- Abstract:
- 1 Introduction▲
- 2 Test program▲
- References
- Figure
- Fig.1 Geometry and reinforcement details of specimens (unit: mm)
- Fig.2 Experimental setup for fire test
- Fig.3 Experimental setup for cyclic loading test
- Fig.4 Final failure modes of specimens: (a) N4T0; (b) N4T0-D; (c1) N0T9, unexposed side; (c2) N0T9, exposed side; (d1) N4T9, unexposed side; (d2) N4T9, exposed side; (e1) N4T9-D, unexposed side; (e2) N4T9-D, exposed side
- Fig.5 Hysteretic curves of lateral load- displacement for different specimens: (a) N4T0; (b) N0T9; (c) N4T9; (d) N4T0-D; (e) N4T9-D
- Fig.6 Skeleton curves of lateral load-displacement for different specimens
- Fig.7 Curves of secant stiffness-top horizontal displacement for different specimens
J. Cent. South Univ. Technol. (2010) 17: 1103-1108
DOI: 10.1007/s11771-010-0603-4
Post-fire cyclic behavior of reinforced concrete shear walls
LIU Gui-rong(刘桂荣)1, 2, SONG Yu-pu(宋玉普)1, QU Fu-lai(曲福来)2
1. State Key Laboratory of Costal and Offshore Engineering, Dalian University of Technology, Dalian 116024, China;
2. School of Civil Engineering and Communication,
North China Institute of Water Conservancy and Electric Power, Zhengzhou 450011, China
? Central South University Press and Springer-Verlag Berlin Heidelberg 2010
Abstract:
The effects of fire exposure, reinforcement ratio and the presence of axial load under fire on the seismic behavior of reinforced concrete (RC) shear walls were investigated. Five RC shear walls were tested under low cyclic loading. Prior to the cyclic test, three specimens were exposed to fire and two of them were also subjected to a constant axial load. Test results indicate that the ultimate load of the specimen with lower reinforcement ratio is reduced by 15.8% after exposure to elevated temperatures. While the reductions in the energy dissipation and initial stiffness are 59.2% and 51.8%, respectively, which are much higher than those in the ultimate load. However, this deterioration can be slowed down by properly increasing reinforcement due to the strength and stiffness recovery of steel bars after cooling. In addition, the combined action of elevated temperatures and axial load results in more energy dissipation than the action of fire exposure alone.
Key words:
shear wall; reinforced concrete; post-fire; seismic behavior; low cyclic loading;
1 Introduction
Reinforced concrete (RC) structures can be repaired properly to resume service if the fires are put out in time [1]. To propose effective and economical repair plan, it is essential to investigate the post-fire behaviors of structural material and elements. Numerous investigations were carried out to study the residual mechanical properties of concrete [2-3], rebar [4] and concrete-rebar interface after exposure to elevated temperatures [5-6]. Some researchers performed studies on the post-fire behavior of RC members. EI-HAWARY et al [7-8] investigated the flexural and shear behaviors of 12 RC beams after being exposed to elevated temperatures and cooled by water. CHEN et al [9] studied the residual strength and stiffness in axial load combined with uniaxial or biaxial bending of nine full-size RC columns after being exposed to different fire durations. WU et al [10] tested four RC columns in a combination of constant axial force and lateral cyclic loading after high temperatures. XIAO et al [11] tested four single-span and single-storey high performance concrete (HPC) frames to investigate their fire response and post-fire seismic performance. It can be concluded that the mechanical properties of structural materials are deteriorated by elevated temperature, which leads to the degradation of load capacity as well as seismic performance of structural elements.
RC shear walls were widely used to resist the lateral force mainly induced by the earthquake, especially in high-rise structures. Consequently, extensive studies on the seismic behavior of RC shear walls at normal temperature were conducted globally [12-14]. It is noted that shear walls, especially in the high-rise buildings are also used as the fire-resistance wall. Therefore, it is very instructive to investigate the post-fire seismic behavior of RC shear walls. XIAO et al [15] tested four HPC shear walls under low frequency cyclic loading to investigate the effect of fire exposure and polypropylene fiber on the seismic behavior of shear walls.
However, the research on the post-fire seismic performance of RC shear walls needs to be conducted further because the relevant research is insufficient. In this work, an experimental study on the seismic behavior of RC shear walls after being exposed to fire was presented.
2 Test program
2.1 Specimen details
Five shear walls were tested in the research program. All the specimens had equal dimensions, cast with 100 mm×100 mm embedded columns. Fig.1 shows the dimensions of specimens together with the reinforcement details. At the top of the specimen, an upper beam was
Fig.1 Geometry and reinforcement details of specimens (unit: mm)
cast monolithically with the wall to facilitate the application of the external axial and lateral loads. At the bottom of the specimen, a lower beam was cast to clamp down the specimen to the laboratory floor for simulating a rigid foundation.
2.2 Fire test
Specimens N0T9, N4T9 and N4T9-D were subjected to elevated temperatures by combustion of the liquefied petroleum gas. These three shear walls were tested at a horizontal position, exposed to fire on one side (Fig.2). Meanwhile, specimens N4T9 and N4T9-D were subjected to an invariant axial load equal to 400 kN during the fire test, simulating the service vertical loads from roof or higher storey. Fire was extinguished after the specimen was heated for 90 min. The average value of the maximum concrete temperatures measured at the location of 5 mm from exposed surface was about 500 ℃.
Fig.2 Experimental setup for fire test
2.3 Low cyclic load test
All the specimens were subjected to a combination of constant axial and cyclic lateral loads exerted by the test rig shown in Fig.3. A constant axial load of 400 kN was first applied to the top of the specimen, which
Fig.3 Experimental setup for cyclic loading test
corresponded to 0.31 of the uniaxial compressive strength of the wall cross section at ambient temperature. Then, the lateral cyclic load was applied by two hydraulic jacks that were operated alternately. The full lateral loading history of the test program contained the loading controlled and displacement controlled stages. Before yielding, one cycle was imposed at each selected load level. In contrast, after yielding, three cycles were applied at the integer multiples of the yield displacement till the wall failed. This experimental program defined failure as the point on the loading curve where the lateral load was reduced to 85% of the peak load previously recorded.
3 Test results3.1 Failure mode of specimens
A view of specimens at failure is given in Fig.4. For shear walls (N4T0 and N4T0-D) unexposed to fire, the phenomena at failure on both sides are basically similar. In contrast, it is quite different for those exposed to fire. There is an obvious concrete exfoliation on the exposed surface since higher temperature leads to more reduction in the strength of concrete.
From Fig.4, it can be seen that one or several obvious critical inclined cracks are observed for specimens N4T0, N4T0-D (without fire exposure) and N0T9 (with fire exposure individually) at failure. In contrast, for specimens N4T9 and N4T9-D (with the combined action of fire and axial load), the width of inclined cracks is small at failure and the principle diagonal cracks are indistinct. This phenomenon may be explained by the following reasons.
(1) Elevated temperatures reduce the strength of concrete, but they enhance the ductility of concrete.
(2) There are random micro-cracks in the specimens and visible diagonal cracks on the unexposed side under
Fig.4 Final failure modes of specimens: (a) N4T0; (b) N4T0-D; (c1) N0T9, unexposed side; (c2) N0T9, exposed side; (d1) N4T9, unexposed side; (d2) N4T9, exposed side; (e1) N4T9-D, unexposed side; (e2) N4T9-D, exposed side
the combined action of elevated temperature and axial load. During the low cyclic test, the continuous propagation of existing microscopic and macroscopic cracks leads to the disappearance of obvious principle diagonal crack.
3.2 Lateral load capacity
Table 1 lists the main results of all test specimens. The results show that the lower the reinforcing steel ratio, the higher the ultimate load reduction rate after the same fire exposure. The ultimate load of specimen with lower reinforcement is about 15.8% smaller than that of unfired values. Whereas, the reduction is about 7.0% for specimens with higher reinforcement after the same fire exposure. This can be attributed to the strength recovery of steel bars after cooling. Temperatures up to 600 ℃ have no significant effect on residual steel strength after cooling in air, which reduces no more than 10% of the unheated value [4]. Consequently, the strength recovery of rebar can slow down the deterioration in resistance of reinforced concrete specimens. Also, it makes more ultimate load rise after fire than at normal temperature with the same increase of reinforcement. In this test, the ultimate loads are increased by 1.7% and 12.4% at normal temperature and after fire exposure, respectively.
Furthermore, specimens N0T9 and N4T9 exhibit the same horizontal load-bearing capacity, which indicates that the presence or absence of axial load under fire does not affect the resistance of the RC shear wall after fire.
3.3 Hysteretic curves
The lateral load-displacement hysteretic curves are shown in Fig.5. Comparing the curves of all the specimens, the following conclusions can be drawn.
(1) The hysteretic loops of the specimens exposed to fire are “thinner” and the number of them is less than that of the unheated walls. This implies that fire exposure decreases the energy dissipation and deformation capacity, hence resulting in a reduction in the seismic resistance.
(2) The hysteretic loops are relatively full for shear walls with higher steel ratio. And this distinction is more prominent for specimens exposed to fire, which proves that the increase of reinforcement can alleviate the damage of fire exposure.
(3) The fullness and number of the hysteretic loops of specimen N4T9 show a little decrease compared with those of specimen N0T9, indicating a little more damage of combined action of axial load and elevated temperatures.
3.4 Skeleton curves
The skeleton curves of all specimens are shown in Fig.6. Comparing the skeleton curve of N4T0 with that
Table 1 Summary of properties and test results of walls
Fig.5 Hysteretic curves of lateral load- displacement for different specimens: (a) N4T0; (b) N0T9; (c) N4T9; (d) N4T0-D; (e) N4T9-D
of N4T9, the ductile behavior for the former is evidently characterized by relatively long yield point elongation. Besides, the load capacity and secant stiffness of specimens N4T9 are lower than those of N4T0. This also reflects the detrimental effect of fire exposure on the seismic performance of shear walls.
Fig.6 Skeleton curves of lateral load-displacement for different specimens
The skeleton curves of N4T0 and N4T0-D are superposed practically, which indicates that an increase of reinforcement has almost no effect on skeleton curves of the unheated specimens. However, for specimens exposed to fire, N4T9-D has higher bearing capacity and stiffness compared with N4T9. Moreover, there is a longer yield point elongation for the former, indicating better plastic deformation capacity. This also reveals that the increase of reinforcement has a good effect on the seismic performance of the fired shear walls.
3.5 Energy dissipation
Cumulated hysteretic energy, as a parameter that reflects the dissipating energy capacity, is commonly quantified by determining the total area under the load-displacement hysteretic loops. The cumulated hysteretic energy of all test specimens is denoted as Ep and listed in Table 1. As described in section 3.3, the heated walls exhibit hysteretic characteristics of “thinner” and less loops, and hence less energy dissipation, than the unheated ones. The cumulated hysteretic energy of specimen N4T9 drops by 59.2% as compared with that of N4T0. Similarly, specimen N4T9-D shows a reduction of 33.8% as compared with N4T0-D. Obviously, the decrease rate is higher for shear walls with lower reinforcement. Furthermore, the cumulated hysteretic energy of specimens N4T0-D and N4T9-D is improved by 6.2% and 72.4% compared with that of their low reinforcement equivalents. This reveals that the increase of reinforcement is beneficial in terms of the energy dissipation, especially for specimens exposed to fire. Besides, the cumulated energy dissipation of N4T9 is about 70% of that of N0T9. This reflects that, compared with individual fire exposure, the combination of fire exposure and axial load results in more energy dissipation reduction due to more damage.
Fig.7 Curves of secant stiffness-top horizontal displacement for different specimens
3.6 Stiffness
Fig.7 illustrates the variation of average secant stiffness with horizontal displacement of the specimens under successive cyclic loadings. It is clear that for every test specimen, there is a considerable reduction of the stiffness at the beginning of the loading, while the stiffness declines slowly after yielding.
The initial stiffness of N4T9 is about 51.8% less than that of N4T0, whereas the value of N4T9-D is 38.5% less than that of N4T0-D. This indicates that fire exposure significantly reduces the initial stiffness of the shear wall, but this reduction can be slowed down by properly increasing reinforcement. This can be explained as follows. The elastic modulus of concrete falls off steeply after high temperature and being cooled in air, whereas the residual elastic modulus of rebar is almost insensibly changed at temperatures up to 500 ℃. Consequently, the elastic modulus recovery of rebar results in more contribution to the stiffness of shear walls after fire exposure than at ambient temperature. A certain increase of reinforcement can slow down the stiffness deterioration of specimens by fire exposure. In addition, there is no significant difference in the stiffness- displacement curves of N4T9 and N0T9, which indicates that the presence of axial load under fire condition has little or no effect on the stiffness of shear walls after fire.
4 Conclusions(1) Fire exposure reduces the ultimate load of the RC shear wall, notably drops down the energy dissipation and initial stiffness. Consequently, much attention should be paid to the seismic and deformation capacity of RC shear walls after fire.
(2) Due to the strength and elastic modulus recovery of steel bars after cooling, a certain increase of reinforcement can decrease the rates of reduction in the ultimate load, energy dissipation capacity and stiffness. As the reinforcement ratio increases from 0.44% to 0.66%, the reductions in the ultimate load, energy dissipation and initial stiffness are decreased by 8.8%, 25.4% and 13.3%, respectively. Thus, shear walls that are used to resist both seismic action and fire attack are suggested the addition of reinforcement.
(3) The presence or absence of axial load under fire condition has almost no effect on the load-bearing capacity and stiffness of shear walls after fire exposure. However, the presence of axial load under fire results in more energy dissipation reduction of shear walls after fire exposure.
References[1] LIN C H, CHEN S T, YANG C A. Repair of fire-damaged reinforced concrete columns [J]. ACI Materials Journal, 1995, 92(4): 406-411.
[2] CHANG Y F, CHEN Y H, SHEU M S, YAO G C. Residual stress-strain relationship for concrete after exposure to high temperatures [J]. Cement and Concrete Research, 2006, 36(10): 1999-2005.
[3] LEE J, XI Y, WILLAM K. Properties of concrete after high-temperature heating and cooling [J]. ACI Materials Journal, 2008, 105(4): 334-341.
[4] NEVES I C, RODRIGUES J P C, LOUREIRO A P. Mechanical properties of reinforcing and prestressing steels after heating [J]. Journal of Materials in Civil Engineering, 1996, 8(4): 189-194.
[5] WANG Kong-fan, XU Qing-feng, LIU Ting-lin. Experimental research on bonding strength between concrete and steel bar after cooled down from high temperature [J]. Construction Technology, 2005, 34(8): 6, 11. (in Chinese)
[6] HADDAD R H, SHANNIS L G. Post-fire behavior of bond between high strength pozzolanic concrete and reinforcing steel [J]. Construction and Building Materials, 2004, 18(6): 425-435.
[7] EI-HAWARY M M, RAGAB A M, EI-AZIM A A, ELIBIARI S. Effect of fire on flexure behavior of RC beams [J]. Construction and Building Materials, 1996, 10(2): 147-150.
[8] EI-HAWARY M M, RAGAB A M, EI-AZIM A A, ELIBIARI S. Effect of fire on shear behavior of RC beams [J]. Computers and Structures, 1997, 65(2): 281-287.
[9] CHEN Y H, CHANG Y F, YAO G C, SHEU M S. Experimental research on post-fire behaviour of reinforced concrete columns [J]. Fire Safety Journal, 2009, 44(5): 741-748.
[10] WU Bo, MA Zhong-cheng, OU Jin-ping. Experimental study on seismic properties of RC columns after high temperature [J]. China Civil Engineering Journal, 1999, 32(2): 53-58. (in Chinese)
[11] XIAO Jian-zhuang, LI Jie, HUANG Zhan-fei. Fire response of high-performance concrete frames and their post-fire seismic performance [J]. ACI Structural Journal, 2008, 105(5): 531-540.
[12] LI Hong-nan, LI-Bing. Experimental study on seismic restoring performance of reinforced concrete shear walls [J]. Journal of Building Structures, 2004, 25(5): 35-42. (in Chinese)
[13] KUANG J S, HO Y B. Seismic behavior and ductility of squat reinforced concrete shear walls with nonseismic detailing [J]. ACI Structural Journal, 2008, 105(2): 225-231.
[14] ZHANG Yun-feng, WANG Zhi-hao. Seismic behavior of reinforced concrete shear walls subjected to high axial loading [J]. ACI Structural Journal, 2000, 97(5): 739-750.
[15] XIAO J Z, LI J, JIANG F. Research on the seismic behavior of HPC shear walls after fire [J]. Materials and Structures, 2004, 37(10): 506-512.
(Edited by YANG You-ping)
Foundation item: Project(200801410005) supported by Doctoral Foundation of Ministry of Education of China
Received date: 2009-11-21; Accepted date: 2010-03-24
Corresponding author: SONG Yu-pu, PhD, Professor; Tel: +86-411-86953174; E-mail: syupu@dlut.edu.cn
- Post-fire cyclic behavior of reinforced concrete shear walls
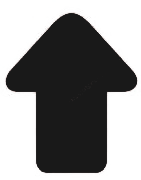