthanide transition-metal-based compounds, generate magnetocaloric effect (MCE). Therefore, these magnetic materials can be adopted in magnetic refrigeration application. Gadolinium (Gd), is the mainly used magnetocaloric material at present. It was firstly applied in room temperature magnetic refrigeration by BROWN in 1976 and then its magnetocaloric physical properties were widely focused. Up to now, the magnetic materials with MCE have become one of the critical parts of magnetic refrigeration.
To improve efficiency of energy utilization and protect environment, investigators in the world have explored new refrigeration technology, as well as new substituting refrigerants. Room temperature magnetic refrigeration is an environment-safe refrigeration technology with many excellent features, such as compact configuration, low noise, high efficiency, high stability and longevity. The newly designed magnetic refrigeration components and systems use water-based heat transfer fluids, and these environmentally desirable products make minimal contributions to global warming. Moreover, efficiency improvements of 20%-30% compared with those of currently available vapor compression-based systems, are envisioned once technology development is completed [1]. Many investigators in America, China, Japan, and some European countries have focused on the performance research of room temperature magnetic refrigeration since the 1990s because of its great applicable prospect. As a result, room temperature magnetic refrigeration has been one of the hot topics in the world. Currently, it has been regarded as one of the nine advanced subjects of the International Institute of Refrigeration (IIR) and a corresponding international workgroup has been built. However, the room temperature magnetic refrigeration still stays in the stage of experimental investigations, and many practical and critical problems for this refrigeration technology are to be solved. The development of room temperature magnetic refrigerator and its application need further theoretical and experimental investigations on magnetic refrigerant, magnetic cycle, active magnetic regenerator (AMR) and corresponding system.
In 1999, PECHARSKY and GSCHNEIDNER [2] published a review paper describing the phenomenon of the MCE in magnetic materials under different temperature ranges and discussing the relationship between MCE and order-disorder magnetic phase transition. Then some important review articles on the development of magnetocaloric materials have been published in worldwide range since 2000. In 2003, YU et al [3] presented in detail the development of magnetic materials in room temperature regions, including Gd and its alloys, perovskite and perovskite-like compounds, transition metal compounds and composite material. In 2005, GSCHNEIDNER et al [4] reviewed systematically different families of magnetic materials, such as the lanthanide (R) Laves phases (RM2, where M=Al, Co andNi), Gd5(Si1-xGex)4, Mn(As1-xSbx), MnFe(P1-xAsx), La(Fe13-xSix) and their hydrides and the manganites (R1-xMxMnO3, where R=lanthanide and M= Ca, Sr and Ba). In 2007, PHAN and YU [5] reviewed a new class of magnetocaloric materials in the temperature range of 100-375 K, that is, the ferromagnetic perovskite manganites (R1-xMxMnO3, where R=La, Nd, Pr, and M= Ca, Sr, Ba), and analyzed the nature of these materials. BR?CK et al [6] reviewed a new class of magnetic refrigerant materials for room temperature applications in conjunction with a magnetic phase transition of the first order, including Gd5(Ge,Si)4 and related compounds, La(Fe,Si)13 and related compounds, MnAs-based compounds, Heusler alloys and Fe2P-based compounds. Then in 2008, GSCHNEIDNER and PECHARSKY [7] discussed the large scale production of giant magnetocaloric effect materials and potential problems for the use of giant magnetocaloric effect materials in magnetic refrigerators with the recent market investigation on rare earth metal. Based on the new published papers on room temperature magnetic refrigeration in recent six years, and proceedings of the first and the second IIF-IIR international conferences on magnetic refrigeration at room temperature, we presented the nature of MCE and giant magnetocaloric effect (GMCE), the criteria to select magnetic refrigerant, the new development of magnetic material in room temperature region, the comparison between different families of magnetic materials, and the further research of room temperature magnetic refrigerant in this paper.
2 Magnetocaloric effect
The magnetocaloric effect, which is intrinsic to all magnetic materials, is a consequence of magneto-thermal couplings between the magnetic entropy and the lattice entropy. When a magnetic field is applied to the material, the atomic magnetic moments of paramagnetic or soft ferromagnetic materials become aligned, making the material more ordered. Consequently, the materials expel heat and their magnetic entropy decreases. Otherwise, the magnetic moments return to their random directions, and the materials absorb heat and their magnetic entropy increases when the magnetic field is reduced isothermally.
The MCE can be related to the isothermal magnetic entropy change (ΔSM) and the adiabatic temperature change (ΔTad), which are shown in the following expression [8-9]:
(1)
(2)
where μ0 is the permeability of vacuum; H0 and Hi are the initial and the final magnetic field strength, respectively; CH is the heat capacity in constant magnetic field; andis the derivative of magnetization with respect to temperature in a constant magnetic field.
Other parameters for comparing magnetic materials are the refrigerant capacity, q, which indicates that how much heat can be transferred between the cold and hot sinks in one ideal refrigeration cycle, and the relative cooling power (PRCP). q and PRCP can be established as follows:
(3)
PRCP(S)=- ΔTFWHMΔSM(T, H) (4)
PRCP(T)=- ΔTFWHMΔTad(T, H) (5)
where ΔTFWHM is the full-width at half-maximum of ΔSM or ΔTad.
3 Criteria for selecting room temperature magnetic material
On the basis of the corresponding theoretical analysis and the nature of MCE, magnetic materials in magnetic refrigeration should satisfy several features for application, including [3, 5, 10]: (1) the large ΔSM and ΔTad (i.e. large total angular momentum number (J) and Lande factor (g) for ferromagnetic material); (2) the large density of magnetic entropy, which is an important factor contributing to the working efficiency of materials; (3) the small lattice entropy (i.e. the high Debye temperature); (4) the modest Curie temperature (TC) in the vicinity of room temperature to guarantee that the large magnetic entropy change can be obtained in the whole temperature range of the cycle; (5) the nearly zero magnetic hysteresis; (6) the very small thermal hysteresis; (7) the small specific heat and large thermal conductivity, which ensure remarkable temperature change and rapid heat exchange; (8) the large electric resistance (i.e. the lowering eddy current heating or the small eddy current loss); (9) the high chemical stability and simple sample synthetic route.
4 Giant magnetocaloric effect material
The discovery of the GMCE is a landmark in the development of room temperature magnetic refrigeration [11]. The GMCE results from the fact that a structural transformation takes place simultaneously with the magnetic ordering. In this case, both the magnetic sub-lattice and crystallographic sub-lattice are easily affected by the magnetic field. Therefore, the MCE in the first-order phase transition materials undergoing the coupled magneto-structural transformations (or the GMCE compounds) arises from the added difference of the entropies of the two crystallographic modifications (polymorphs) of the material [8]. Further research on the GMCE shall focus on its nature and mechanism.
For the GMCE materials, the added entropy change resulted from structural transformation in the first-order phase transition can be expressed by ΔSst, and hence the total entropy change is given by
(6)
An estimation based on comparison of the MCEs exhibited by closely related materials with and without the magnetic field-induced structural transformation indicates that ΔSst may account for more than a half of the total magnetic entropy change in magnetic fields less than 5 T [8, 12].
Fig.1 schematically compares the magnetocaloric effects in the first-order magnetic phase transition (FOMT) compounds (GMCE materials) and the second- order magnetic phase transition (SOMT) compounds (conventional MCE materials) [8], where TC is the transition temperature. It can be seen from the figure that the GMCE materials have much larger magnetic entropy changes compared with those of the conventional MCE compounds at any temperature, and the former have much wider applicable prospect.
Fig.1 Comparison between GMCE materials and conventional MCE materials at μ0ΔH=5 T [8]
5 New research progress of room tempera- ture magnetic material
The new research development of MCE materials near room temperature mainly focuses on Gd and its binary and ternary intermetallic compounds, Mn-based compounds, La(Fe13-xMx)-based compounds and manganites, which are to be reviewed in the next four sections.
5.1 Gd and its binary and ternary intermetallic compounds
As the mainly applied magnetic material at present, Gd has large MCE at the ordering temperature of TC=294 K. Fig.2 illustrates the maximum MCE of Gd measured indirectly or directly at its ordering temperature under different magnetic field strength [8]. The solid drawn through the data points represents the power-law fit of the data. It can be seen from the figure that the maximum ΔTad of Gd is in the range of 2.3-6.0 K for magnetic field changes between 0.5 and 2.0 T in permanent magnets.
Fig.2 The maximum MCE of Gd at its ordering temperature (Fitted equation of MCE for Gd at TC: ?Tad=3.675×(μ0H)0.7) [8]
GSCHNEIDNER et al [4] summarized the binary and ternary intermetallic compounds studied recently, including RCo2-based systems (R=Dy, Ho, and Er) [13-16], RAl2-based systems (R=Pr, Nd, Tb, Dy, Ho, Er, and Tm)[17], RNi2-based systems (R=Pr, Nd, Gd, Tb, Dy, Ho, and Er) [18] and miscellaneous intermetallic compounds [19-20]. The magnetic entropy change and Curie temperature of several magnetic material families at ΔH=5 T are shown in Fig.3, indicating that TC of the most binary and ternary intermetallic compounds deviates from room temperature seriously. However, TC of 13 kinds of compounds, including Gd4(Bi2.25Sb0.75), Gd4(Bi1.5Sb1.5), Gd4(Bi0.75Sb2.25), Gd4Sb3, Gd4Bi3, Gd5Si2.3Ge1.7, Gd5Si2.5Ge1.5, Gd5Si3Ge, Gd5Si1.98Ge2.02, Gd5Si2Ge2, Gd5Si2.09Ge1.91, Gd5Si2.02Ge1.98, and Gd5Si2.1Ge1.9, locates in the near room temperature region, and MCE of the former 8 kinds of refrigerant is smaller than that of Gd with the reverse situation for the latter 5 kinds of materials [4].
Fig.3 ΔSM at ?H=5 T for RCo2, RAl2, Gd5(Si1-xGex)4, Mn(As1-xSbx), MnFe(P1-xAsx) and La(Fe13-x Six) families plus a number of individual compounds versus Curie temperature [4]: 1─ErAl2; 2─(Dy0.7Er0.3)Al2; 3─DyAl2; 4─TbAl2; 5─(Tb0.4Gd0.6)Al2; 6─GdAl2; 7─Er(Co0.85Si0.15)2; 8─TbCo2; 9─Gd4Bi3; 10─Gd4(Bi2.25Sb0.75); 11─Gd4(Bi1.5Sb1.5); 12─Gd4(Bi0.75Sb2.25); 13─Gd4Sb3; 14─ErCo2; 15─HoCo2; 16─DyCo2; 17─Gd; 18─Gd5Si2.3Ge1.7; 19─Gd5Si3Ge; 20─Gd5Si4; 21─Gd5Si0.5Ge3.5; 22─Gd5SiGe3; 23─Gd5Si1.2Ge2.8; 24─Gd5Si1.3Ge2.7; 25─Gd5Si1.5Ge2.5; 26─Gd5Si1.6Ge2.4; 27─Gd5Si1.8Ge2.2; 28─Gd5Si1.95Ge2.05; 29─Gd5Si1.98Ge2.02; 30─Gd5Si2.02Ge1.98; 31─Gd5Si2.1Ge1.9; 32─HoCoAl; 33─DyCoAl; 34─TbCoAl; 35─GdCoAl; 36─MnAs; 37─MnFeP0.45As0.55; 38─TbN; 39─HoN; 40─Tb5Si2Ge2; 41─Dy5Si3Ge; 42─La(Fe11.7Si1.3); 43─La(Fe11.5Si1.5)H1.8; 44─La1.4Ca1.6Mn2O7; 45─Gd5Sn4; 46─Ni55.2Mn18.6Ga26.2
In 1997, the GMCE was first found in Gd-Si-Ge (GSG) alloys by PECHARSKY and GSCHNEIDER [21] at the AMES Laboratory, and the ΔSM and ΔTad of GSG alloys are larger than those of Gd by 70%-80% [21]. About 140 papers were published on the R5T4 materials, where R = a rare-earth element and T = Si, Ge or Sn [4] in mid-2004. The excitement for these compounds is not only due to their GMCE, but can also be ascribed to a number of other unusual features, such as a colossal magnetostriction and giant magnetoresistance. These excellent behaviors are a consequence of a coupled magnetic-structural first-order transition from either the paramagnetic (PM) monoclinic Gd5(Si2Ge2)-type structure to the ferromagnetic (FM) orthorhombic Gd5Si4-type structure, or from the antiferromagnetic (AFM) Sm5Ge4-type structure to the ferromagnetic orthorhombic Gd5Si4-type structure. An interesting feature is that the first-order magnetic phase transition (FOMT) temperature in Gd5(Si1-xGex)4 is strongly dependent on the molar ratio Si to Ge, as shown in Fig.4 [4]. In the figure, the solid lines indicate the magnetic phase boundaries, and the vertical dotted lines delineate the regions where the alloys are single phase materials.
Fig.4 Magnetic phase diagram of Gd5Si4-Ge5Ge4 pseudo-binary system [4]: ○—Monolinic Gd5(SiGe2)-type phase (Heat treated); ●—Monolinic Gd5(SiGe2)-type phase (As cast); ■—Orthorhombic Gd5Si4-type phase; ▲, ◆—Orthorhombic Gd5Ge4-type phase
YUCEL et al [22] investigated the effect of alloying Gd5(Si2-zGe2-zR2z) compound with 3d (Co and Mn) and p (Ga and B) orbits. They found that the substitution of Si and Ge could adjust TC of the compound between 20 and 286 K, with ΔSM variation in this process. For R=Mn, the maximum ΔSM is decreased by up to 17% with the increase of Mn content from z=0.01 to 0.1. When R denotes Co and z is above 0.04, the increase of Co content causes strong reduction of TC down to 260 K with a 13% increase of ΔSM. For R=Ga or B, the magnetic entropy is slightly reduced by 12% with increasing R content. CHEN et al [11] studied the magnetic properties of Gd5Si2-xGe2-xSn2x (x=0.05, 0.10, 0.15, 0.20, 0.25, and 0.50). They reported that ΔSM of the Gd5Si2-xGe2-xSn2x alloys gradually increases with the increase of Sn content from x=0 to x=0.25. For x≤0.2, the alloys have a dominant phase of monoclinic Gd5Si2Ge2-type crystal structure and a small quantity of Gd5Si4-type phase at room temperature, and the latter decreases with the increase of Sn content. The maximum ΔSM of Gd5Si1.75Ge1.75Sn0.5, which has only one paramagnetic-ferromagnetic magnetic phase transition, is as large as 16.7 J/(kg?K) under a magnetic field change of 1.8 T at its TC of 269 K. Its MCE exceeds that of Gd about two times. When Dy replaces Gd in Gd5Si4, XIE et al [23] found that TC decreases in a linear fashion from Gd5Si4 (TC=338 K) to Dy5Si4 (TC=140 K), but ΔSM only slightly lowers, by about 8% for (Gd2.5Dy2.5)Si4.
The GMCE in Gd-Si-Ge alloys can be negatively influenced by the impurities of the commercial Gd, because the FOMT is destroyed due to the impurities. WU and LU [24] evaluated the MCE of Gd-Si-Ge alloys made of Chinese commercial Gd, in which major impurities are C, O, N, and Ca, and the GMCE is mainly impaired by the first two elements. For magnetic refrigerant Gd, the poor resistance to corrosion in water will seriously impede against its wider application, and then it is needed to examine the influence of physical properties of different heat transfer solutions on corrosion of magnetic refrigerants. ZHANG et al [25] pointed out that NaOH solution with pH=10 is the best one to inhibit the corrosion of Gd in water.
Table 1 exhibits the MCE of newly investigated Gd-Si-Ge alloys and Mn-based compounds. The conclusion can be drawn from Table 1 that GSG alloys have the modest TC and large MCE for its application in room temperature regions, and they possess a prominent applicable potential.
Table 1 MCE of some Gd-Si-Ge alloys and Mn-based compounds
5.2 Mn-based compounds
The base material of Mn-based compounds is MnAs. Different alloys, such as Mn(As1-xSbx), MnFe(P1-xAsx), and Ni-Mn-Ga Heusler alloys, can be obtained through element substitution [4].
A large value of ΔSM=27 J/(kg?K) in MnAs can be obtained at a magnetic field change of 6 T. However, MnAs is not suitable for magnetic refrigerant as the FOMT of MnAs is accompanied with large thermal hysteresis. PALACIOS et al [29] pointed out that the problem above can be avoided by partial substitution of Sb for As. For MnAs1-xSbx (0≤x≤0.4), ΔSM reaches a maximum value of 25 J/(kg?K) as the magnetic field change increases from 0 to 6 T, and ΔTad reaches peak values between 10.8 K at x=0.4 and 12.4 K at x=0. Moreover, the addition of Sb makes TC tunable from 317 to 225 K with increasing x from 0 to 0.4, and the peak values of ΔSM under a magnetic field change of 5 T are in the range of 27-30 J/(kg?K) at 0.05≤x≤0.30 [30]. WADA et al [31] noted that Sb stabilizes the NiAs-type structure when x≥0.1, and the FOMT changes to SOMT, resulting in a reduction of ΔSM and ΔTad.
TEGUS et al [32] studied the effect of Cr and Co substitutions for Fe in MnFe(P1-xAsx) alloy. For the Mn(Fe1-xCrx)P0.47As0.53 alloy, whose TC is about 305 K, Cr lowers both TC and ΔSM. When ΔH=2 T and x=0.02, the FOMT changes to an SOMT, and ΔSM decreases by about 25% at its TC≈275 K. For the alloy Mn(Fe1-xCox)P0.5As0.5, a 10% substitution of Co for Fe (x=0.1) reduces TC from 282 to 260 K and ΔSM by about 55% at ΔH=2 T.
Ni-Mn-Ga Heusler alloy is a ferromagnetic alloy that undergoes a reversible FOMT from a tetragonal martensitic phase to the cubic austenitic phase. It has been paid a wide attention due to its large MCE recently [33]. In 2004, CHERECHUKIN et al [34] found a large ΔSM of 20.7 J/(kg?K) in Ni2.18Mn0.82Ga alloy at a magnetic field change of 1.8 T, which is the best result observed up to present. Then ZHANG et al [33] performed experimental investigations on Ni53.6Mn23.2Ga23.2 Heusler alloy. Their results indicate that a plateau in the peak of ΔSM exists and the distance between the two peaks is 4 K. ZHOU et al [35] reported large ΔSM for Ni55.2Mn18.6Ga26.2 alloy, with values of 20.37 J/(kg?K) at ΔH=5 T and TC=317 K and 9.46 J/(kg?K) at ΔH=1.5 T. The above description suggests that the Ni-Mn-Ga alloys near the Ni55Mn19Ga26 composition may be good magnetic regenerator alloys for refrigerators/heat pumps operating between 300 and 350 K. However, the ΔSM peak for Ni55.2Mn18.6Ga26.2 is quite sharp, only 5 K wide at half of the peak height maximum, which may limit the use of the Ni-Mn-Ga Heusler alloys as a near room temperature magnetic refrigerant.
Mn contained transition-metal-based compounds also present GMCE. For example, the maximum ΔSM of 26.1 J/(kg?K) in Mn1.1Fe0.9P0.76Ge0.24 alloy at TC=299 K and ΔH=5 T can be achieved [36]. In addition, the series of Fe2-xMxP (M = Ru, Rh, Pd, Pt) in the Fe2P type hexagonal structure and R2Fe17 (R=Pr, Y) system show large value of MCE, and the former shall be explored in further research [37-38].
5.3 La(Fe13-xMx)-based compounds
The heavy lanthanide metals and their compounds have the largest magnetic moments, which make them the best potential magnetocaloric materials. GUTFLEISCH et al [38] studied the magnetocaloric properties of La(FeSi)13 alloy. They obtained a ΔSM peak value of 10.3 J/(kg?K) in LaFe11.2Si1.8 alloy at 5 T and 240 K, and TC of LaFe11.2Si1.8 alloy can be easily adjusted between 200 and 290 K with the increase of Co content. LIU and ALTOUNIAN [39] investigated the La(Fe1-zCoz)11.4Si1.6 series of alloys from z=0 to z=0.10. They found that Co additions significantly and linearly increase TC from about 210 K at z=0 to about 330 K at z=0.10, but ΔSM decreases from 23.2 J/(kg?K) at z=0.02 to 11.6 J/(kg?K) at z=0.06 and ΔH=7 T. HUANG et al [40] studied the magnetocaloric properties of LaFe11.17Co0.78Si1.05 alloys prepared with low-purity raw materials in a low magnetic field. Their results indicate that proper addition amount of B to the alloys can improve the stability and enhance MCE and TC.
For La(FexSi1-x)13 alloys, their ΔSM and ΔTad can keep large maximum values even in a relatively low magnetic field after controlling TC by the hydrogenation and the partial substitution of Ce or Pr. FUJITA et al [41-45] investigated the MCEs of La1-zPrz(Fe0.88Si0.12)13, La1-zCez(Fe0.88Si0.12)13, and various La(Fe13-xSix)Hy samples. They found that the maximum values of ΔSM, ΔTad and PRCP in La0.5Pr0.5(Fe0.88Si0.12)13 alloy at a magnetic field change of 5 T are 30 J/(kg?K), 11.9 K and 576 J/kg, respectively, and TC increases up to 324 K by controlling y. For La(Fe0.88Si0.12)13H1.6 alloy, its TC is 330K, which is the largest in the La(Fe0.88Si0.12)13Hy compounds. Moreover, it has a large MCE and its refrigerant capacity achieves 130 J/kg, which is almost the same as that of Gd5Ge2Si2 and MnFeAs0.5P0.5 alloy. Fig.5 illustrates the temperature dependence of the isothermal magnetic entropy change for La(Fe0.88Si0.12)13Hy alloys [46]. It can be seen from the figure that the Curie temperature of these compounds can be increased by hydrogen absorption. In addition, the thermal conductivity of La(FexSi1-x)13Hy alloy is better than that of other candidates. Consequently, La(FexSi1-x)13Hy alloy is one of the most practical candidates for magnetic refrigerants working in a wide temperature range. Table 2 lists the magnetocaloric properties of new La(Fe13-xMx) compounds investigated, indicating that La(Fe13-xMx) compounds have the modest Curie temperature varying in room temperature region and much larger maximum ΔSM or PRCP compared with those of Gd through Co addition or hydrogen absorption.
Table 2 MCE of new La(Fe13-xMx) compounds studied
Fig.5 Dependence of isothermal magnetic entropy change ΔSM on temperature for La(Fe0.88Si0.12)13Hy alloys [46]
5.4 Manganites
In 1950, JONKER and van SANTEN firstly synthesized the distorted perovskite-type structure of manganites whose general formula is A1-xBxMnO3 (where A stands for trivalent rare-earth elements, such as La, Pr, Nd, Sm, Eu, Gd, Ho, Tb, and Y; and B denotes Na+, K+ and Ag+ , or bivalent alkaline earth ions such as Sr, Ca, Ba, and Pb) [5, 48]. The series of manganites have advantages of low cost, good chemical stability, small coercive force, and large electrical resistivity.
Manganites are now attracting attention in the field of magnetic refrigeration for their large MCE. For example, when the magnetic field changes are 6 and 8 T, the peak values of ΔSM in (La0.5Gd0.2)Sr0.3MnO3 alloy achieve 7.2 and 8.8 J/(kg?K), respectively [49]. The MCE above can satisfy the magnetic refrigerators presented in recent years. However, TC of a majority of manganites deviates from room temperature, which will limit their applications in room temperature regions. This problem can be avoided by alloying substituting ions. For example, La0.70Sr0.30MnO3 (LSMO) alloy is a ferromagnet presenting considerable magnetocaloric effect with TC less than 90℃, and then TC lowers effectively to near room temperature when La ion is substituted by Er or Eu ions, with increasing PRCP and keeping constant of ΔSM in this process [48].
DAS and DEY [50] studied a series of K-doped manganese perovskites of La1-xKxMnO3 type (x=0.05, 0.10, and 0.15) with nanometric crystallite size. They noted that TC of the compound enhances from 260.4 (x=0.05) to 309.7 K (x=0.15). Increasing K content in the compound increases the maximum ΔSM at 1 T to 3.00 J/(kg?K), which is about 89% to that reported for Gd. PRCP of LKMO compounds is nearly one-third to that reported for Gd (119 J/kg). HOU et al [51] examined single-phase polycrystalline samples of La0.8-xNdx- Na0.2MnO3 (x=0, 0.05, 0.10, 0.15 and 0.20) alloys by the sol-gel method. It is found that TC decreases with the increase of x, and the maximum ΔSM, for x=0.20, is 0.369 2 J/(mol?K) at about 295 K. HANH et al [52] fabricated La0.7Ca0.3-xPbxMnO3 (x=0.05, 0.10, 0.15, and 0.20) alloy with La1-xCaxMnO3 and La1-xPbxMnO3 by solid-state reaction technique with the expectation that they could establish large MCE in the room temperature region.
Some reports present that the crystal structure plays an important role in the transport, magnetic properties and thermodynamic properties of magnetic refrigerants. However, TERASHITA et al [53] observed the little influence of structural transition, which is in close proximity to the ferromagnetic transition, on ΔSM in La1-xSrxMnO3 compositions. In order to elucidate the effect of lattice structure on the MCE, KIM et al [54] performed an experimental study on ΔSM in La0.7Ca0.3-xSrxMnO3 (x=0.120, 0.135 and 0.150) ferromagnetics. The samples of x=0.120 and 0.150 show the usual M-T behavior with TC of 300 and 323 K, respectively. The composition with x=0.135 displays two-step dependence with inflection points at 309 and 320 K, which correspond to TC of orthorhombic and rhombohedral phases, respectively. Moreover, the maximum values of ΔSM are 1.87, 1.72, and 1.7 J/(kg?K) for the samples of x=0.120, 0.135, and 0.150, respectively. BEJAR et al [55] discussed new perovskite manganites La0.7Ca0.3-xKxMnO3 (0≤x≤0.150). The results show that these compounds crystallize in the orthorhombic structure for x≤0.075 and become rhombohedral for x=0.100 and 0.150. The disorder for the orthorhombic phase is enhanced with the increase of x content, which is followed by an increase of TC. For the rhombohedral structure, the disorder is suppressed, and thus TC decreases.
Table 3 shows the magnetocaloric properties of newly investigated manganites in near room temperature region. From Table 3, it can be noted that TC can be easily tuned in the temperature range of 290-360 K by selecting suitable manganites. The manganite materials with the superior magnetocaloric properties in addition to cheap materials processing cost will be an option of magnetic material in the future magnetic refrigeration technology.
Table 3 Magnetocaloric properties of manganites near room temperature
5.5 Comparison between various magnetic refri- gerants near room temperature
Many investigators [71–78] revealed that the magnetic hysteresis and thermal hysteresis might have negative influence on magnetic refrigerants exhibiting the GMCE. However, the greater concern is not the hysteresis of the FOMTs, but the time dependence of ΔTad [4]. Unfortunately, nothing is known about the time dependence of ΔTad for the manganites, the Mn(As1-xSbx), MnFe(P1-xAsx) and Heusler alloy families.
Moreover, corrosion of different magnetic refrigerants in water-based solution should not be ignored in most magnetic refrigerators. Thus, suitable magnetic materials should be selected on the basis of the factors as mentioned above for given magnetic refrigerator. Table 4 [4] summarizes the advantages and disadvantages of candidate magnetic materials, where a zero indicates that the factor is essentially the same as that of Gd; a plus means that the behaviour is somewhat better than that of Gd, and two pluses mean that the behavior is much better; a minus sign indicates that the property is inferior to that of Gd, and two or three minus signs indicate the behaviour is much worse than that for Gd; the question mark indicates that it is not clear whose specific performance between some magnetic materials and Gd is better. From the table, it can be found that Gd and its solid-solution alloys may occupy their prominent positions in room temperature magnetic refrigeration because of their excellent over-all properties; and LaFeSi alloys may be a suitable choice for their low raw material cost and relatively high MCE in large scale application.
Table 4 Advantages and disadvantages of various magnetic refrigerants
6 Conclusions
(1) Magnetic material is a critical part in magnetic refrigeration, but the present magnetic materials in room temperature region cannot match the demand of practical application. For example, Gd and Gd-Si-Ge alloys have a narrow applicable temperature region, and the Curie temperature of many manganites deviates from room temperature. In order to seek magnetic materials of low cost and large MCE, further studies will be focused on making full use of magnetic entropy change, overcoming magnetic hysteresis and thermal hysteresis, achieving large MCE in relative low magnetic field, and reducing heat capacity of materials under zero magnetic field strength.
(2) The GMCE results from the fact that a structural transformation takes place simultaneously with the magnetic ordering. The next investigation should be performed on the mechanism and relationship between the structural transformation and the magnetic ordering. In other words, the connection between the MCE and the magnetic-phase transformation is to be emphasized in the future study on GMCE materials. The most promising compounds for room temperature magnetic refrigeration include: Gd5(Si2Ge4-x) alloys with x near 2; MnAs and MnAs1-xSbx; FeMn(P1-xAsx) and related compounds; and La(Fe0.88Si0.12)13Hy with y≥1.
References
[1] RUSSEK S L, ZIMM C. Potential for cost effective magnetocaloric air conditioning systems [C]// Proceedings of the First IIF–IIR International Conference on Magnetic Refrigeration at Room Temperature. Montreux, Switzerland, 2005.
[2] PECHARSKY V K, GSCHNEIDNER Jr K A. Magnetocaloric effect and magnetic refrigeration [J]. Journal of Magnetism and Magnetic Materials, 1999, 200(1/3): 44-56.
[3] YU Bing-feng, GAO Qiang, ZHANG Bin, MENG Zhao-xiang, CHEN Zhi. Review on research of room temperature magnetic refrigeration [J]. International Journal of Refrigeration, 2003, 26(6): 622-636.
[4] GSCHNEIDNER Jr K A, PECHARSKY V K, TSOKOL A O. Recent developments in magnetocaloric materials [J]. Reports on Progress in Physics, 2005, 68(66): 1479-1539.
[5] PHAN M H, YU S C. Review of the magnetocaloric effect in manganite materials [J]. Journal of Magnetism and Magnetic Materials, 2007, 308(2): 325-340.
[6] BR?CK E, TEGUS O, THANH D T C, BUSCHOW K H J. Magnetocaloric refrigeration near room temperature (invited) [J]. Journal of Magnetism and Magnetic Materials, 2007, 310(2): 2793-2799.
[7] GSCHNEIDNER Jr K A, PECHARSKY V K. Thirty years of near room temperature magnetic cooling: Where we are today and future prospects [J]. International Journal of Refrigeration, 2008, 31(6): 945-961.
[8] PECHARSKY V K, GSCHNEIDNER Jr K A. Advanced magnetocaloric materials: What does the future hold [J]. International Journal of Refrigeration, 2006, 29(8):1239-1249.
[9] TISHIN A M, SPICHKIN Y I. The magnetocaloric effect and its applications [M]. Bristol: Institute of Physics Publishing, 2003: 4-68
[10] TISHIN A M. Physical Mechanisms of large magnetoccaloric effect [C]// Proceedings of the First IIF–IIR International Conference on Magnetic Refrigeration at Room Temperature. Montreux, Switzerland, 2005.
[11] CHEN Y G, ZHANG T B, TANG Y B, TU M J. Magnetic phase transition and magetic entropy change of Gd5Si2-xGe2-xSn2x alloys [C]// Proceedings of the First IIF–IIR International Conference on Magnetic Refrigeration at Room Temperature. Montreux, Switzerland, 2005.
[12] PECHARSKY V K, GSCHNEIDNER Jr K A. Magnetocaloric effect associated with magneto-structural transitions [C]// Magnetism and Structure in Functional Materials. Heidelberg, 2005, 79: 199-222.
[13] WANG YU, YANG Sen, SONG Xiao-ping. Low-field magnetic properties and magnetocaloric effect in annealed and as-cast Dy(Co1-xSix)2 compounds [J]. Journal of Alloys and Compounds, 2003, 354(1/2): 81-84.
[14] WANG Dun-hui, TANG Shao-long, LIU Hai-dong, ZHONG W, DU You-wei. The study of magnetic entropy change in Dy(Co1-xMx)2 (M=Al, Si, Ga, Ge) compounds [J]. Materials Letters, 2003, 57(24/25): 3884-3888.
[15] TROPER A, von RANKE P J, de OLIVEIRA N A. Magnetocaloric effect in the pseudobinary Ho(Co1-cRhc)2 [J]. Journal of Magnetism and Magnetic Materials, 2004, 272/276: 583-584.
[16] SINGH N K, TRIPATHY S K, BANERJEE D, TOMY C V, SURESH K G, NIGAM A K. Effect of Si substitution on the magnetic and magnetocaloric properties of ErCo2 [J]. Journal of Applied Physics, 2004, 95 (11): 6678-6680.
[17] de OLIVEIRA N A, von RANKE P J, TROPER A. Magnetocaloric effect in rare-earth pseudobinary Er(Co1-cNic)2 [J]. Physical Review B, 2004, 69: 064421.
[18] von RANKE P J, GRANGEIA D F, CALDAS A, de OLIVEIRA N A. Investigations on magnetic refrigeration: Application to RNi2 (R=Nd, Gd, Tb, Dy, Ho, and Er) [J]. Journal of Applied Physics, 2003, 93(7): 4055-4059.
[19] NAKAGAWA T, SAKO K, ARAKAWA T, YAMAMOTO T A. Magnetocaloric effect of mononitride containing gadolinium and dysprosium GdxDy1-xN [J]. Journal of Alloys and Compounds, 2004, 364(1/2): 53-58.
[20] PLAZA E J R, ALVES C S, COELHO A A, GAMA S, VON RANKE P J. Magnetic and magnetocaloric properties of Nd monopnictides [J]. Journal of Magnetism and Magnetic Materials, 2004, 272/276(III): 2373-2374.
[21] PECHARSKY V K, GSCHNEIDNER Jr K A. Giant magnetocaloric effect in Gd5(Si2Ge2) [J]. Physical Review Letters, 1997, 78(23): 4494-4498.
[22] YUCEL A, ELERMAN Y, AKSOY S. Effects of alloying on magnetocaloric characteristics of Gd5(Si2Ge2) [C]// Proceedings of the First IIF–IIR International Conference on Magnetic Refrigeration at Room Temperature. Montreux, Switzerland, 2005.
[23] XIE Kun, SUN Zhan-bo, ZHU Yao-min, YANG Sen, SONG Xiao-ping. Magnetic entropy change in (Gd1-xDyx)5Si4 compound [J]. Journal of Alloys and Compounds, 2004, 372(1/2): 49-51.
[24] WU W, LU D W. Study on Gd-Si-Ge alloys using Chinese Gd [C]// Proceedings of the First IIF–IIR International Conference on Magnetic Refrigeration at Room Temperature. Montreux, Switzerland, 2005.
[25] ZHANG Z Y, LONG Y, YE R C, CHANG Y Q, WU W. Corrosion resistance of magnetic refrigerant Gadolinium in water [C]// Proceedings of the First IIF–IIR International Conference on Magnetic Refrigeration at Room Temperature. Montreux, Switzerland, 2005.
[26] YAN A, HANDSTEIN A, KERSCHL P, NENKOV K, M?LLER K H, GUTFLEISCH O. Effect of composition and cooling rate on the structure and magnetic entropy change in Gd5SixGe4-x [J]. Journal of Applied Physics, 2004, 95(11): 7064-7066.
[27] DUPUIS C, ARTIGAS M, BALLI M, CHAJEK W, FRUCHART D, GIGNOUX D, KEDOUS-LEBOUC A, TOBOLA J, WIENDLOCHA B, ZACH R, ZIEBA A. Giant magnetocaloric effect in Mn1-t(Ti0.5V0.5)tAs compounds near room temperature [C]// Proceedings of the Second IIF–IIR International Conference on Magnetic Refrigeration at Room Temperature. Portoroz, Slovenia, 2007: 105-111.
[28] SASSO C P, GIUDICI L, PASQUALE G M. Negative and positive magnetocaloric effect in NiMnSn [C]// Proceedings of the Second IIF–IIR International Conference on Magnetic Refrigeration at Room Temperature. Portoroz, Slovenia, 2007: .
[29] PALACIOS E, TOCADO L, BURRIEL R, WADA H. Heat capacity and direct determination of the magnetocaloric effect in MnAs1-xSbx (x=0, 0.1, 0.3, 0.4) [C]// Proceedings of the First IIF–IIR International Conference on Magnetic Refrigeration at Room Temperature. Montreux, Switzerland, 2005.
[30] WADA H, FUNABA C H, ASANO T, ILYN M, TISHIN A M. Recent progress of magnetocaloric effect of MnAs1-xSbx [C]// Proceedings of the First IIF–IIR International Conference on Magnetic Refrigeration at Room Temperature. Montreux, Switzerland, 2005.
[31] WADA H, MORIKAWA T, TANIGUCHI K, SHIBATA T, YAMADA Y, AKISHIGE Y. Giant magnetocaloric effect of MnAs1-xSbx in the vicinity of first-order magnetic transition [J]. Physica B, 2003, 328(1/2): 114-116.
[32] TEGUS O, BRUCK E, LI X W, ZHANG L, DAGULA W, de BOER F R, BUSCHOW K H J. Tuning of the magneto-caloric effects in MnFe(P, As) by substitution of elements [J]. Journal of Magnetism and Magnetic Materials, 2004, 272/276(III): 2389-2390.
[33] ZHANG Z, LONG Y, WEN D. Magnetocaloric effect and phase transition in Ni53.6Mn23.2Ga23.2 Heusler alloy [C]// Proceedings of the First IIF–IIR International Conference on Magnetic Refrigeration at Room Temperature. Montreux, Switzerland, 2005.
[34] CHERECHUKIN A A, TAKAGI T, MATSUMOTO M, BUCHEL'NIKOV V D. Magnetocaloric effect in Ni2+xMn1-xGa Heusler alloys [J]. Physics Letters A, 2004, 326(1/2): 146-151.
[35] ZHOU X Z, LI W, KUNKEL H P, WILLIAMS G. A criterion for enhancing the giant magnetocaloric effect: (Ni-Mn-Ga)—A promising new system for magnetic refrigeration [J]. Journal of Physics: Condensed Matter, 2004, 16(6): L39-L44.
[36] YAN A, MULLER K H, SCHULTZ L, GUTFLEISCH O. Magnetic entropy change in melt-spun MnFePGe (invited) [J]. Journal of Applied Physics, 2006, 99(8): 08K903.
[37] BALLI M, FRUCHART D, HLIL E K, MIRAGLIA S, de RANGO P, WOLFERS P, GINOUX D, ALLAB F, LEBOUC A M. FOURNIER J, YONNET J P. Magnetocaloric effect in some Fe2P like compounds [C]// Proceedings of the First IIF–IIR International Conference on Magnetic Refrigeration at Room Temperature. Montreux, Switzerland, 2005.
[38] GUTFLEISCH O, YAN A, KUZ′MIN M, LYUBINA J, M?LLER K H, SCHULTZ L. Research activities on magnetocaloric effect at IFW Dresden [C]// Proceedings of the First IIF–IIR International Conference on Magnetic Refrigeration at Room Temperature. Montreux, Switzerland, 2005.
[39] LIU X B, ALTOUNIAN Z. Effect of Co content on magnetic entropy change and structure of La(Fe1-xCox)11.4Si1.6 [J]. Journal of Magnetism and Magnetic Materials, 2003, 264(2/3): 209-213.
[40] HUANG J H, SONG L, JIN P Y, YAN H W, WANG Y, TEGUS O, ZHANG J X. The magnetocaloric effect in LaFe11.17-xCo0.78Si1.05Bx alloys and present research undertaken [C]// Proceedings of the Second IIF–IIR International Conference on Magnetic Refrigeration at Room Temperature. Portoroz, Slovenia, 2007: 155-160.
[41] FUJITA A, FUJIEDA S, HASEGAWA Y, FUKAMICHI K. Itinerant–electron metamagnetic transition and large magnetocaloric effects in La(FexSi1-x)13 compounds and their hydrides [J]. Physical Review B, 2003, 67: 104416.
[42] FUJITA A, FUKAMICHI K. Influence of volume change due to hydrogenation and partial substitution on magnetocaloric effects in La(FexSi1-x)13 magnetic refrigerants [C]// Proceedings of the First IIF–IIR International Conference on Magnetic Refrigeration at Room Temperature. Montreux, Switzerland, 2005.
[43] FUJIEDA S, FUJITA A, FUKAMICHI K. Control of large magnetocaloric effects in La1-zPrz(FexSi1-x)13Hy magnetic refrigerants working around room temperature [C]// Proceedings of the First IIF–IIR International Conference on Magnetic Refrigeration at Room Temperature. Montreux, Switzerland, 2005.
[44] FUKAMICHI K, FUJITA A, FUJIEDA S. Large magnetocaloric effects and thermal transport properties of La(FeSi)13 and their hydrides [J]. Journal of Alloys and Compounds, 2006, 408/412: 307-312.
[45] FUJITA A, FUJIEDA S, FUKAMICHI K. Relative cooling power of La(FexSi1-x)13 after controlling the Curie temperature by hydrogenation and partial substitution of Ce [J]. Journal of Magnetism and Magnetic Materials, 2007, 310(2): 1006-1007.
[46] TASKAEV S V, BUCHELNIKOV V D, SOKLOVSKIY V V. Theoretical description of magnetocaloric effect in La-Fe-Si alloys [C]// Proceedings of the Second IIF–IIR International Conference on Magnetic Refrigeration at Room Temperature. Portoroz, Slovenia, 2007: 89-97.
[47] CHEN Yuan-fu, WANG Fang, SHEN Bao-gen, HU Feng-xia, SUN Ji-rong, WANG Guang-jun, CHENG Zhao-hua. Magnetic properties and magnetic entropy change of LaFe11.5Si1.5Hy interstitial compounds [J]. Journal of Physics: Condensed Matter, 2003, 15(7): L161-L167.
[48] AMARAL J S, REIS M S, ARAUJO J P, TAVARES P B, AMARAL V S. Development of manganite materials for room-temperature magnetocaloric applications [C]// Proceedings of the First IIF–IIR International Conference on Magnetic Refrigeration at Room Temperature. Montreux, Switzerland, 2005.
[49] SUN Young, TONG Wei, LIU Ning, ZHANG Yu-heng. Magnetocaloric effect in polycrystalline (La0.5Gd0.2)Sr0.3MnO3 [J]. Journal of Magnetism and Magnetic Materials, 2002, 238(1): 25-28.
[50] DAS S, DEY T K. Magnetic entropy change in polycrystalline La1-xKxMnO3 perovskites [J]. Journal of Alloys and Compounds, 2007, 440(1/2): 30-35.
[51] HOU Deng-lu, YUE Cai-xia, YUN Bai, LIU Qing-hua, ZHAO Xiu-ying, TANG Gui-de. Magnetocaloric effect in La0.8-xNdxNa0.2MnO3 [J]. Solid State Communications, 2006, 140(9/10): 459-463.
[52] HANH D T, ISLAM M S, KHAN F A, MINH D L, CHAU N. Large magnetocaloric effect around room temperature in La0.7Ca0.3-xPbxMnO3 perovskites [J]. Journal of Magnetism and Magnetic Materials, 2007, 310(2): 2826-2828.
[53] TERASHITA H, MYER B, NEUMEIER J J. Influence of a first-order structural transition on magnetocaloric effects in manganese oxides [J]. Physical Review B, 2005, 72: 132415.
[54] KIM J S, ULYANOV A N, KANG Y M, MIN S G, YU S C, YOO S I. Influence of structural-phase transition on the magnetocaloric effects of lanthanum manganites [J]. Journal of Magnetism and Magnetic Materials, 2007, 310(2): 2818-2819.
[55] BEJAR M, FEKI H, DHAHRI E, ELLOUZE M, BALLI M, HLIL E. Effects of substituting divalent by monovalent ion on the physical properties of La0.7Ca0.3-xKxMnO3 compounds [J]. Journal of Magnetism and Magnetic Materials, 2007, 316(2): 707-709.
[56] PHAN M H, PHAN T L, YU S C, NGUYEN D T, CHAU N. Large magnetocaloric effect in La0.845Sr0.155Mn1-xMxO3 (M=Mn, Cu, Co) perovskites [J]. Physica Status Solidi B, 2004, 241: 1744-1747.
[57] SZEWCZYK A, GUTOWSKA M, DABROWSKI B, PLACKOWSKI T, DANILOVA N P, GAIDUKOV Y P. Specific heat anomalies in La1–xSrxMnO3 (0.12≤x≤0.20) [J]. Physical Review B, 2005, 71: 224432.
[58] PHAN M H, TIAN S B, HOANG D Q, YU S C, NGUYEN C, ULYANOV A N. Large magnetic-entropy change above 300 K in CMR materials [J]. Journal of Magnetism and Magnetic Materials, 2003, 258/259: 309-311.
[59] PHAN M H, TIAN S B, YU S C, ULYANOV A N. Magnetic and magnetocaloric properties of La0.7Ca0.3-xBaxMnO3 compounds [J]. Journal of Magnetism and Magnetic Materials, 2003, 256(1/3): 306-310.
[60] ZHONG W, CHEN W, AU C T, DU Y W. Dependence of the magnetocaloric effect on oxygen stoichiometry in polycrystalline La2/3Ba1/3Ca0.3MnO3-δ [J]. Journal of Magnetism and Magnetic Materials, 2003, 261(1/2): 238-243.
[61] CHAU N, NHAT H N, LUONG N H, MINH D L, THO N D, CHAU N N. Structure, magnetic, magnetocaloric and magnetoresistance properties of La1-xPbxMnO3 perovskite [J]. Physica B: Condensed Matter, 2003, 327(2/4): 270-278.
[62] MIN S G, KIM K S, YU S C, SUH H S, LEE S W. Magnetocaloric properties of Lai1-xPbxMnO3 (x=0.1, 0.2, 0.3) compounds [J]. IEEE Transactions on Magnetics, 2005, 41(10): 2760-2762.
[63] HAN M H, YU S C, HUR N H. Excellent magnetocaloric properties of La0.7Ca0.3-xSrxMnO3(0.05≤x≤0.25) single crystals [J]. Applied Physics Letters, 2005, 86: 072504.
[64] SUN W A, LI J Q, AO W Q, TANG J N, GONG X Z. Hydrothermal synthesis and magnetocaloric effect of La0.7Ca0.2Sr0.1MnO3 [J]. Powder Technology, 2006, 166(2): 77-80.
[65] LI J Q, SUN W A, AO W Q, TANG J N. Hydrothermal synthesis and magnetocaloric effect of La0.5Ca0.3Sr0.2MnO3 [J]. Journal of Magnetism and Magnetic Materials, 2006, 302(2): 463-466.
[66] PHAN M H, TIAN S B, YU S C. Magnetic and magnetocaloric properties of La0.7Ca0.3-xBaxMnO3 compounds [J]. Journal of Magnetism and Magnetic Materials, 2003, 256(1/3): 306-310.
[67] PHAN M H, PENG H X, YU S C. Manganese perovskites for room temperature magnetic refrigeration applications [J]. Journal of Magnetism and Magnetic Materials, 2007, 316(2): 562-565.
[68] BEJAR M, DHAHRI E, HLIL E K, HENITI S. Influence of A-site cation size-disorder on structural, magnetic and magnetocaloric properties of La0.7Ca0.3-xKxMnO3 compounds [J]. Journal of Alloys and Compounds,2007, 440(1/2): 36-42.
[69] BEJAR M, DHAHRI R, DHAHRI E, BALLI M, HLIL E K. Large magnetic entropy change at room temperature in La0.7Ca0.3-xKxMnO3 [J]. Journal of Alloys and Compounds, 2007, 442(1/2): 136-138.
[70] CHEN W, NIE L Y, ZHONG W, SHI Y J, HU J J, LI A J, DU Y W. Magnetocaloric effect in Nd doped perovskite La0.7-xNdxBa0.3MnO3 polycrystalline near room temperature [J]. Journal of Alloys and Compounds, 2005, 395(1/2): 23-25.
[71] PROVENZANO V, SHAPIRO A J, SHUL R D. Reduction of hysteresis losses in the magnetic refrigerant Gd5Ge2Si2 by the addition of iron [J]. Nature, 2004, 429(6994): 853-857.
[72] BASSO V, BERTOTTI G, LOBUE M, SASSO C P. Theoretical approach to the magnetocaloric effect with hysteresis [J]. Journal of Magnetism and Magnetic Materials, 2005, 290/291: 654-657.
[73] SASSO C P, BASSO V, LOBUE M, BERTOTTI G. Carnot cycle for magnetic materials: The role of hysteresis [J]. Physica B: Condensed Matter, 2006, 372(1/2): 9-12.
[74] BASSO V, SASSO C P, BERTOTTI G, LOBUE M. Effect of material hysteresis in magnetic refrigeration cycles [J]. International Journal of Refrigeration, 2006, 29(8): 1358-1365.
[75] BASSO V, SASSO C P, LOBUE M. Thermodynamic aspects of first-order phase transformations with hysteresis in magnetic materials [J]. Journal of Magnetism and Magnetic Materials, 2007, 316(2): 262-268.
[76] RIVAS M, GARCIA J A, ANGELES M, FAL-MIYAR V, TEJEDOR M. Time dependence of hysteresis loop displacement in hard-soft magnetic systems [J]. Journal of Magnetism and Magnetic Materials, 2006, 304: e684-e686.
[77] SATO TURTELLI R, SINNECKER J P, GROSSINGER R. Magnetic orderings and temperature dependence of the hysteresis loops of Nb1-xFe2+x [J]. Journal of Magnetism and Magnetic Materials,2007, 316(2): e492-e495.
[78] XIA Z C, WU Y Y, ZHU G F, HUB A Z, FENG X N, WANGA Y, XIONG C S, YUAN S L, TANG C Q. Magnetoresistance and hysteresis phenomena of (La0.75Bi0.25)0.67Ca0.33MnO3 [J]. Journal of Magnetism and Magnetic Materials, 2008, 320: 368-373.
Foundation item: Project(50876082) supported by the National Natural Science Foundation of China; Project (IRT0746) supported by the Changjiang Scholars and Innovative Research Team in University
Received date: 2008-07-05; Accepted date: 2008-09-06
Corresponding author: YU Bing-feng, Professor; Tel: +86-29-82667953; E-mail: bfyu@mail.xjtu.edu.cn
- Development of magnetocaloric materials in room temperature magnetic refrigeration application in recent six years
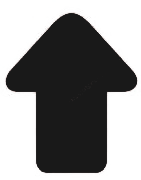