- Abstract:
- 1 Introduction▲
- 2 Experimental▲
- 3 Microstructure analysis▲
- 4 Conclusions▲
- References
- Figure
- Fig.1 Stock of aluminum/copper clad composite and copper tubes
- Fig.2 Schematic diagram of die structure and extrusion: 1—Punch die; 2—Cavity die; 3—External bush; 4—Internal bush; 5— Stock
- Fig.3 Successive processes of cold extrusion forming
- Fig.4 Microstructures of raw materials: (a) Copper; (b) Aluminum
- Fig.5 Microstructures of aluminium in cone-shaped deformation zone (a) and interface of original billet (b)
- Fig.6 Microstructures of deformed copper in cone-shaped deformation areas: (a) At interface; (b) On surface
- Fig.7 Microstructure of aluminum in axial section of cone-shaped deformation zone
- Fig.8 Microstructure of copper in axial section
- Fig.9 Streamline of copper in cone-shape zone
- Fig.10 Microstructures of extrusion products in axial section: (a) Aluminum; (b) Copper
- Fig.11 Microstructure of interface of extrusion product
- Fig.12 EDS energy spectrum analysis of interface: (a) SEM microstructure; (b) EDS spectrum
J. Cent. South Univ. Technol. (2011) 18: 1013-1017
DOI: 10.1007/s11771-011-0796-1
Microstructure of aluminum/copper clad composite fabricated by casting-cold extrusion forming
LUO Jun-ting(骆俊廷), ZHAO Shuang-jing(赵双敬), ZHANG Chun-xiang(张春祥)
State Key Laboratory of Metastable Materials Science and Technology, School of Mechanical Engineering,
Yanshan University, Qinhuangdao 066004, China
? Central South University Press and Springer-Verlag Berlin Heidelberg 2011
Abstract:
An aluminum/copper clad composite was fabricated by the casting-cold extrusion forming technology and the microstructures of the products were observed and analyzed. It is found that aluminum grains at the interface are refined in the radial profiles of cone-shaped deformation zone, but the grains in the center maintain the original state and the grain size is non-uniform. A clear boundary presents between the refined area and center area. In contrast, the copper grains in the radial profiles have been significantly refined. In the center area of the copper, the grains are bigger than those at the boundary. On the surface of the deformable body, the grain size is the smallest, but with irregular grain morphology. After the product is entirely extruded, all the copper and aluminum grains are refined with small and uniform morphology. In the center area, the average diameter of aluminum grains is smaller than 5 μm, and the copper grain on the surface is about 10 μm. At the interface, the grain size is very small, with a good combination of copper and aluminum. The thickness of interface is in the range of 10-15 μm. Energy spectrum analysis shows that CuAl3 phase presents at the interface.
Key words:
aluminum/copper clad composite; casting; cold extrusion; microstructure;
1 Introduction
The aluminum/copper clad composite is based on aluminum in core and a layer of copper coated on it to achieve the copper-aluminum solid combination at the interface [1]. The aluminum/copper clad composite is widely used in the inner conductor of the radio- frequency (RF) coaxial cable, with both the advantages of copper and aluminum [2].
Compared with the copper wire, the aluminum/ copper clad composite wire has the following advantages: smaller density, lighter mass, more convenience for transportation and installation, lower cost of production [3-4]. Due to these advantages of the aluminum/copper clad composite, its production process has attracted much attention in the recent years [5]. There are several ways to prepare the aluminum/copper clad composite: plating aluminum wire with copper, coated welding, roll extrusion, hydrostatic extrusion and continuous extrusion [6-7].
The casting-cold extrusion forming technology of aluminum/copper clad composite has been reported in Ref.[8]. The focus of the current work is to study the microstructure of the aluminum/copper clad composite since the microstructure not only decides the properties of the product but also affects the performance of the product [9-10]. Based on the forming of the composite product, a detailed study on microstructure of the aluminum/copper clad composite was carried out.
2 Experimental
2.1 Billet preparation and experimental conditions
Experiment was carried out at ambient temperature, and the basic parameters are listed in Table 1. The mixture of grease and graphite was used as lubricants. Billets for tests were aluminum/copper clad composite billets which were fabricated by the low-pressure casting technology [11]. The copper tube and low pressure casting billet samples are shown in Fig.1. Experiments were conducted on a hydraulic press YA315. Self-designed extrusion dies used during extrusion procedure are shown in Fig.2.
2.2 Experiment results
The successive processes of the cold extrusion formation of the casting billet are shown in Fig.3. Because aluminum is softer than copper and the flowing speed is quicker, the aluminum will outflow faster than than copper, and form a small pure aluminum rod zone in the head of the product. It is found that the product has uniform copper distribution, which shows the feasibility of the fabrication of the aluminum/copper clad composite.
Table 1 Experimental parameters for extrusion deformation
Fig.1 Stock of aluminum/copper clad composite and copper tubes
Fig.2 Schematic diagram of die structure and extrusion: 1—Punch die; 2—Cavity die; 3—External bush; 4—Internal bush; 5— Stock
Fig.3 Successive processes of cold extrusion forming
3 Microstructure analysis
3.1 Microstructure in radial cross-section
Products were carved along the radial and axial directions in the deformed conical region and the final extrusion region. The surface was ground with a metallographic sandpaper and then polished with polishing machine. Different etching solutions were used separately to copper and aluminum materials because they have different corrosion resistances. Hydrofluoric- nitric acid aqueous solution was used for aluminum and alcohol iron nitrate solution was used for copper. The microstructure was observed by metallographic microscopy and scamming electronic microscopy (SEM). The phase composition of interface was conformed by EDS energy spectrum analysis.
The microstructures of original billets are shown in Fig.4. The copper has irregular grains with diameters of about 40-50 μm in Fig.4(a); in contrast, aluminum consists of equiaxed grains, with diameters of 70-75 μm in Fig.4(b).
Fig.4 Microstructures of raw materials: (a) Copper; (b) Aluminum
The microstructure of aluminum in cone-shaped deformation zone is shown in Fig.5. Aluminum grains at the interface are refined when materials begin to come into the cone-shaped deformation zone, while the grains in the center remain the original state with large grain and non-uniform distribution. The boundary between the refined region and the large grain region is obvious. This configuration is mainly caused by different deformation degrees of materials between the outer layer and the center. Larger shear deformation takes place at the interface than in the center area, so the grains at the interface break more dramatically than those in the center. The thickness of the interface (about 10 μm) is more obviously attenuated than that of the casting billet (20-30 μm).
Fig.5 Microstructures of aluminium in cone-shaped deformation zone (a) and interface of original billet (b)
The microstructures of copper at the interface and on the surface of the deformation body in the cone-shaped deformation zone are shown in Figs.6(a) and (b), respectively. It can be seen that, the grains of copper is obviously refined with an average diameter of 20-25 μm. The grain size in the center of copper is larger, with an average diameter of about 35-40 μm. The grain size of copper on the surface is much smaller, with an average diameter of 10-15 μm, but the grain shape is very irregular, which shows that the shear deformation plays a major role during the grain refinement.
3.2 Microstructure in axial cross-section
The microstructure of aluminum in the axial section of the cone-shaped deformation zone near the interface is shown in Fig.7. A lot of strips or fibrous organization along the extrusion direction can be found, which are mainly caused by the shear strain at the interface during the extrusion process.
Fig.6 Microstructures of deformed copper in cone-shaped deformation areas: (a) At interface; (b) On surface
Fig.7 Microstructure of aluminum in axial section of cone-shaped deformation zone
The microstructure of copper in the axial section in the cone-shaped deformation zone is shown in Fig.8. In the axial section, the grains are refined for copper during the deformation. There are obvious streamlines in the axial section of the cone-shaped deformation zone in Fig.9, which proves that there exists orientation during the flow of copper along the cone angle direction. The existence of orientation and a large number of irregular grains indicate that the shear breakage plays a role in grain refinement.
3.3 Microstructure of extrusion products
The microstructure of extrusion products in the axial section is shown in Fig.10. From the microstructure of aluminum in Fig.10(a), it is noted that after complete extrusion, the aluminum grains are refined with small size and uniform distribution, with an average diameter of smaller than 5 μm. The microstructure of copper in Fig.10(b) shows that the copper grains are also very small and uniform after complete extrusion, but larger than those of aluminum, with an average diameter of about 10 μm.
Fig.8 Microstructure of copper in axial section
Fig.9 Streamline of copper in cone-shape zone
Fig.10 Microstructures of extrusion products in axial section: (a) Aluminum; (b) Copper
3.4 Microstructure at interface of final product
The microstructure at the interface of the extrusion product is shown in Fig.11. The grain size at the interface is very small and uniform, and the copper and aluminum have a good combination. The thickness of interface is about 10-15 μm, which is thicker than that in the cone-shaped deformation zone because of the combination of Cu and Al.
Fig.11 Microstructure of interface of extrusion product
The EDS energy spectrum analysis of interface is shown in Fig.12. The interface is an alloy phase of Cu and Al. According to the energy spectrum analysis of the alloy composition, the interface phase is most probably CuAl3.
Fig.12 EDS energy spectrum analysis of interface: (a) SEM microstructure; (b) EDS spectrum
4 Conclusions
1) Aluminum/copper clad composite can be fabricated by the casting-cold extrusion forming technology. The grain size of finial products can be refined completely during the cold extrusion. The shear breakage plays important role during the extrusion process for the grain refinement.
2) In the center area, the average diameter of aluminum grains is smaller than 5 μm, and the copper grain on the surface is about 10 μm. At the interface, the grain size is very small, with a good combination of copper and aluminum. The thickness of interface is in the range of 10-15 μm. Energy spectrum analysis shows that the CuAl3 phase presents at the interface.
References
[1] KANG C G, JUNG Y J, KWON H C. Finite element simulation of die design for extrusion process of Al/Cu composite and its experimental investigation [J]. Journal of Materials Processing Technology, 2002, 124(1/2): 49-56.
[2] CHEN Z C, IKEDA K. Fabrication of composite pipes by multi-billet extrusion technique [J]. Journal of Materials Processing Technology, 2003, 137(1/2/3): 10-16.
[3] LEE J S, SON H T, OH I H . Fabrication and characterization of Ti-Cu clad materials by indirect extrusion [J]. Journal of Materials Processing Technology, 2007, 187/188(15): 723-729.
[4] XU S, ZHAO G, REN G, MA X. Numerical simulation and experimental investigation of pure copper deformation behavior for equal channel angular pressing/extrusion process [J]. Computational Materials Science, 2008, 44(2): 247-252.
[5] RHEE K Y, HAN W Y, PARK H J, KIM S S. Fabrication of aluminum/copper clad composite using hot hydrostatic extrusion process and its material characteristics [J]. Materials Science and Engineering A, 2004, 384(1/2): 70-76.
[6] PARK H J, NA K H, CHO N S, LEE Y S. Hydrostatic extrusion of copper-clad aluminum rod [J]. Journal of Korean Society, Technical Plasticity, 1994, 4(2): 123-130.
[7] EIVANI A R, TAHERI K A. A new method for producing bimetallic rods [J]. Materials Letters, 2007, 61(4): 4110-4113.
[8] LUO Jun-ting, XU Yan, ZHAO Shuang-jing. Cold extrusion forming of copper/aluminum clad composite [J]. International Journal of Applied Mechanics and Materials, 2009, 16/17/18/19: 441-444.
[9] ZAO G Q, WANG G C, RAMANA V. Die cavity design of near flashless forging process using FEM-based backward simulation [J]. Journal of Materials Processing Technology, 2002, 121(2/3): 173-181.
[10] NARAYAASAMY R, RAMESH T, PANDEY K S. Some aspects on strain hardening behaviour in three dimensions of aluminium-iron powder metallurgy composite during cold upsetting [J]. Materials & Design, 2006, 27(8): 279-286.
[11] XU Yan, LUO Jun-ting, JIA Jian-bo. Study on the fabrication of aluminum/copper clad composite by low-pressure compression casting-cold extrusion technology [J]. China Mechanical Engineering, 2009, 20(22): 2755-2758. (in Chinese)
(Edited by PENG Chao-qun)
Foundation item: Project(60806006) supported by the National Natural Science Foundation of China
Received date: 2010-09-03; Accepted date: 2011-01-14
Corresponding author: LUO Jun-ting, Associate Professor, PhD; Tel: +86-335-8055529; E-mail: ljtlyk@yahoo.com.cn
- Microstructure of aluminum/copper clad composite fabricated by casting-cold extrusion forming
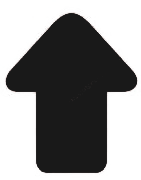