- Abstract:
- 2 Experimental ▲
- 3 Results and discussion ▲
- 4 Conclusions▲
- References
- Figure
- Fig.1 TEM image of silica
- Fig.2 Complex viscosity (η*) as function of oscillation stress (σ) for SiO2/PEG suspension
- Fig.3 Storage modulus (G′) and loss modulus (G″) as functions of oscillation stress (σ) for SiO2 /PEG suspension
- Fig.4 Lissajous plots for SiO2/PEG suspension of different stress amplitudes
- Fig.5 Scaled Lissajous plots for PEG(★)and SiO2/PEG suspension at stress amplitudes
- Fig.6 Energy dissipated (Ed) as function of measured maximum strain (γmax) for SiO2 /PEG suspension
J. Cent. South Univ. Technol. (2007)06-0737-05
DOI: 10.1007/s11771-007-0140-y
Viscoelastic properties of monodisperse spherical silica suspension
WU Qiu-mei(伍秋美), RUAN Jian-ming(阮建明), HUANG Bai-yun(黄伯云),
ZHOU Zhong-cheng(周忠诚), ZOU Jian-peng(邹俭鹏)
(State Key Laboratory of Powder Metallurgy, Central South University, Changsha 410083, China)
Abstract:
The viscoelastic properties of the suspension of monodisperse spherical silica produced by hydrolysis of tetraethoxysilane in alcohol solvent with ammonia as a catalyst in polyethylene glycol (PEG) were studied. The results show that the SiO2/PEG suspension possesses the reversible shear thinning and shear thickening behaviors. In the shear thinning region, the loss modulus (G″) almost remains unchanged, whereas the storage modulus (G′) decreases. In the shear thickening region, G″ and G′ increase for the formation of the “clusters”. The larger G″ over G′ in all the stress studied shows that the system mainly possesses the viscous property, and that the energy dissipated(Ed) is larger than that stored. Ed of this suspension is proportional to the maximum strain (γmax) rising with the exponent of 1.92 under low shear stress; however, in the shear thickening region, Ed is proportional to γmax rising with the exponent of 5.00.
Key words:
viscoelasticity; monodisperse silica; suspension; shear thickening;
1 Introduction
Many colloidal suspensions, such as paints, coatings, and lubricants, are subjected to rapid shearing during processing. In some instances the shear force can be strong enough to drive shear thickening, which is marked by a rapid, sometimes discontinuous increase in viscosity with small increase in shear rate. This behavior can induce dramatic changes in the suspension microstructure such as driving irreversible flocculation and particle aggregation and damage processing equipment. The change in microstructure leads to poor fluid and coating qualities. Thereby a number of documents have been addressed about the factors that influence the shear thickening degree. For example, the volume fraction of the particles has no influence on the critical stress where shear thickening takes place[1-2], the increasing particle size and the lower temperature make the shear thickening behavior take place at lower shear stress and shear rate, and the increase in the polydispersity of the particles decreases the severity of the shear thickening[1-3].
On the other hand, this shear thickening behavior could be exploited in the design of damping and control devices, whereby the fluid can limit the maximum rate of flow through a highly nonlinear response[4]. LEE et al[5] and TAN et al[6] used the shear-thickening properties of the silica suspensions in ethylene glycol, polyethylene glycol (PEG) and water to make advanced body armor materials. These materials can offer equivalent ballistic performance of existing body armor materials, but with significantly more compactness and flexibility. Despite a lot of documents have reported the rheological properties of the shear thickening suspensions[7-9], there is little information addressing the viscoelastic properties of this suspension in detail. In this work, the controlled stress rheometer was used to study the viscoelastic properties of the monodisperse spherical silica suspension in PEG and the energy dissipated in the stress shear was calculated.
2 Experimental
2.1 Materials
The monodisperse spherical silica was produced by hydrolysis of tetraethoxysilane in alcohol solvent using ammonia as a catalyst[10-11]. The silica produced by these means is hydrophilic due to the presence of hydroxyl groups (Si-OH) on the surface[12].
A polar liquid, polyethylene glycol(PEG), with average relative molecular mass of 200 provided by Sinopharm Chemical Reagent Co Ltd was used as the continuous phase. It is a Newtonian liquid with low viscosity of 0.03 Pa·s at 25 ℃.
2.2 Morphology of silica
The morphology of silica was observed on H-800 transmission electron microscope(TEM) after silica was dispersed in ethanol by ultrasonic treatment for 30 min and then placed on copper foil for drying.
2.3 Sample preparation
The suspension with the volume fraction of SiO2 of 51% was prepared by adding the silica powder into the liquid in a blender and stirring for approximately 30 min. The samples were placed under vacuum at room temperature for 24 h to remove air bubbles. When the silica powder was dispersed in polar suspending fluid, the hydroxyl groups of the silica preferentially interacted with suspending molecules through hydrogen bonds, leading to nonflocculated suspension[12]. The volume fraction of the silica in the suspension was calculated from the mass fraction, the density of the silica and PEG. Here, the silica density was calculated from solution densimetry measurements using a volumetric balance assuming ideal mixing.
2.4 Viscoelastic behavior measurements
The viscoelastic behavior measurements were conducted on a stress-controlled rheometer AR2000 (TA Company, USA). Experiments were conducted under oscillatory stress shear at 25 ℃, and the frequency was fixed at 20 rad/s and the shear stress was in the range from 0.01 to 1 000 Pa. In all the experiments, cone-plate fixture (diameter of 40 mm, angle of 2?) was used.
3 Results and discussion
3.1 Morphology of silica
The morphology image of silica observed by H-800 transmission electron microscope (TEM) is shown in Fig.1. TEM measurement demonstrates that the particles are nearly monodisperse, spherical ones with the average diameter of 500 nm.
Fig.1 TEM image of silica
3.2 Dynamic rheological behavior
The dependence of the complex viscosity (η*) on the shear stress (σ) for the silica suspension at the frequency of 20 rad/s is presented in Fig.2. As can be observed that at low amplitude of σ, η* almost remains unchanged with increasing σ (RegionⅠ), and then η* decreases (RegionⅡ). After reaching a minimum value, η* starts to increase (Region Ⅲ). The point where η* is the minimum is defined as the critical point and the stress at this point is denoted as the critical shear stress (σc).
Fig.2 Complex viscosity (η*) as function of oscillation stress (σ) for SiO2/PEG suspension
The change in the viscosity of the silica suspension is assumed to be the result of the microstructure change. At small stress amplitude, Brownian motion and the interparticle forces are able to restore the structure to the equilibrium state during the oscillatory, therefore the viscosity almost remains unchanged. When the stress increases further, the structure breakdown caused by the stress shear becomes significant and Brownian motion and the interparticle forces are no longer capable of restoring the microstructure, so the viscosity decreases. Certainly, the relaxation of the molecular chain of the PEG has contribution to the decrease in the viscosity. At the critical point, the hydrodynamic forces equal the repulsive interactions, after that the hydrodynamic forces exceed the repulsive interactions, and the temporary clusters of the silica particles form[4,13-14]. These clusters are separated by a thin solvent layer and play an important role in blocking the flow of the fluid, which makes η* increase as the shear stress increases.
To confirm the reversibility of these shear thinning and shear thickening behaviors, η* was measured for both ascending and descending stress sweeps, as shown in Fig.2. The good agreement except some hysteresis of η* under the low stress for the descending plot shows that the “clusters” can decompose and disperse into the suspending fluids again when the shear stress decreases. This indicates that the shear-thinning and shear- thickening behaviors are reversible.
3.3 Storage modulus and loss modulus
The dependence of storage modulus (G′) and loss modulus (G″) on the oscillatory stress (σ) for SiO2/PEG suspension is shown in Fig.3. The experimental conditions are the same as those in Fig.2. It is clear that the system also shows linear viscoelastic (RegionⅠ), shear thinning (RegionⅡ) and shear thickening (Region Ⅲ) behavior as increasing σ, and the magnitude of G″ is larger than that of G′ in all the stress ranges studied. According to the viscoelastic theory[15],elasticity is considered to be the behavior of solid, whereas viscidity is the behavior of fluid. The values of storage modulus G′ and loss modulus G″ represent the intensity of elasticity and viscidity, respectively, and the complex viscosity η* is composed of elastic-like viscosity (G′/ω) and the viscous-like viscosity (G″/ω). In this system, the larger G″ over G′ in the entire range of the stress indicates the suspension possesses the viscous property predominantly, and the portion of the energy dissipated by viscous forces is larger than that stored in the structure of the suspension for this system.
Fig.3 Storage modulus (G′) and loss modulus (G″) as functions of oscillation stress (σ) for SiO2 /PEG suspension
It can be observed that the plot of G′ in Fig.3 has obvious decrease, while the plot of G″ only shows slight decrease in region II. These phenomena were also observed by BENDER and WAGNER[16] and KAFFASHI et al[17]. The stress-optical relationship shows that the shear thinning of those hard-sphere silica suspensions is due to the changes in the thermodynamic contribution to the viscosity, while the hydrodynamic contribution remains relatively constant throughout the shear thinning regime, and BENDER and WAGNER[16] also provided evidence that shear-thickening was attributed to the increased hydrodynamic interactions. In the experiments of KAFFASHI et al[17], the shear rate appears to be too low for the shear thickening to occur, while in the the shear thinning region, they observed that the elastic-like viscosity introduced by the Brownian motion was contributed to the shear- thinning and appeared to be sensitive to structure changes in the sheared suspension, conversely the viscous-like viscosity, due to hydrodynamics, was approximately independent of the shear rate.
3.4 Lissajous plot
To further understand the viscoelastic properties of the suspension, the dissipated energy under stress shear can be obtained by integrating the area contained in a plot of stress vs strain (Lissajous plot) for a cycle stress shear[18-19]. During oscillatory shear, for every stress point σ, sine stress wave: σ=σmaxsin(ωt) will be exerted on the suspension with σ being the maximum stress σmax. When one cycle shearing of sine stress wave is performed, there will be a maximum strain γmax. The area circled by the Lissajous plot is the energy dissipated for one cycle shearing of sine stress wave. The Lissajous plots of the SiO2/PEG suspension at 0.1, 2.0, 500.0 Pa in linear viscoelastic, shear thinning and shear thickening ranges respectively at the frequency of 20 rad/s are shown in Fig.4. Comparing these figures, it is clear that as increasing the stress, the area circled by the plots becomes larger, which means the dissipated energy becomes more.
Fig.4 Lissajous plots for SiO2/PEG suspension of different stress amplitudes
(a) σmax=0.1 Pa; (b) σmax=2.0 Pa; (c) σmax=500.0 Pa
It is clear that the larger the shear stress is, the more the energy will be dissipated, but the shapes of Lissajous plots are different in Fig.4. The normalized strain (γ/γmax) as a function of normalized applied stress (σ/σmax) when the stress is 0.1, 0.5, 2.0, 5.0, 40.0, 100.0, 250.0 and 500.0 Pa (sinusoidal frequency ω=20 rad/s) for the SiO2/PEG suspension is shown in Fig.5. For the sake of comparing the suspension with the suspending fluid PEG (Newtonian fluid), the same experiment was performed on PEG, as shown in Fig.5. For the Newtonian fluid PEG, the scaled Lissajous plot is nearly a superposed line, whereas the scaled Lissajous plots for suspension deviate from a superposed line and take on elliptic forms. The more closely the shear stress approaches the critical stress, the more similar the shape of Lissajous plot is to that of the curve of PEG. Compared with Fig.3, when σ=σc=5.0 Pa for the SiO2/PEG suspension, G′ being the minimum, the portion of G″ in G* is larger than that of any other points, that is, the system takes on fluid property the most obviously, so the plot is the most similar to that of PEG.
Fig.5 Scaled Lissajous plots for PEG(★)and SiO2/PEG suspension at stress amplitudes
3.5 Dissipated energy
Through the mathematical treatment, the relation between the dissipated energy (Ed) and the maximum strain (γmax) during one cycle shear can be obtained from the relation[18-20]:
(1)
It is clear that when G″ is constant, Ed is a quadratic function of γmax, and when G″ is variable, Ed is affected by both of γmax and G″.
Fig.6 shows the relation between the dissipated energy Ed and the maximum strain γmax for SiO2/PEG suspension when σ is 0.1, 0.5, 2.0, 5.0, 40.0, 100.0, 250.0 and 500.0 Pa, respectively. It can be seen that under low stress shear (before shear thickening), G″ nearly remains constant as increasing shear stress, the slope of Ed vs γmax obtained on the log-log plot for SiO2/PEG suspension is 1.92, approximating to two, as anticipated by Eqn.(1). However, in the shear thickening region, G″ increases as the shear stress increasing, Ed of SiO2/PEG suspension is proportional to γmax rising with the exponent about 5, that is to say, G″ increases proportionally to γmax with the power law relation in the shear thickening region.
Fig.6 Energy dissipated (Ed) as function of measured maximum strain (γmax) for SiO2 /PEG suspension
4 Conclusions
1) The monodisperse spherical silica suspension in PEG shows reversible shear thinning and shear thickening behaviors. During shear thinning region, G″ almost remains unchanged, whereas η* and G′ decrease. During shear thickening region, all of η*, G′ and G″ increase at the same time due to the formation of the temporary and flow-induced clusters.
2) The viscoelastic measurements indicate that in the entire range of stress studied, G″ is larger than G′, indicating that the portion of the energy dissipated is more than that stored, and the suspension shows viscous behavior predominantly.
3) The slope of energy dissipated (Ed) vs maximum strain(γmax)on the log-log plots approach to 2 under low shear stress. However, in the shear thickening region, Ed of SiO2/PEG suspension is proportional to γmax rising with the exponent of about 5.
References
[1] MARANZANO B J, WAGNER N J. The effect of particle size on reversible shear thickening of concentrated colloidal suspensions[J]. J Chem Phys, 2001, 114(23): 10514-10527.
[2] RAGHAVAN S R, KHAN S A. Shear-thickening response of fumed silica suspensions under steady and oscillatory shear[J]. J Colloid Interface Sci, 1997, 185: 57-67.
[3] LEE J D, SO J H, YANG S M. Rheological behavior and stability of concentrated silica suspensions[J]. J Rheol, 1999, 43(5): 1117-1139.
[4] SUCIU C V, IWATSUBO T, DEKI S. Investigation of a colloidal damper [J]. J Colloid Interface Sci, 2003, 259: 62-80.
[5] LEE Y S, WETZEL E D, WAGNER N J. The ballistic impact characteristics of Kevlar woven fabrics impregnated with a colloidal shear thickening fluid[J]. Journal of Materials Science, 2003, 38: 2825-2833.
[6] TAN V B C, TAY T E, TEO W K. Strengthening fabric armour with silica colloidal suspensions[J]. Solids and Structures, 2005, 42: 1561-1576.
[7] JONATHAN B, WAGNER N J. Reversible shear thickening in monodisperse and bidisperse colloidal suspensions[J]. J Rheol, 1996, 40(5): 899-916.
[8] MARAZANO B J, WAGNER N J. The effects of interparticle interactions and particle size on reversible shear thickening: Hard-sphere colloidal suspensions[J]. J Rheol, 2001, 45(5): 1205-1222.
[9] RAGHAVAN S R, KHAN S A. Shear-induced microstructural changes in flocculated suspensions of fumed silica[J]. J Rheol, 1995, 39(6): 1311-1325.
[10] CHANG S M, LEE M, KIM W S. Preparation of large monodispersed spherical silica particles using seed particle growth[J]. J Colloid Interface Sci, 2005, 286: 536-542.
[11] CHEN Sheng-li, DONG Peng, YANG Guang-hua, et al. TEM examination and mechanism of the formation of monosize colloidal silica spheres[J]. J Inorg Mater, 1998, 13(3): 368-374. (in Chinese)
[12] ZHAO Li, YU Jia-guo, CHENG Bei, et al. Preparation and formation mechanism of monodispersed silicon dioxide spherical particles[J]. Acta Chim Sinica, 2003, 61(4): 562-566. (in Chinese)
[13] MARANZANO B J, WAGNER N J. Flow-small angle neutron scattering measurements of colloidal suspension microstructure evolution through the shear thickening transition[J]. J Chem Phys, 2002, 117(22): 10291-10302.
[14] HOFFMAN R L. Explanations for the cause of shear thickening in concentrated colloidal suspensions[J]. J Rheol, 1998, 42(1): 111-123.
[15] LI Xiao-jun. Practical Approach to Rheology and Rheometry[M]. Beijing: Petroleum Industry Press, 1998: 113. (in Chinese)
[16] BENDER J W, WAGNER N J. Optical measurement of the contributions of colloidal forces to the rheology of concentrated suspensions[J]. J Colloid Interface Sci, 1995, 172: 171-184.
[17] KAFFASHI B, O’BRIEN V T, MACKAY M E. Elastic-like and viscous-like components of the shear viscosity for nearly hard sphere, Brownian suspensions[J]. J Colloid Interface Sci, 1997, 187: 22-28.
[18] CITERNE G P, CARREAU P J, MOAN M. Rheological properties of peanut butter[J]. Rheol Acta, 2001, 40: 86-96.
[19] LEE Y S, WAGNER N J. Dynamic properties of shear thickening colloidal suspensions[J]. Rheol Acta, 2003, 42: 199-208.
[20] YZIQUEL F, CARREAU P J, TANGUY P A. Non-linear viscoelastic behavior of fumed silica suspensions[J]. Rheol Acta, 1999, 38: 14-25.
Foundation item: Projects(50774096; 50606017) supported by the National Natural Science Foundation of ChinaReceived date: 2007-03-07; Accepted date: 2007-05-20
Corresponding author: RUAN Jian-ming, PhD, Professor; Tel:+86-731-8876644; E-mail: jianming@mail.csu.edu.cn
- Viscoelastic properties of monodisperse spherical silica suspension
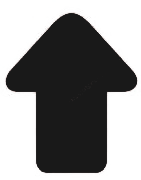