- Abstract:
- 1 Introduction▲
- 2 Materials and meth...▲
- 3 Results and discus...▲
- 3.1 Influence of different disinfection methods on E. Coli disinfection effects in outflow of wastewater treatment plant
- 3.2 Photoreactivation experiment of E. coli after UV-TiO2 disinfection
- 3.3 Photoreactivation experiment for E. faecalis after UV-TiO2 disinfection
- 3.4 Comparison of two kinds of different bacteria in UV-TiO2 disinfection effect
- 4 Conclusions▲
- References
- Figure
- Fig. 1 Experimental device
- Fig. 2 SEM image of filler
- Fig. 3 XRD pattern of filler
- Fig. 4 FITR spectrum of filler
- Fig. 5 Effect of disinfection method on E. coli disinfection
- Fig. 6 Effect of disinfection method and light intensity on photoreactivation of E. coli:
- Fig. 7 Effect of disinfection method and light intensity on photoreactivation of E. faecalis:
J. Cent. South Univ. (2016) 23: 3115-3121
DOI: 10.1007/s11771-016-3377-5
UV-TiO2 photocatalytic disinfection and photoreactivation of pathogenic bacterium in municipal wastewater
WANG Xi-feng(王西峰)1, HU Xiao-lian(胡晓莲)1, GONG Xin(龚昕)1, BAN Yun-xiao(班云霄)2
1. School of Civil Engineering, Hunan University of Science and Technology, Xiangtan 411201, China;
2. School of Environmental and Municipal Engineering, Lanzhou Jiaotong University, Lanzhou 730070, China
Central South University Press and Springer-Verlag Berlin Heidelberg 2016
Abstract:
The disinfected bacteria will be a photoreactivation under the irradiation of the sunlight, and the light intensity plays an important role in the bacteria resurrection. The effect of light intensity on photoreactivation of Escherichia coli (E. coli) and Enterococcus faecalis (E. faecalis) in secondary effluents which were disinfected respectively by pure UV and UV-TiO2 was investigated. The results show that the disinfection efficiency of UV-TiO2 is much higher than that of the pure UV disinfection. The photoreactivation rate of E. coli is much higher in pure UV disinfection than in UV-TiO2 photocatalytic disinfection. Under high light intensity in UV-TiO2 disinfection, high resurrection rate can be induced. However, a higher resurrection rate can be introduced even under low light intensity in pure UV disinfection alone. Meanwhile, UV-TiO2 disinfection has a strong inhibition effect on E. faecalis photoreactivation. When the light intensity is lower than 21 μW/cm2, nearly no resurrection of E. faecalis occurs after 72 h resurrection irradiation, and a little resurrection rate is observed only under a strong photoreactivating light intensity.
Key words:
1 Introduction
Disinfection is one of the important measures to ensure the safety of the water discharged from municipal wastewater treatment plant. As the ultraviolet disinfection is characterized by high efficiency, less byproducts and simple operation, this process is used most commonly in wastewater treatment plants. However, as the ultraviolet has no durability in the disinfection capability, the bacteria in the water which have been inactivated by the ultraviolet can revive for the second time [1-3]. Therefore, it is one of the hot issues in the current UV disinfection studies to improve the ultraviolet disinfection effect in the wastewater treatment plant and inhibit the photoreactivation of micro- organisms in water.
TiO2 photocatalytic oxidation is an advanced oxidation process. Its main principle can be described as follows: Under the action of ultraviolet (UV) energy, TiO2 can stimulate the generation of hydroxyl radical (×OH) with strong oxidation property; ×OH can oxidize refractory pollutants in water into inorganic small molecular substances without selection, such as water, CO2 and N2; moreover, ×OH has strong broad-spectrum disinfection capability against the bacteria in water [4-5]. The focus of this disinfection principle is that the bacteria are inactivated mainly through destroying the metabolism process of bacteria, genetic materials in the bacteria and structure of cell wall (or cell membrane) [6-7]. So, the combination of TiO2 photocatalytic oxidation and ultraviolet disinfection has become one of hot topics in the disinfection technology researches [8-9]. TiO2 photocatalytic reaction is usually based on the powdery catalyst. However, although the powdery catalyst has larger specific surface area and higher optical catalytic efficiency, it is usually difficult to recycle. In addition, it requires higher energy to be kept in the suspended state [10-11]; otherwise, its photocatalytic activity will decrease dramatically. As for TiO2 photo catalyst passivation, studies show that the basal material after acetic acid activation can obviously improve the load properties and photocatalytic activity of TiO2 catalyst, improve its ability to resist passivation, and prolong the service life of catalyst [12]. And through the cation doping, metal ions doping, precious metals doping and sensitization of the photosensitive material, these methods can also effectively improve the light efficiency of TiO2 photocatalyst [13-14].
In this work, using the fiber glass as the carrier of photocatalyst, TiO2 is loaded onto its surface to form a three-dimensional space photocatalytic reactor [15-17].Thus, the problem of TiO2 particles recycling can be resolved; meanwhile, as the fiber glass has large specific surface area, good transparency and stable physical and chemical properties, the large load capacity and high photocatalysis efficiency can be achieved. In addition, the optical energy can also be utilized rationally, improving the photocatalytic efficiency of TiO2.
On the basis of combining the glass fiber-loaded TiO2 catalytic oxidation and ultraviolet disinfection technology, two methods (pure UV disinfection and UV-TiO2) are used to disinfect the Escherichia coli (E. coli) and Enterococcus faecalis (E. faecalis). In addition, the photoreactivation process after the disinfection of E. coli and E. faecalis is studied, and the influence of UV-TiO2 photocatalytic disinfection on the photoreactivation capability of E. coli and E. faecalis in reclaimed water is analyzed.
2 Materials and methods
2.1 Materials
The strains of E. coli and E. faecalis were obtained from China General Microbiological Culture Collection Center. The E. coli strain was inoculated into lactose peptone broth for cultivation of 24 h at constant temperature to prepare the suspension of E. coli for experiment. The E. faecalis was inoculated into the EC broth tube for cultivation of (24±2) h in the water bath of (44.5±0.2) °C to prepare the suspension of E. faecalis for experiment. The bacterial suspension was frozen at the temperature of -80 °C for experiment.
The water sample was obtained from the outflow of secondary sedimentation tank of Xiangtan Hexi Wastewater Treatment Plant (Hunan Province of China). The water sample was filtered through the 45 μm filter paper to eliminate the influence of bacteria in water sample on experimental results. The dissolvedions and minerals in the aquatic environment were preserved as nutrients for microbe, and their effects on photocatalytic react were ignored. The melted bacterial suspension of E. coli and E. faecalis was respectively inoculated into the nutrient broth culture medium of 1000 mL with the amount of 100 mL. Then, they were placed in the constant temperature incubator shaker of 37 °C for cultivation of 24 h, and were centrifuged for 1 min at the rate of 10000 r/min. After the supernatant was removed, they were washed twice with sterilizing physiological saline solution and centrifuged repeatedly twice. After that, the sediments were dissolved in the water of secondary sedimentation tank, and the water sample of bacterial suspension with the initial concentration of 105 CFU/L was obtained, which was used to simulate the pathogenic bacteria in the outflow of secondary sedimentation tank of wastewater treatment plant. This water sample can be preserved for not more than 24 h.
2.2 Experimental device
As shown in Fig. 1, the experimental device was a homemade photocatalytic reactor. In the centre of reactor, there was an UV lamp sleeve, where the UV-light was provided by the low-pressure mercury lamp (UV365- 250W, Shanghai Jiguang Lighting Co. Ltd.). On the furthest exterior of it there was a casing for cooling water; in the middle there was a reaction tank, where the glass- fiber-loaded TiO2 photocatalytic fillers were packed. In order to compare the effect of TiO2 photocatalytic disinfection, the glass fiber without loading TiO2 was packed in the reactor when the pure UV disinfection was used. The power of the UV lamp was adjusted to obtain different UV intensity. The effective UV light intensity measured at the exterior diameter of reactor was taken as the irradiated light intensity. Before the experiment, the lamp was preheated for 0.5 h to stabilize the working status of UV lamp.
Fig. 1 Experimental device
2.3 Preparation of filler
The sol-gel method was used to prepare the glass-fiber-loaded TiO2 photocatalytic filler. Fibre glass yarn was soaked in absolute ethyl alcohol for 24 h, flushed with distilled water, and then heated up to 450 °C with a speed of 5-10 °C/min in muffle furnace and kept at this temperature for 2 h for heat treatment. Take some volume of tetrabutyl titanate, absolute ethyl alcohol, distilled water and nitric acid, mix tetrabutyl titanate and half of absolute ethyl alcohol to form A fluid. Mix another half of absolute ethyl alcohol, some distilled water, some nitric acid of 1:4 for concentration and some Fe(NO3)3 to form B fluid. Then, add B fluid into A fluid slowly under severe agitation, maintain this severe stirring for 2 h to make sol-gel liquid, and then still stand for 24 h for seasoning. Soak the treated fibre glass yarn into the seasoning sol-gel liquid for 2 h, take out of the liquid vertically, and dry at room temperature and ventilated environment. Put processed fibre glass yarn into muffle furnace, warm up to 550 °C with 5-10 °C/min heating speed, and keep at 550 °C for 3 h for calcine. Finally, cool it in a dry environment. Repeat this process three times and then get the photocatalytic filler.
The SEM, XRD and FTIR characterizations of the filler are shown in Figs. 2-4. Figure 3 shows that the TiO2 loaded on the surface of the glass fiber was the mixture of rutile and anatase crystal. And as shown in Fig. 4, the absorption peak at 502.8 cm-1 was the Ti-O-Si stretching vibration, the existence of Ti—O—Si keys means that the TiO2 was tightly bound to glass fiber, which guarantees that the catalyst will not come off from the carrier surface during the photocatalytic reaction. The specific surface area of filler test by BET was 0.1527 m2/g and the amount of TiO2 on the surface of glass fiber was 52.116 g/kg.
Fig. 2 SEM image of filler
Fig. 3 XRD pattern of filler
Fig. 4 FITR spectrum of filler
2.4 Disinfection experiment
The UV disinfection dose commonly used by the wastewater treatment plant is 15-22 mJ/cm2, which can meet the requirement that the total number of bacteria in the discharged water shall be less than 1000 CFU/L [18-19]. In order to make this experiment practically significant, the ultraviolet doses used in this study were 5 mJ/cm2 and 20 mJ/cm2 respectively. In common cases, the low UV dose of 5 mJ/cm2 cannot meet the requirement for pathogenic bacteria inactivation in the wastewater. In the photocatalytic reactor shown in Fig. 1, the absorbance of water sample under the wavelength of 254 nm was measured. The UV-A ultraviolet radiation meter was used to measure the UV intensity at the outer wall of the reactor, and the measured UV intensity was 0.24 mw/cm2. Under this intensity, the disinfection time was calculated under different ultraviolet doses; for the doses of 5 mJ/cm2 and 20 mJ/cm2, their disinfection time was 21 s and 83.3 s, respectively [17]. The disinfection time used in the experiment was 20 s and 85 s, respectively.
2.5 Photoreactivation experiment of bacteria after disinfection
The photoreactivation experiment after disinfection was conducted in a rectangular tank. The fluorescent lamp of 40 W was used to simulate the sunlight and adjust the distance between the lamp and the surface of water to obtain the different sunlight photoreactivation light intensity. And then, the photoreactivation of bacteria in the water was studied at the wavelength of 365 nm and the photoreactivation light intensity of 9, 21 and 41 μW/cm2, respectively. The bacterial concentration was taken as the objective of this study. In the experiment, the impact of micro-organisms in the air on the experimental results was not considered.
2.6 Method of counting bacteria
Plate count was used for bacteria counting. A certain amount of water sample diluted and the melted nutrient agar culture medium were mixed. After the mixture was shaken, it was placed in the incubator of 37 °C for 24 h. And then, the number of bacterial colonies on the plate was calculated to determine the bacteria concentration in the water [18]. For the same water sample, three parallel samples were prepared.
3 Results and discussion
3.1 Influence of different disinfection methods on E. Coli disinfection effects in outflow of wastewater treatment plant
The methods of pure UV disinfection and UV-TiO2 photocatalytic disinfection are used for water sample. Under the condition of UV doses of 5 mJ/cm2 and 20 mJ/cm2, the disinfection effect of E. coli in the water sample is shown in Fig. 5.
Fig. 5 Effect of disinfection method on E. coli disinfection
As shown in Fig. 5, when the UV dose is 5 mJ/cm2, the number of E. coli in the water after the disinfection is 7600 CFU/L and the inactivation rate is 96%. However, after the method of UV-TiO2 photocatalytic disinfection, the number of E. colis in the water is only 734 CFU/L and the inactivation rate is 99.7% under the low UV dose of only 5 mJ/cm2. In addition, it can also be seen from the figure that, under the condition of UV-TiO2 disinfection, when the UV doses are 5 mJ/cm2 and 20 mJ/cm2, respectively, the numbers of E. coli in water are 734 CFU/L and 176 CFU/L, and the inactivation rates are 99.7% and 99.9%, respectively. At the dose of 20 mJ/cm2, the number of E. coli after the pure UV disinfection is 620 CFU/L, the inactivation rate is lower than that under UV-TiO2 combined disinfection. The disinfection conclusion of E. faecalis is similar to that of E. coli: the effect of UV-TiO2 combined disinfection is better than that of pure UV disinfection. Therefore, the use of UV-TiO2 can bring about the saving in energy consumption in the disinfection and better effect in pathogenic bacteria inactivation. Great difference in disinfection effect lies in the difference in disinfection principle. In the process of pure UV disinfection, the UV light can penetrate the cell body and destroy the molecular structure of DNA or RNA within the microbial cells, resulting in the death of growing cells or destruction of cell reproduction performance; or the energy of UV-light is used to trigger the denaturation of protein in the body of microorganism, enabling the enzyme system in the cell to lose its role and result in the death of cells [20-22]. In the process of UV-TiO2 disinfection, the inactivation of bacteria mainly depends on the combined effect of UV disinfection and advanced oxidation disinfection. First, the UV-light can inactivate the microorganisms in the water; meanwhile, under the trigger action of UV, the ·OH which has strong oxidation function is generated on the TiO2 surface to oxidize the coenzyme A in the microbial cell, preventing the respiration of microorganism and causing the death of microorganism. Hydroxyl radicals are very small particles and they can be easy to penetrate inside the microbial cell, destroying the penetration of bacteria in the cell wall and causing the death of microorganisms because of electrolyte imbalance. In addition, the strong oxidizing property of the ×OH enables the protein in the cell to change its nature, making the enzyme system in the microbial cell lose its role. Finally, the ·OH can directly oxidize the microbial cells to inorganic substances, such as CO2, H2O, causing the death of microorganisms [7, 20]. So, the efficiency of UV-TiO2 combined disinfection is much higher than that of pure UV disinfection.
3.2 Photoreactivation experiment of E. coli after UV-TiO2 disinfection
The water samples containing E.coli after the pure UV disinfection and UV-TiO2 photocatalytic disinfection at the dose of 20 mJ/cm2 were respectively placed under different reactivation light intensities for irradiation. Thus, the photoreactivation information of bacteria can be obtained. The experiment results are shown in Fig. 6.
As shown in Fig. 6, in the water samples after the pure UV disinfection and UV-TiO2 photocatalytic disinfection, the photoreactivation capabilities of E. coli are different. After the UV-TiO2 disinfection, the photoreactivation capability of E. coli declines obviously. Its photoreactivation capability varies with the intensity of light for reactivation. Under the condition of pure UV disinfection, the photoreactivation phenomenon in the water sample is obvious under the light intensity of 9, 21 and 41 μW/cm2. Moreover, the higher the light intensity is, the quicker the photoreactivation of E. coli is. The main reason is that, under the stronger light intensity, the photoreactivation accelerates when the energy received by the bacteria for restoring the damaged cell structure becomes higher and higher [23-24]. This phenomenon shows that, to the slightly damaged cell structure, E. coli can use less light energy to complete the reactivation process. The similar conclusion can be found in the related researches [25-26]. However, when the UV-TiO2 photocatalytic disinfection method is used, the photoreactivation rates of E. coli are obviously different under different light intensities. As seen in Fig. 6(b), the total number of bacteria in the water changes little after the UV-TiO2 disinfection of 20 mJ/cm2 under the irradiation of reactivation light with the lower intensity of 0 and 9 μW/cm2. After the first 12-h irradiation, the bacterial in the water are still in a state of equilibrium. The possible reason is that UV-TiO2 photocatalytic disinfection dramatically damages the structures of bacterial cell. Thus, under the irradiation of reactivation light with weaker intensity, the damaged cells are difficult to recover; even for E. coli with minor damage, it also goes through a long recovery period under the condition of low irradiation. After 72-h irradiation, the total number of E. coli is still less than 1000 CFU/L. Only under the irradiation of reactivation light with the intensity of 41 mJ/cm2, can the damaged cells quickly recover under the high-energy supplement, and the E. coli achieves an obvious photoreactivation.
Fig. 6 Effect of disinfection method and light intensity on photoreactivation of E. coli:
The UV-TiO2 photocatalytic disinfection can significantly inhibit the photoreactivation speed of E. coli. As seen from Fig. 6, in the case of pure UV disinfection, within the first 12 h of reactivation light irradiation, the photoreactivation speed of E. coli under the light intensity of 9, 21 and 41 μW/cm2 varies little. However, for the water sample after the UV-TiO2 photocatalytic disinfection, the photoreactivation speed of E. coli is almost zero within the first 12 h under the irradiation of light with the intensity less than 9 μW/cm2. Only under the irradiation of light with the intensity more than 21 μW/cm2, can the photoreactivation rate of E. coli be relatively higher.
This result also shows that the UV-TiO2 photocatalytic disinfection can not only inactivate E. coli effectively, but also inhibit the photoreactivation capability of E. coli.
3.3 Photoreactivation experiment for E. faecalis after UV-TiO2 disinfection
The water samples containing E. faecalis were disinfected through using the pure UV disinfection method and UV-TiO2 photocatalytic disinfection method at the dose of 20 mJ/cm2. The photoreactivation experimental results of water samples after the disinfection are shown in Fig. 7.
Fig. 7 Effect of disinfection method and light intensity on photoreactivation of E. faecalis:
From Fig. 7, it can be seen that the photoreactivation of E. faecalis in the water sample is similar to that of E. coli. That is, under the irradiation of reactivation light, the reactivation phenomenon occurs to varying degree. But differently, E. faecalis is higher than E. coli in the tolerance of UV disinfection. In the case of pure UV disinfection, under the reactivation light with the lower and even lower to zero intensity, the more obvious photoreactivation phenomenon also occurs to E. faecalis after 12 h. Under the intensity of 0, 9, 21 and 41 μW/cm2, the average photoreactivation speed of E. faecalis after 2 h irradiation is 21, 41, 58 and 98 CFU/(L·h) respectively, which shows that the photoreactivation speed of E. faecalis increases with the increase in the intensity of reactivation light.
In the case of UV-TiO2 disinfection, the photoreactivation rate of E. faecalis decreases dramatically. Under the joint action of the hydroxyl radicals (×OH) and ultraviolet light, the photoreactivation rate of E. faecalis is far less than that under the condition of pure UV disinfection. As shown in Fig. 7(b), under the irradiation of reactivation light with the dose of less than 21 μW/cm2, the phenomenon of photoreactivation hardly occurs to E. faecalis. Only when the light intensity increases to 41 μW/cm2, can E. faecalis produce a lower level of reactivation. In the process of reactivation light irradiation, the highest concentration of E. faecalis appears between 8 and 24 h and the highest concentration is 580 CFU/L and the maximum reactivation speed is 65 CFU/(L·h). The reactivation rate is far less than that under the condition of pure UV disinfection, which shows that the efficiency of UV-TiO2 photocatalytic disinfection is far higher than that of pure UV disinfection.
3.4 Comparison of two kinds of different bacteria in UV-TiO2 disinfection effect
From the above results, it can be seen that E. coli and E. faecalis, two common pathogens, are considerably different in the sensitivity to UV-TiO2 photocatalytic disinfection. E. coli has strong resistance against UV-TiO2 photocatalytic disinfection and its photoreactivation has no threshold. Under the lower intensity of reactivation light, the photoreactivation phenomenon can occur to a certain degree. The photoreactivation rate of E. coli increases with the increase in the intensity of reactivation light. This shows that E. coli has stronger photoreactivation capability [19, 27-29]. But the UV-TiO2 disinfection can effectively inactivate E. coli. After the UV-TiO2 disinfection, the total number of E. coli in water is still less than 1000 CFU/L even if being irradiated for 72 h by the reactivation light with the stronger intensity of 41 μW/cm2, which shows that the UV-TiO2 photocatalytic disinfection has strong disinfection capability [30-31].
E. faecalis has weaker tolerance against the UV-TiO2 disinfection. After the UV-TiO2 disinfection, the photoreactivation of E. faecalis has an obvious light intensity threshold [32-33]. Only when the light intensity reaches 21 μW/cm2 or more, can the more obvious photoreactivation phenomenon appear, but the photoreactivation rate is still very lower. If the intensity of reactivation light is lower than the threshold, no obvious photoreactivation phenomenon appears for E. faecalis.
4 Conclusions
The efficiency of UV-TiO2 photocatalytic disinfection is much higher than that of pure UV disinfection. For the UV-TiO2 photocatalytic disinfection method, the total bacteria number of E. coli and E. faecalis in water will be less than 1000 CFU/L even under the irradiation of UV light with the low dose of 5 mJ/cm2. After the UV-TiO2 disinfection, to E. coli and E. faecalis, the photoreactivation phenomenon can occur only under the condition of strong light irradiation, but the total number of E. coli or E. faecalis is still lower than 1000 CFU/L even if being irradiated for 72 h under the light with the strong intensity of 41 μW/cm2. This shows that the UV-TiO2 photocatalytic disinfection not only has strong disinfection capability, but also can inhibit the photoreactivation of bacteria after disinfection. After the UV-TiO2 photocatalytic disinfection, the sensitivity of E. coli and E. faecalis to reactivation light intensity is different. The UV-TiO2 disinfection can effectively inhibit the photoreactivation of E. faecalis, and its photoreactivation has a threshold. If the reactivation light intensity is lower than this threshold, the reactivation phenomenon hardly occurs to E. faecalis. But for E. coli, as its sensitivity to reactivation light intensity is poorer, it can also generate a small amount of photoreactivation even under the irradiation of reactivation light with lower intensity.
References
[1] RAJALA-MUSTONEN R L, TOIVOLA P S, HEINONEN-TANSKI H. Effects of peracetic acid and UV irradiation on the inactivation of coliphages in wastewater [J]. Water Science & Technology, 1997, 35(11/12): 237-241.
[2] SANZ E N, I S, BALAO J A A, ALONSO J M Q. Modelling of reaction after UV disinfection: Effect of UV-C dose on subsequent photoreactivation and dark repair [J]. Water Research, 2007, 41(14): 3141-3151.
[3] SAKAI H, OGUMA K, KATAYAMA H, OHGAKI S. Effects of low or medium-pressure UV irradiation on the release of intracellular microcystin [J]. Water Research, 2007, 41(15): 3458-3464.
[4] MILLS A, HUNTE S L. A over view of semiconductor photocatalysis [J]. J Photochem Photobiol A: Chem, 1997, 108(1): 1-35.
[5] THOMSON T L, YATES J T. Surface science studies of the photoactivation of TiO2-New photochemical processes [J]. Chemical Reviews, 2006, 106(10): 4428-4453.
[6] TADASHI M, RYOZO T, TOSHIAKI N. Photoeletro chemical sterilization of microbial cells by semiconductor powders [J]. FEMS Microbiology Letters, 1985, 29(1/2): 211-214.
[7] LIBERTI L, LOPEZ A, NOTARNICOLA M, BARNEA N, PEDAHZUR R, FATTAL B. Comparison of advanced disinfecting methods for municipal wastewater reuse in agriculture [J]. Water Science & Technology, 2000, 42(1/2): 215-220.
[8] KIKUCHI Y, SUNADA K, IYODA T, HASHIMOTO K, FUJISHIMA A. Photocatalytic bactericidal effect of TiO2 thin film: Dynamic view of the active oxygen species responsible for the effect [J]. J Photochem Photobiol A: Chem, 1997, 106(2): 51-56.
[9] SUNADA K, WATANABE T, HASHIMOTO K. Studies on photo killing of bacteria on TiO2 thin film [J]. J Photochem Photobiol A: Chemi, 2003, 156(1/2/3): 227-233.
[10] MATSUMOTO T, IYI N, KANEKO Y, KITAMURA K, ISHIHARA S, TAKASU Y, MURAKAMI Y. High visible-light photocatalytic activity of nitrogen-doped titania prepared from layered titania/isostearate nanocomposite [J]. Catal Today, 2007, 120(2): 226-232.
[11] HOFFMANN M R, MARTIN S T, CHOI W, BAHNEMANN D W. Environmental applications of semiconductor photocatalysis [J]. Chem Rev, 1995, 95(1): 69-96.
[12] LI Zhang, ZHANG Peng-yi, CHEN Song-zhe. Influence of pretreatment of titanium substrate on long-term stability of TiO2 film [J]. Chinese Journal of Catalysis, 2007, 28(4): 299-306.
[13] de JONG K P, GEUS J W. Carbon nanofibers: Catalytic synthesis and applic ations [J]. Catalysis Reviews, 2000, 42(4): 481-510.
[14] YUE Ling-hai, SHUI Miao, XU Zhu-de, ZHENG Yi-fan. The A-R transformation and photocatalytic activities of mixed TiO2 rare earth oxides [J]. Journal of Zhe jiang University: Sciences Edition, 2000, 27(1): 69-74.
[15] AO C H, LEE S C, JIMMY YU C. Photocatalyst TiO2supported on glass fiber for indoor air purification: Effect of NO on the photodegradation of CO and NO2 [J]. J Photochem Photobiol A: Chem, 2003, 156(1/2/3): 171-177.
[16] UBOLCHONLAKATE K, SIKONG L, TONTAI T. Formaldehyde degradation by photocatalytic Ag-doped TiO2 film of glass fiber roving [J]. J Nanosci Nanotechnol,2010, 10(11): 7522-7525.
[17] SAKAI S, HIRAOKA K, YAMASHITA R, MATSUMOTO Y. Effect of TiO2photocatalyst on odorous gases using glass fiber filter for repeatable use [J]. Japanese Journal of Applied Physics, 2006, 45: 8-11.
[18] FARIBORZ T. Ultraviolet and ionizing radiation for microorganism inactivation [J]. Water Res, 2004, 38(6): 3940-3948.
[19] HASSEN A, MAHROUK M, OUZARI H, CHERIF M, BOUDABOUS A, DAMELINCOURT J J. UV disinfection of treated wastewater in a large-scale pilot plant and inactivation of selected bacteria in a laboratory UV device [J]. Bioresource Technology, 2000, 74: 141-150.
[20] BOLTON J R, LINDEN K G. Standardization of methods for fluence (UV Dose) determination in bench-scale UV experiments [J]. Journal of Environmental Engineering, 2003, 129(3): 209-216.
[21] VAN A B, LIN L S. Effect of the disinfection agents chlorine, UV irradiation, silver ions, and TiO2 nanoparticles/near-UV on DNA molecules [J]. Water Science and Technology,2011, 64(6): 1226-1232.
[22] KASHIMADA K, KAMIKO N, YAMAMOTO K, OHGAKI S. Assessment of photoreactivation following ultraviolet light disinfection [J]. Water Science and Technology, 1996, 33(10/11): 261-269.
[23] IRELAND J C, KLOSTERMANN P, RICE E W, CLARK R M. Inactivation of Escherichia coli by titanium dioxide photo-catalytic oxidation [J]. Applied and Environmental Microbiology, 1993, 59(5): 1668-1670.
[24] LIN C H, YU R F,CHENG W P,LIU C R. Monitoring and control of UV and UV-TiO2disinfections for municipal wastewater reclamation using artificial neural net works [J]. Journal of Hazardous Materials, 2010, 209/210: 348-354.
[25] OGUMA K, KATAYAMA H, OHGAKI S. Photoreactivation of Escherichia coli after low-or medium-pressure UV disinfection determined by an endonuclease sensitive site assay [J]. Applied and Environmental Microbiology, 2002, 68(12): 6029-6035.
[26] JIN X, LI Z F, ZHAO X, XIE L L, WANG T T. Effect of ultrasound pre-treatment on ultraviolet disinfection in controlling bacterial photoreactivation [J]. Advanced Materials Research, 2011, 347-353: 2369-2374.
[27] RAHMANI A, SAMARGHANDI M R, SAMADI M T, NAZEMI F. Photocatalytic disinfection of coliform bacteria using UV/TiO2 [J]. Journal of Research in Health Sciences, 2009, 9(1): 1-6.
[28] EDE S, HAFNER L, DUNLOP P, BYRNE J, WILL G. Photocatalytic disinfection of bacterial pollutants using suspended and immobilized TiO2 powders [J]. Photochemistry and Photobiology, 2012, 88(3): 728-735.
[29] NATH R K, ZAIN M F M, KADHUM A A H. Photocatalysis—A novel approach for solving various environmental and disinfection problems: A brief review [J]. Journal of Applied Sciences Research, 2012, 8(8): 4147-4155.
[30] SHANG C, CHEUNG L M, HO C M, IENG M. Repression of photoreactivation and dark repair of coliform bacteria by TiO2-modified UV-C disinfection [J]. Applied Catalysis B: Environmental, 2009, 89(3/4): 536-542.
[31] LOCAS A, DEMERS J, PAYMENT P. Evaluation of photoreactivation of Escherichia coli and Enterococci after UV disinfection of municipal wastewater [J]. Canadian Journal of Microbiology, 2008 , 54(11): 971-975.
[32] MOUNAOUER B, SAIDI N, ABDENNACEUR H. UV disinfection of secondary wastewater: Influence of photoreactivation on the kinetics of inactivation of Pseudomonas aeruginosa [J]. Current Biotechnology, 2012, 1(4): 346-357.
[33] CHOI Y S,KIM B W. Photocatalytic disinfection of E coliin a UV/TiO2-immobilised optical-fibre reactor [J]. Journal of Chemical Technology and Biotechnology, 2000, 75(12): 1145-1150.
(Edited by YANG Hua)
Foundation item: Projects(51174090, 51168026) supported by the National Natural Science Foundation of China
Received date: 2015-06-08; Accepted date: 2016-01-18
Corresponding author: WANG Xi-feng, Associate Professor, PhD; Tel: +86-13100329098; E-mail: wxfwk88@163.com
Abstract: The disinfected bacteria will be a photoreactivation under the irradiation of the sunlight, and the light intensity plays an important role in the bacteria resurrection. The effect of light intensity on photoreactivation of Escherichia coli (E. coli) and Enterococcus faecalis (E. faecalis) in secondary effluents which were disinfected respectively by pure UV and UV-TiO2 was investigated. The results show that the disinfection efficiency of UV-TiO2 is much higher than that of the pure UV disinfection. The photoreactivation rate of E. coli is much higher in pure UV disinfection than in UV-TiO2 photocatalytic disinfection. Under high light intensity in UV-TiO2 disinfection, high resurrection rate can be induced. However, a higher resurrection rate can be introduced even under low light intensity in pure UV disinfection alone. Meanwhile, UV-TiO2 disinfection has a strong inhibition effect on E. faecalis photoreactivation. When the light intensity is lower than 21 μW/cm2, nearly no resurrection of E. faecalis occurs after 72 h resurrection irradiation, and a little resurrection rate is observed only under a strong photoreactivating light intensity.
- UV-TiO2 photocatalytic disinfection and photoreactivation of pathogenic bacterium in municipal wastewater
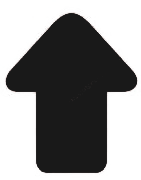