- Abstract:
- 1 Introduction▲
- 2 ExperimentsExperimental▲
- 3 Results and discussions▲
- 4 Conclusions▲
- References
- Figure
- Fig.1 Photo of compaction mould
- Fig.2Specimen Photos of specimen of compacted bentonite: (a) Un- confined specimen; (b)confined Confined specimen in self-designed cell
- Fig.3 Setup of vapour phase technique
- Fig.4 Comparison of SWRCs in unconfined/confined wetting paths at different temperatures
- Fig.5 Relationship between Ratio kt versusand suction (t1 is 40 ℃, and t2 is 80 ℃)
- Fig.6 Hysteretic characteristics of unconfined compacted GMZ bentonitein at high suctionat and different temperatures: (a) Hysteretic characteristic curves; (b) Relationship between suction and hysteretic coefficients
- Fig.7 Hysteretic behaviors of unconfined compacted GMZ bentonite in different suction ranges of suction at 40 ℃ (B1 stands for hysteretic cycle inat high suction and B2 stands for hysteretic cycle inat low suction): (a) Hysteretic characteristics curves; (b) Relationship between suction and hysteretic coefficients
J. Cent. South Univ. Technol. (2009) 16: : 0821- -0826
DOI: 10.1007/s11771-009- -0136- x
Effect of temperature on soil-water characteristics and hysteresis of compacted Gaomiaozi bentonite
YE Wei-min(叶为民)1, 2, WAN Min(万 敏)1, CHEN Bao(陈 宝)1,
CHEN Yong-gui(陈永贵)1, CUI Yu-jun(崔玉军)3, WANG Ju(王 驹)4
(1. Key Laboratory of Geotechnical and Underground Engineering of, Ministry of Education,
Tongji University, Shanghai 200092, China;
2. United Research Center for Urban Environment and Sustainable Development,
Ministry of Education, Shanghai 200092, China;
3. Ecole des Ponts Paries Tech, Paris, France;
4. Beijing Research Institute of Uranium Geology, Beijing 100029, China)
Abstract:
Laboratory tests under different constraint conditions were carried out to obtain the soil-water retention curves (SWRCs) of highly-compacted confined/unconfined Gaomiaozi (GMZ) bentonite at 20, 40 and 80 ℃, respectively. The effect of temperature on the soil-water characteristics of the highly-compacted GMZ bentonite was analyzed. The results show that the water retention capacity of the highly-compacted GMZ bentonite decreases as the temperature increases under unconfined and confined conditions. At a certain temperature, the constraint conditions have little influence on the water retention capacity of the compacted bentonite at high suction, but the water retention capacity of the confined specimen is lower than that of the unconfined specimen at low suction. Under unconfined conditions, the hysteretic behaviour of the compacted bentonite decreases with the temperature increasing temperature. At high suction (>4 MPa), the hysteretic behaviour of the unconfined bentonite tends to increase with the decrease of the suction. In summary, the hysteretic behaviour of the compacted bentonite is not significant.
Key words:
1 Introduction
In the design concept of multi-barrier system of deep geological disposal for high-level radioactive waste, the highly-compacted bentonite has been widely used for construction of artificial barrier. Because of the radiogenic heat released from the waste container, the infiltration groundwater from the surrounding rock and the mechanical stress induced by the hygroscopic expansion of the working compacted bentonite, the highly-compacted bentonite experiences coupling of thermo-hydro-mechanical process. In order to ensure the safety and effective operation of high-level radioactive waste repository, it is important to investigate the effect of temperature on hydro-mechanical characteristics of the compacted bentonite used as buffer materials [1].
Soil-water characteristic is one of the key indexes of hydro-mechanical property of bentonite. Much work wasMany studieds were conducted on the effect of temperature on soil-water characteristics of the highly-compacted bentonite [2-5]. ROMERO et al [6], and VILLAR and LLORET [7] experimentally obtained soil-water retention curves of the compacted BOOM clay and FEBEX bentonite by different temperature experiments, respectively. JACINTO et al [8] measured the retention curves of different dry densities of MX-80 bentonite following the wetting path at different temperatures, and modified the van Genuchten’s model by considering the effect of temperature on the water retention capacity.
ROMERO et al [6], and VILLAR and LLORET [7] experimentally obtained soil-water retention curves of the compacted BOOM clay and FEBEX bentonite by different temperature experiments, respectively. JACINTO et al [8] measured the retention curves of the different dry densities of MX-80 bentonite following the wetting path at different temperatures, and modified the Van Genuchten’s model by considering the effect of temperature on the water retention capacity.
But the above studies only concerned about the effect of temperature on the soil-water characteristics following single drying/wetting path. In fact, under the influence of gas generation and groundwater infiltration during of radioactive waste disposal, the compacted bentonite has a soil-water hysteresis caused by the multi-drying/ wetting circles in a certain range of suction [9-10]. The studies on the soil-water hysteresis were focused on developing thermo-dynamic models of hysteresis for soil-water characteristic curves, such as the wetting circles in a certain range of suction [9-10]. The studies on the soil-water hysteresis were focused on developing thermo-dynamic models of hysteresis for soil-water characteristic curves, such as the bounding surface model [11-12], and the internal- variable model [13]. Based on the internal-variable theory of capillary hysteresis . , XU et al [14] developed a mathematical model to describe the soil-water characteristics of geotechnical media experiencing arbitrary drying-wetting paths. The effect of air entrapment was fully taken into account in XU’s model.
6ujuowing ature increasesed NaClGaomiaozi (GMZ) bentonite was selected as buffer materials of Chinese geological repository for high level radioactive nuclear waste [15]. CHEN et al [16] studied the soil-water characteristic curves of highly-compacted bentonite using the osmotic technique and vapor equilibrium technique to control suction. YE et al [17] studied the swelling pressure characteristics of the GMZ bentonite by the constant volume test, and found that there was an exponential relationship between the swelling pressure and the dry density. QIN et al [18] analyzed the effects of the dry density, the vertical stress and the soaking liquid on the swelling characteristics of the GMZ bentonite. The objective of the present study is to investigate the temperature effects on the soil-water characteristics and hysteretic behaviors of highly- compacted GMZ bentonite under unconfined and confined conditions.
2 ExperimentsExperimental
2.1 Materials
The properties of GMZ bentonite used in the experiment are shown in Table 1, indicating that GMZ bentonite has high cation exchange capacity, great adsorption ability, good dispersion and good hydraulic properties.
The GMZ bentonite powder was compacted into a thin cylindrical specimen. A digital frame was employed for the compaction. The compaction was digitally controlled at a vertical velocity of 0.1 mm/min to ensure the specimen uniformity. Even so, it is difficult to achieve the optimum homogenization [19].
The initial state parameters of the specimen are listed in Table 2.
2.2 Apparatus
The compaction devices are shown in Fig.1, including a steel mould and a piston. The bentonite powder can be compressed into a thin cylindrical specimen in the steel mould under the universal press.
Self-designed cells with small holes in two ends are
Table 1 Properties of GMZ bentonite
The GMZ bentonite powder was compacted into a thin cylindrical specimen. A digital frame was employed for the compaction. The compaction was digitally controlled at the vertical velocity of 0.1 mm/min to ensure the specimen uniformity. Even though, it is difficult to achieve the optimum homogenization [19].
The initial state parameters of the specimen are listed in Table 2.
Table 2 Initial state parameters of specimen
2.2 Equipments
The compaction devices are shown in Fig.1, including a steel mould and a piston. The bentonite powder can be compressed into a thin cylindrical specimen in the steel mould under the universal press.
Fig.1 Photo of compaction mould
Self-designed cells with small holes in two ends are shown in Fig.2, which were employed for suction control test under confined conditions. The holes were designed as channels for moisture exchanges between specimen in the cell and the circulating air around it. For the test under unconfined conditions, the specimen was placed inside an aluminous container as shown in Fig.2,.
The apparatus using vapour phase technique suggested by YE et al [20], as shown in Fig.3, was placed in the desiccator, and the salt solution was used
Fig.2Specimen Photos of specimen of compacted bentonite: (a) Un- confined specimen; (b)confined Confined specimen in self-designed cell
The apparatus using vapour phase technique suggested by YE et al [20], as shown in Fig.3, was employed to control the suction in this test. The specimen was placed in the desiccator, and the salt solution was used for suction control. The air pump was usedfor keeping air in circulation, and the whole system was placed in a digital oven for temperature control with the resolution of ±0.1 ℃.
Fig.3 Setup of vapour phase technique
for suction control. The air pump was used to keep air in circulation. The whole system was placed in a digital oven for temperature control with the resolution of (±0.1) ℃.
2.3 Methods and test procedure
2.3.1 Temperature control
In order to investigate temperature effects on soil-water characteristics of the compacted Gaomiaozi GMZ bentonite, 20, 40 and 80 ℃ were selected for temperature control in the tests.
2.3.2 Suction control
The vapour equilibrium method was employed to control the suction. Different saturated salt solutions were used to control the relative humidity of the circulating air in the whole system, and t. Their corresponding imposed suctions at 20, 40 and 80 ℃ were are shown in Table 3. The high suctions (>4 MPa) were calibrated by TANG and CUI [21] and the low suctions were controlled by unsaturated NaCl solutions suggested by LYTTON [22].
Table 3 Corresponding suction of salt solution at different temperatures (unit: MPa)
In order to obtain the temperature effect on the soil-water characteristics of highly-compacted GMZ bentonite, soil-water retention (SWR C) tests of GMZ bentonite following drying/wetting cycles were carried out under both unconfined and confined conditions at 20, 40 and 80 ℃ respectively, and soil water retention curves (SWRCs) and hysteretic circles were obtained correspondingly.
3 Results and discussions
3.1 Soil-water retention curves (SWRCs) of highly-compacted GMZ bentonite
T6f compacted bentonite is not soTThe SWRCs of the highly-compacted GMZ bentonite following wetting path at different temperatures under unconfined and confined conditions are shown in Fig.4. It can be seen from Fig.4 that, the water retention capacity under both confined and unconfined compacted bentonite decreases with the temperature increasing temperature, and the influence increases with the suction decreasing suction at a certain suction.
Fig.4 Comparison of SWRCs in unconfined/confined wetting paths at different temperatures
The mechanism for the influence of temperature on the water retention capacity of the highly-compacted bentonite can be analyzed in the aspect of the low suction and the high suction, respectively [7]. At high suction (>4 MPa), the change of clay fabric and intra-aggregate water plays a significant role in water retention capacity of GMZ bentonite. Much intra- aggregate water moves into the macropore with temperature increasing temperature. The excessive water flows out from the macropore, for degree of saturation is invariable in macropores at a certain suction. Therefore, the water retention capacity of bentonite decreases as the temperature increases. At low suction, capillary effect plays a decisive role in the performance of the water retention capacity. The changes of surface tension caused by the increasinge of temperature, result in the increase of the water content of bentonite with increasing the temperature increasing at a certain suction.
The effects of the constraint conditions on the water retention capacity of bentonite can also be analyzed in the aspect of the high and low suctions. At high suction, the influence of constraint conditions on the soil-water retention capacity of bentonite is not significant, which agrees with the result obtained by CHEN et al [16]. But at low suction, the water retention capacity of the confined specimen is clearly lower than that of unconfined specimen. The difference between water content of the compacted bentonite under the confined and unconfined conditions becomes greater with the suction decreasing suction, which suggests that water retention capacity has a close relationship with the changes of the microstructure. Under the unconfined conditions, the inter-aggregate pores of bentonite expand constantly, and the water can flow into these pores easily. Therefore, the water retention capacity of the unconfined specimen increases rapidly as the suction decreases. As clay particles can only swell and collapse into the macropores under confined conditions, the increasing rate of water content in the confined specimen is significantly lower than that of in the unconfined specimen with the decrease of the controlling suction.
In order to analysisze the influence of temperature on the water retention capacity, a ratio (kt), which indicates that the water content changes with temperature, is defined as,
where are the water contents in the SWRCs at temperature t1 and t2 to andfor a given suction, respectively.
The relationship between kt and the suction in temperature range from 40 to 80 ℃ is shown in Fig.5, indicating that the influence of temperature on the water retention capacity has a close relationship with the suction. And temperature has a great effect on the water retention capacity in the suction range from 40 to 80 MPa. It can also be seen from Fig.5 that the impact of constraint conditions on kt tends to increase with decreasing suction.
3.2 Hysteretic behaviour of SWRC
The hysteretic characteristic curves for unconfined GMZ bentonite following the wetting/drying cycles in high suction at 20 and 40 ℃ are shown in Fig.6(a). It is quite obvious that the hysteresis loop of SWRC at 20 ℃ is larger than that at 40 ℃. That is to say, the hysteretic
Fig.5 Relationship between Ratio kt versusand suction (t1 is 40 ℃, and t2 is 80 ℃)
The relationship between kt with temperature changing from 40 ℃ to 80 ℃ and the suction is shown in Fig.5,indicating that the influence of temperature on the water retention capacity has a close relationship with the suction. And the temperature has the great effect on the water retention capacity in the suction range from 40 to 80 MPa. It is also can be seen from the Fig.5 that the impact of constraint conditions on the kt trend toward increasing with suction decreasing.
3.2 Hysteretic behaviour of SWRC
The hysteretic characteristic curves for unconfined GMZ bentonite following the wetting/drying cycles in high suction at 20 and 40 ℃ are shown in Fig.6(a). It is quite obviously that the hysteresis loop of SWRC at 20 ℃ is larger than that at 40 ℃. That is to say, the hysteretic behaviour of the compacted bentonite is less obvious as temperature increases. The hysteretic coefficient, η, wasintroduced to quantitatively describe the hysteretic behavior of bentonite of water retention curves following wetting/drying cycles, which can be expressed as
×100% (2)
where wd and ww are the water contents in the drying, wetting path to a given matric suction, respectively. Eqn.(2) indicates that thehysteretic behaviour is less significant when η is small.
Fig.6 Hysteretic characteristics of unconfined compacted GMZ bentonitein at high suctionat and different temperatures: (a) Hysteretic characteristic curves; (b) Relationship between suction and hysteretic coefficients
behaviour of the compacted bentonite is less obvious as temperature increases. The hysteretic coefficient, η, was introduced to quantitatively describe the hysteretic behavior of bentonite of water retention curves following wetting/drying cycles, which can be expressed as
×100% (2)
where wd and ww are the water contents in the drying and wetting paths at a given matric suction, respectively. Eq.(2) indicates that the hysteretic behaviour is less significant when η is small.
The hysteretic coefficients of unconfined compacted GMZ bentonite changing with suction at 20 and 40 ℃ were calculated using Eqn.(2). The results are given in Fig.6 (b). Fig.6(b) shows that the hysteretic coefficients are all less than 7%, and decrease as the temperature increases. , It seemswhich indicates that the hysteresis of highly- compacted bentonite is not so significant.
The hysteretic behavior of the unconfined compacted bentonite in different of ranges of suction at 40 ℃ is shown in Fig.7. Fig.7 (a) shows that the hysteretic cycle becomes larger with suction the decreases of suction. Fig.7(b) also indicates that hysteretic coefficients are lower than 5% at high suction (>4 MPa), and decrease with the increase of suction increases.
Fig.7 Hysteretic behaviors of unconfined compacted GMZ bentonite in different suction ranges of suction at 40 ℃ (B1 stands for hysteretic cycle inat high suction and B2 stands for hysteretic cycle inat low suction): (a) Hysteretic characteristics curves; (b) Relationship between suction and hysteretic coefficients
4 Conclusions
(1) The water retention capacity of the highly- compacted GMZ bentonite decreases as the temperature increases. At the same time, the impact of temperature on the water retention capacity has a close relationship with the suction.
(2) At a certain temperature, the influence of constraint conditions on the water retention curves capacity of the compacted bentonite is not obvious at high suction, but the water retention capacity of confined specimen is significantly lower than that of unconfined specimen at low suction.
(3) The hysteretic behaviour of the compacted bentonite under unconfined conditions decreases as the temperature increases. At high suction (>4 MPa), the hysteretic behaviour of the unconfined bentonite tends to increase with the suction decreasing suction. In summary, the hysteretic behaviour of compacted bentonite is not significant.
References
[1] WERSIN P, JOHNSON L H, MCKINLEY I G. Performance of the bentonite barrier at temperatures beyond 100 ℃: A critical review [J]. Physics and Chemistry of the Earth, 2007, 32(8/14): 780-788.
[2] ROMERO E, VILLAR M V, LLORET A. Thermo-hydro-mechanical behaviour of two heavily overconsolidated clays [J]. Engineering Geology, 2005, 81(3): 255-268.
[3] SA?NCHEZ M. Thermo-hydro-mechanical coupled analysis in low permeability media [D]. Barcelona: The Polytechnic University of Catalunya, 2004.
[4] VILLAR M V, LLORET A. Dismantling of the first section of the FEBEX in situ test: THM laboratory tests on the bentonite blocks retrieved [J]. Physics and Chemistry of the Earth, 2007, 32(8/14): 716-729.
[5] LLORET A, VILLAR M V. Advances on the knowledge of the thermo-hydro-mechanical behaviour of heavily compacted ‘‘FEBEX’’ bentonite [J]. Physics and Chemistry of the Earth, 2007, 32(8/14): 701-715.
[6] ROMERO E, GENS A, LLORET A. Temperature effects on the hydraulic behaviour of an unsaturated clay [J]. Geotechnical and Geological Engineering, 2001, 19(3/4): 311-332.
[7] VILLAR M V, LLORET A. Influence of temperature on the hydro-mechanical behaviour of a compacted bentonite [J]. Applied Clay Science, 2004, 26(1/4): 337-350.
[8] JACINTO A C, VILLAR M V, ROBERTO G E, LEDESMA A. Adaptation of the van Genuchten expression to the effects of temperature and density for compacted bentonites [J]. Applied Clay Science, 2009, 42(3-/4 ),): 575- -582.
[9] ALONSO E E. Gas migration through barriers [J]. Chinese Journal of Rock Mechanics and Engineering, 2006, 25(4): 693-708.
[10] PHAM H Q, FREDLUND D G, BARBOUR S L. A study of hysteresis models for soil-water characteristic curves [J]. Canadian Geotechnical Journal, 2005, 42(6): 1548-1568.
[11] LI X S. Modelling of hysteresis response for arbitrary wetting/drying paths [J]. Computers and Geotechnics, 2005, 32(2): 133-137.
[12] NUTH M, LALOUI L. Advances in modeling hysteretic water retention curve in deformable soils [J]. Computers and Geotechnics, 2008, 35(6): 835-844.
[13] LIU Y, ZHAO C G. Modeling the hysteresis for soil-water characteristic curves [C].// New Frontiers in Chinese and Japanese Geotechniques. Beijing: China Communications Press, 2007: 383--392.
[14] XU Yan-bing, WEI Chang-fu, CHEN Hui, LUAN Mao-tian. A model of soil-water characteristics for unsaturated geotechnical materials under arbitrary drying/wetting paths [J]. Chinese Journal of Rock Mechanics and Engineering, 2008, 27(5): 42-45. (in Chinese)
[15] LIU Yue-miao, WEN Zhi-jian. Study on clay-based materials for the repository of high level ratioactive waste [J]. Journal of Mineralogy and Petrology, 2001, 28(12): 19-21. (in Chinese)
[16] CHEN Bao, QIAN Li-xin, YE Wei-min, CUI Yu-jun, WANG Ju. Soil-water characteristic curves of Gaomiaozi bentonite [J]. Chinese Journal of Rock Mechanics and Engineering, 2006, 25(4): 788-793. (in Chinese)
[17] YE W M, SCHANZ T, QIAN L X, et al. Characteristics of swelling pressure of densely compacted Gaomiaozi bentonite GMZ01 [J]. Chinese Journal of Rock Mechanics and Engineering, 2007, 26(S2): 2861-3865. (in Chinese)
[18] QIN Bing, CHEN Zheng-han, LIU Yue-miao, WANG Ju. Swelling-shrinkage behaviour of Gaomiaozi bentonite [J]. Chinese Journal of Geotechnical Engineering, 2008, 30(7): 1005-1010. (in Chinese)
[19] CUI Y J, DELAGE P. Yielding and plastic behavior of an unsaturated compacted silt [J]. Geotechnique, 1996, 46(2): 291-311.
[20] YE Wei-min, TANG Yi-qun, CUI Yu-jun. Measurement of soil suction in laboratory and soil-water characteristics of Shanghai soft soil [J]. Chinese Journal of Geotechnical Engineering, 2005, 27(3): 347-349. (in Chinese)
[21] TANG A M, CUI Yu-jun. Controlling suction by the vapour equilibrium technique at different temperatures and its application in determining the water retention properties of MX80 clay [J]. Canadian Geotechnical Journal, 2005, 42: 1-10.
[22] LYTTON L. Isothermal water movement in clay soils [D]. Austn: University of Tekas, 1967.
Foundation item: Projects(40772180, 40572161, 40802064) supported by the National Natural Science Foundation of China; Project ([2007]831) supported by Commission of Science, Technology and Industry for National Defense of China; Project(07JJ4012) supported by Hunan Provincial Natural Science Foundation of China; Project(20080430680) supported by China Postdoctoral Science Foundation; Project(08R214155) supported by Shanghai Postdoctoral Scientific Program of China; Project(B308) supported by Shanghai Leading Academic Discipline Project of China
Received date: 2008-12-26; Accepted date: 2009-03-24
Corresponding author: YE Wei-min, PhD, Professor; Tel: +86-21-65985086; E-mail: ye_tju@tongji.edu.cn
- Effect of temperature on soil-water characteristics andhysteresis of compacted Gaomiaozi bentonite
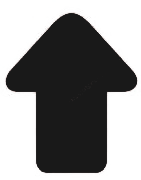