- Abstract:
- 1 Introduction▲
- 2 Experimental▲
- 3 Results and discus...▲
- 4 Conclusions▲
- References
- Figure
- Fig.1 XRD patterns of AC and Cu/AC
- Fig.3 SEM images of AC (a) and Cu/AC (b)
- Fig.4 Evolution of concentrations of phenol (a) and oxalic acid (b) in AC and Cu/AC systems (Ct is concentration at time t, C0 is initial concentration)
- Fig.5 Evolution of concentrations of phenol (a) and oxalic acid (b) in O3, O3/Cu/AC and O3/AC systems
- Fig.7 Evolution of pH value with phenol (a) and oxalic acid (b) in catalytic ozonation systems
J. Cent. South Univ. Technol. (2010) 17: 300-306
DOI: 10.1007/s11771-010-0046-y
Catalytic ozonation of phenol and oxalic acid with copper-loaded activated carbon
RAO Yi-fei(饶义飞)1, LUO Han-jin(罗汉金)1, WEI Chao-hai(韦朝海)1, LUO Ling-feng(罗凌峰)2
1. College of Environmental Science and Engineering, South China University of Technology,
Guangzhou 510006, China;
2. Knox College, The Second East South Street, Galesburg IL 61401, USA
? Central South University Press and Springer-Verlag Berlin Heidelberg 2010
Abstract:
A novel heterogeneous catalytic ozonation process in water treatment was studied, with a copper-loaded activated carbon (Cu/AC) that was prepared by an incipient wetness impregnation method at low temperature and tested as a catalyst in the ozonation of phenol and oxalic acid. Cu/AC was characterized using XRD, BET and SEM techniques. Compared with ozonation alone, the presence of Cu/AC in the ozonation processes significantly improves the degradation of phenol or oxalic acid. With the introduction of the hydroxyl radical scavenger, i.e., turt-butanol alcohol (t-BuOH), the degradation efficiency of both phenol and oxalic acid in the Cu/AC catalyzed ozonation process decreases by 22% at 30 min. This indicates that Cu/AC accelerates ozone decomposition into certain concentration of hydroxyl radicals. The amount of Cu(Ⅱ) produced during the reaction of Cu/AC-catalyzed ozonation of phenol or oxalic acid is very small, which shows that the two processes are both heterogeneous catalytic ozonation reactions.
Key words:
copper-loaded activated carbon; phenol; oxalic acid; hydroxyl radical; catalytic ozonation;
1 Introduction
Ozone is one of the most efficient oxidants for color and odour abatement and for water disinfection. However, ozonation alone for organics in the aqueous phase has some disadvantages [1], such as incomplete oxidation and low efficiency. In addition, saturated intermediates may accumulate in the final oxidation solution. Researches were focused on improving the utilization efficiency of ozone and overcoming the weakness of ozonation alone. Common adoption is the combination of ozone with other techniques. Many studies reported ozone-coupled advanced oxidation processes (AOPs) [2-7] such as O3/H2O2, O3/UV, and homogeneous catalytic ozonation, for instance, O3/Mn(Ⅱ), O3/Fe(Ⅱ), O3/Zn(Ⅱ) and O3/Co(Ⅱ), and heterogeneous catalytic ozonation, e.g., O3/Fe2O3/Al2O3, O3/TiO2/Al2O3, and O3/activated carbon. AOPs are aimed at producing more hydroxyl radicals (HO?) that have less selectivity and stronger oxidizability than molecular ozone. Among numerous AOPs, heterogeneous catalytic ozonation [8], which is the joint oxidation by ozone with a metal oxide or metal and metal oxide-supported catalyst, is one of the most promising processes and has earned lots of attention recently.
Activated carbon (AC) was recognized as an efficient adsorbent and support for catalysts in water treatment [7, 9-10]. Among metals, Cu is a common and effective precursor in catalytic applications [11-13]. AC was studied as a support for copper, bimetallic copper or copper oxide [14-21]. These catalysts are mainly applied in the treatment of gases [11, 14-17], such as the removal of SO2, NO and HCl from incineration flue gas, dehydrogenation ethylbenzene and methanol decomposition. There are just a few applications in water treatment [18-20]. Indeed, the reports about metal- loaded AC catalyzed ozonation in water treatment are very limited. LI et al [22] investigated the Ce-impregnated activated carbon catalyzed ozonation of dimethyl phthalate. FARIA et al [21, 23] studied the ceria-activated carbon composite for the catalytic ozonation of carboxylic acids, dyes, and textile effluents. LI et al [24] tested a nickel-loaded activated carbon (Ni/AC) as a catalyst in the ozonation of p-chlorobenzoic acid degradation in water.
To our knowledge, there has been no application of copper-loaded activated carbon (Cu/AC) prepared by an incipient wetness impregnation method at low temperature in the ozonation of organics in water. In this work, a novel heterogeneous catalytic ozonation process was studied with Cu/AC that was prepared by an incipient wetness impregnation method at low temperature. Cu/AC was characterized and tested in an oxidation system comprising ozone to achieve mineralization of organics, which was evaluated by using phenol and oxalic acid as model pollutants. The removal of both pollutants was compared with that of AC adsorption, ozonation alone, and catalyzed ozonation processes. Reaction mechanisms were investigated by the presence of the hydroxyl radical scavenger, turt-butanol alcohol (t-BuOH), and the measurement of pH and Cu(Ⅱ) concentrations in the reaction media.
2 Experimental
2.1 Materials
A granulated coal-based AC purchased from Xinhua Carbon Corp., Shanxi Province, China, was crushed to 0.20-0.45 mm. Chemicals were all analytical grades or guaranteed reagents. The ozone generator was model HF-3 (Yinhe Corp., China); the UV-Vis spectrophotometer was model UV 2450 (Shimazu Corp., Japan); pH meter was model pHS-3C (Leici Corp., China); ion chromatograph (IC) was model ICS-90 (Dionex Corp., USA); and the atomic absorption spectrophotometer (AAS) was model Z-2000 (Hitachi Corp., Japan).
2.2 Catalyst preparation
The preparation method for Cu/AC was slightly modified from that reported by CHOI et al [25]. AC was first washed with ultrapure water and dried at 110 ℃ overnight. Cu was incorporated into AC via an incipient wetness impregnation method, during which 13.6 g Cu(NO3)3·3H2O was dissolved at 55-60 ℃ with 5 mL ultrapure water and then mixed with 10 g AC for 10 min. For the total incorporation of Cu into AC, the slurry was dried at room temperature for 8 h, followed by drying at 60-70 ℃ using an infrared lamp for 6 h. The mixture was further calcined in a muffle furnace. The temperature was first ramped to 150 ℃ for 1 h, and then increased to 300 ℃ and held for 4 h. Finally, it was allowed to cool naturally.
2.3 Catalyst characterization
An XRD (Cu Kα radiation, λ=0.154 06 nm) technique was employed to observe the crystal structure of AC and Cu/AC at an ambient temperature using an X-ray diffractometer (X’Pert Pro, Panalytical Corp., Holand). The textural properties of AC and Cu/AC were determined from the corresponding N2 adsorption/ desorption isotherms obtained at 77 K with an automatic instrument (TriStarⅡ3020, Micromeritics Instrument Corp., USA). The samples were previously degassed in N2 atmosphere at 200 ℃ for 4 h. The surface areas, pore size distributions and micropore volumes of AC and Cu/AC were determined by the BET calculation method applied to the adsorption branch of their isotherms. The surface morphologies of AC and Cu/AC were obtained from SEM analysis using a scanning electronic micro- scope (model JCM-5700 JEOL, JEOL Crop., Japan).
2.4 Analytical methods
Ozone concentrations in the gas and aqueous solution were measured by the iodometric titration method [26] and the indigo method [27], respectively. The concentration of phenol in the aqueous solution was determined using UV-Vis spectrophotometery at the wavelength of λ=287.5 nm quantitatively [28]. IC was employed to detect the concentration of oxalic acid. Cu(Ⅱ) concentrations in Cu/AC and various reaction media during the catalytic ozonation were determined by AAS.
2.5 Ozonation experiments
Ozone was produced from pure oxygen in an ozone generator at a constant inlet ozone concentration of 11.2 mg/L. In each experiment, the initial concentration and volume of phenol and oxalic acid were 200 mg/L, 200 mL, and 10 mg/L and 1 000 mL, respectively. In consideration of catalytic effect and the initial concentration of pollutants, 500 mg of AC or Cu/AC was placed in the reactor. In the experiments in which ozone was involved, Na2S2SO3 solution was added to the sampling tube to quench any residual ozone in the reaction solution.
At a predetermined time, samples were first withdrawn and filtered (pore size 0.45 μm) and then the concentrations of phenol, oxalic acid, Cu(Ⅱ) and the pH value of the solution were measured. All the experiments were performed in an agitated glass reactor at an ambient temperature under the same operating conditions. All the glass containers were first soaked in HNO3 solution overnight, then placed in a sonic bath for 30 min, and finally rinsed with ultrapure water. The residual ozone in the off gas was adsorbed by KI solution. All solutions were prepared with ultrapure water obtained from a Millipore Milli-Q system.
3 Results and discussion
3.1 Catalyst characterization
Fig.1 shows the XRD patterns for AC and Cu/AC. Cu/AC has characteristic reflections at 2θ=35.5?, 38.7?, 48.8? and 61.4?, corresponding to CuO. This suggests that, after impregnation, Cu is loaded onto AC in the form of CuO. Furthermore, in both AC and Cu/AC there exists a strong peak at 2θ=26.5?, which is the typical peak of crystalline turbostratic carbon [29-30]. This indicates that the formation of CuO on Cu/AC does not alter the XRD patterns appreciably, but the signals of turbostratic carbon are weakened remarkably.
Fig.1 XRD patterns of AC and Cu/AC
Fig.2 N2 adsorption/desorption isotherms at 77 K (a) and BJH pore size distribution plots (b) of AC and Cu/AC (V is pore volume; D is pore diameter; BJH is one pore distribution calculation method that takes cylindrical pore as model)
N2 adsorption/desorption isotherms and pore size distributions of AC and Cu/AC are presented in Figs.2(a) and (b), respectively. With the formation of CuO in Cu/AC, the adsorption plateaus for AC are systematically above those of Cu/AC, which reveal that the adsorption capacity of Cu/AC is significantly lower than that of AC and the micropore volume of AC is always larger than that of Cu/AC. From Fig.2(b), there is a diminution of peak height and peak area around 4 nm, which reveals that the specific surface area, pore volume and pore size of Cu/AC decrease greatly.
The BET specific surface areas of AC and Cu/AC are 787 and 388 m2/g, separately. Compared with AC, the specific surface area of Cu/AC decreases by 51%, which is consistent with the N2 adsorption/desorption isotherms and pore size distribution results.
Fig.3 displays SEM images of AC and Cu/AC. Pores of various sizes and shapes can be observed from Fig.3. It can be seen from Fig.3(b) that Cu/AC is filled with some rod-like structures, which are compactly dispersed, probably because of the calcination or impregnation of CuO.
Fig.3 SEM images of AC (a) and Cu/AC (b)
The concentration of Cu(Ⅱ) in Cu/AC, as determined by AAS, was 2 μg/L. After calculation, it can be confirmed that Cu in Cu/AC almost exists in the form of CuO.
3.2 Degradation of phenol and oxalic acid
The results of evolution of phenol and oxalic acid in AC, Cu/AC, ozonation alone (O3), O3/AC and O3/Cu/AC systems are shown in Figs.4 and 5.
Fig.4 Evolution of concentrations of phenol (a) and oxalic acid (b) in AC and Cu/AC systems (Ct is concentration at time t, C0 is initial concentration)
Fig.5 Evolution of concentrations of phenol (a) and oxalic acid (b) in O3, O3/Cu/AC and O3/AC systems
3.2.1 Adsorption efficiency
The relationship between reaction time and the removal of phenol and oxalic acid by AC and Cu/AC adsorption is shown in Figs.4 (a) and (b), respectively. It can be seen that an increase in contact time results in an increase in the percentage of phenol and oxalic acid adsorbed by AC. At 30 min, the proportions of phenol or oxalic acid adsorbed by Cu/AC are only 5% and 4%, respectively. This can be explained by the limited BET specific surface area of Cu/AC, which only accounts for 49% of that of the AC. Moreover, with the formation of CuO, the surface structure of AC changes significantly, which can be confirmed from the characterization results of N2 adsorption/desorption and SEM. This observation is in agreement with the findings reported by FARIA et al [9].
3.2.2 Degradation under various ozonation processes
It can be observed from Fig.5 that the degradation rate of phenol by O3 at 30 min is 53%. However, the degradation of oxalic acid is very small. This is due to the refractory nature of oxalic acid. The degradation of phenol or oxalic acid by O3/AC is higher than that by O3/Cu/AC reactions. This can be explained by the different adsorption capacities of AC and Cu/AC. In the O3/Cu/AC process, Cu/AC increases the degraded proportion of phenol or oxalic acid to 30% and 52%, which indicates that the presence of Cu/AC can significantly improve the degradation of phenol or oxalic acid.
It is generally recognized that there are two ways by which ozone reacts with organics in water [1]. One is the direct oxidation by molecular ozone, the other is the radical chain reaction by the strong oxidant HO? that is decomposed by ozone. Ozone is mainly decomposed into HO? in aqueous systems, with E0=2.33 V, higher than that of ozone E0=2.07 V [1].
From the discussion above, AC and Cu/AC both improve the degradation of phenol and oxalic acid in catalytic ozonation processes. In order to verify whether the formation of HO? in aqueous ozone decomposition is promoted by AC or Cu/AC, a well known hydroxyl radical scavenger, t-BuOH, was used in the reactions. t-BuOH reacts very quickly with HO? (the rate constant is 5×108 mol/(L?s)), while very slowly with ozone (the rate constant is 0.03 mol/(L?s)) [10]. The results in the non-catalytic and catalytic ozonation in the presence of t-BuOH are shown in Fig.6.
Fig.6 Effects of t-BuOH dosage on phenol (a) and oxalic acid (b) removal in catalytic ozonation systems (Ct-BuOH (phenol)= 200 mg/L, Ct-BuOH (oxalic acid)=50 mg/L)
3.3 Impact of HO?
It can be observed from Fig.6 that the introduction of t-BuOH has little impact on the degradation of phenol or oxalic acid in the processes of O3 or O3/AC. This suggests that, the degradation of phenol or oxalic acid in ozonation alone is mainly performed by ozone molecules directly. Moreover, the addition of AC accelerates decomposition of ozone into a very small amount of HO?. This is probably due to the large specific surface area of AC that leads to a large adsorption capacity [9].
In the Cu/AC catalyzed ozonation of phenol or oxalic acid in the presence of t-BuOH, the degradation decreases gradually with the increase of reaction time. At 30 min, HO? accounts for 22% of the degradation of both pollutants. This denotes that Cu/AC accelerates O3 decomposition into HO? and there is a significant concentrations of HO? formed in these reactions. Moreover, the maximum concentrations of Cu(Ⅱ) in the middle and final oxidation of phenol and oxalic acid in O3/Cu/AC are less than 4 mg/L. After calculation, it can be obtained that Cu/AC has high stability during ozonation reactions. Besides, the reaction mechanism in the O3/Cu/AC processes of phenol or oxalic acid is heterogeneous catalytic ozonation.
3.4 Evolution of pH value
Different pH conditions lead to different mechanisms of ozonation of organics [1]. In order to determine the reaction mechanism, it is necessary to observe and interpret the effect of pH value. The evolution of pH value in various processes with phenol and oxalic acid is shown in Fig.7. It can be observed that in the reactions in which ozone is involved, the final pH value is much lower than the initial one. This is because phenol is degraded into carboxylic acids such as maleic, fumaric, oxalic and formic acid [5, 13] before complete mineralization, which leads to an increase in acidity. Because of the surface oxygen groups in AC [9], the final pH value is slightly higher in the AC adsorption and O3/AC than that in O3. Since the weak alkalinity of CuO in Cu/AC is coupled with the effect of the surface oxygen group in AC, the final pH value is only slightly increased in the Cu/AC adsorption and O3/Cu/AC process.
Fig.7 Evolution of pH value with phenol (a) and oxalic acid (b) in catalytic ozonation systems
Oxalic acid is one of the final products of phenol ozonation [13, 25] because of its refractory nature. Therefore, it is difficult to be oxidized by ozone molecules. From Fig.7(b), in the ozonation of oxalic acid alone, no major pH change is observed. With the reaction processing, pH value becomes stable. In the processes of AC adsorption and O3/AC, pH value slightly increases. While in the processes of Cu/AC and O3/Cu/AC, pH value increases gradually and finally becomes stable at around 7.3. This can be explained by the refractory nature of oxalic acid, the weak alkalinity of CuO and the surface oxygen group of activated carbon.
4 Conclusions
(1) A novel catalyst Cu/AC is prepared by an incipient wetness impregnation method at low temperature, which is tested in the catalytic ozonation processes.
(2) Cu is loaded on the Cu/AC in the form of CuO, which is evidenced by XRD and AAS analysis. The BET specific surface area and pore size distribution of Cu/AC are changed significantly compared with those of the original AC. These are confirmed by the findings of N2 adsorption/desorption, pore size distributions, and SEM images of AC and Cu/AC.
(3) The presence of Cu/AC in ozonation processes significantly improves the degradation of phenol or oxalic acid. With the introduction of t-BuOH, the degradations of phenol or oxalic acid in O3/Cu/AC processes both decrease by 22% at 30 min. Cu/AC accelerates ozone decomposition into a certain amount of HO?. The results of Cu(Ⅱ) concentration suggest that Cu/AC-catalyzed ozonation of phenol and oxalic acid is heterogeneous catalytic ozonation.
(4) The pH evolution in the catalytic ozonation of phenol or oxalic acid indicates that CuO and the surface oxygen groups on activated carbon have a slight effect on the degradation of phenol and oxalic.
References
[1] KASPRZYK-HORDERN B, ZI??EK M, NAWROCKI J. Catalytic ozonation and methods of enhancing molecular ozone reactions in water treatment [J]. Applied Catalysis B: Environmental, 2003, 46(4): 639-669.
[2] WU Yao-guo, ZHAO Chen-hui, WANG Qiu-hua, DING Ke. Integrated effects of selected ions on 2, 4, 6-trinitrotoluene-removal by O3/H2O2 [J]. Journal of Hazardous Materials, 2006, 132(2/3): 232-236.
[3] SHANG N C, CHEN Y H, MA H W, LEE C W, CHANG C H, YU Y H, LEE C H. Oxidation of methyl methacrylate from semiconductor wastewater by O3 and O3/UV processes [J]. Journal of Hazardous Materials, 2007, 147(1/2): 307-312.
[4] WU C H, KUO C Y, CHANG C L. Homogeneous catalytic ozonation of C.I. Reactive Red 2 by metallic ions in a bubble column reactor [J]. Journal of Hazardous Materials, 2008, 154(1/3): 748-755.
[5] BELTR?N F, RIVAS F, MONTERO-DE-ESPINOSA R. Iron type catalysts for the ozonation of oxalic acid in water [J]. Water Research, 2005, 39(15): 3553-3564.
[6] BELTR?N F, RIVAS F, MONTERO-DE-ESPINOSA R. A TiO2/Al2O3 catalyst to improve the ozonation of oxalic acid in water [J]. Applied Catalysis B: Environmental, 2004, 47(2): 101-109.
[7] KHAN M, JUNG J. Ozonation catalyzed by homogeneous and heterogeneous catalysts for degradation of DEHP in aqueous phase [J]. Chemosphere, 2008, 72(4): 690-696.
[8] LEGUBE B, KARPEL VEL LEITNER N. Catalytic ozonation: A promising advanced oxidation technology for water treatment [J]. Catalysis Today, 1999, 53(1): 61-72.
[9] FARIA P, ?RF?O J, PEREIRA M. Catalytic ozonation of sulfonated aromatic compounds in the presence of activated carbon [J]. Applied Catalysis B: Environmental, 2008, 83(1/2): 150-159.
[10] VALD?S H, ZAROR C A. Heterogeneous and homogeneous catalytic ozonation of benzothiazole promoted by activated carbon: Kinetic approach [J]. Chemosphere, 2006, 65(7): 1131-1136.
[11] OLIVEIRA S, BARBOSA D, MONTEIRO A, RABELO D, RANGEL M. Evaluation of copper supported on polymeric spherical activated carbon in the ethylbenzene dehydrogenation [J]. Catalysis Today, 2008, 133/135: 92-98.
[12] BO L L, ZHANG Y B, QUAN X, ZHAO B. Microwave assisted catalytic oxidation of p-nitrophenol in aqueous solution using carbon-supported copper catalyst [J]. Journal of Hazardous Materials, 2008, 153(3): 1201-1206.
[13] PIMENTEL M, OTURAN N, DEZOTTI M, OTURAN M. Phenol degradation by advanced electrochemical oxidation process electro-Fenton using a carbon felt cathode [J]. Applied Catalysis B: Environmental, 2008, 83(1/2): 140-149.
[14] TSENG H H, WEY M Y, LIANG Y S, CHEN K H. Catalytic removal of SO2, NO and HCl from incineration flue gas over activated carbon-supported metal oxides [J]. Carbon, 2003, 41(5): 1079-1085.
[15] TSONCHEVA T, VANKOVA S, BOZHKOV O, MEHANDJIEV D. Effect of rhenium on copper supported on activated carbon catalysts for methanol decomposition [J]. Journal of Molecular Catalysis A: Chemical, 2005, 225(2): 245-251.
[16] ZHANG Zhen, MA Xin-bin, ZHANG Ping-bo, LI Ye-ming, WANG Sheng-ping. Effect of treatment temperature on the crystal structure of activated carbon supported CuCl2-PdCl2 catalysts in the oxidative carbonylation of ethanol to diethyl carbonate [J]. Journal of Molecular Catalysis A: Chemical, 2007, 266(1/2): 202-206.
[17] ZHU Zhen-ping, LIU Zhen-yu, LIU Shou-jun, NIU Hong-xian, HU Tian-don, LIU Tao, XIE Ya-ning. NO reduction with NH3 over an activated carbon-supported copper oxide catalyst at low temperatures [J]. Applied Catalysis B: Environmental, 2000, 26(1): 25-35.
[18] MATATOV-MEYTAL U, SHEINTUCH M. Activated carbon cloth-supported Pd-Cu catalyst: Application for continuous water denitrification [J]. Catalysis Today, 2005, 102/103: 121-127.
[19] CALVO L, GILARRANZ A, CASAS A, MOHEDANO F, RODR?GUEZ J. Hydrodechlorination of alachlor in water using Pd, Ni and Cu catalysts supported on activated carbon [J]. Applied Catalysis B: Environmental, 2008, 78(3/4): 259-266.
[20] ZHAO Jiang-hong, LIU Zhen-yu, SUN De-kui. TPO-TPD study of an activated carbon-supported copper catalyst-sorbent used for catalytic dry oxidation of phenol [J]. Journal of Catalysis, 2004, 227(2): 297-303.
[21] FARIA P, ?RF?O J, PEREIRA M. A novel ceria-activated carbon composite for the catalytic ozonation of carboxylic acids [J]. Catalysis Communications, 2008, 9(11/12): 2121-2126.
[22] LI Lai-sheng, YE Wei-ying, ZHANG Qiu-yun, SUN Feng-qiang, LU Ping, LI Xu-kai. Catalytic ozonation of dimethyl phthalate over cerium supported on activated carbon [J]. Journal of Hazardous Materials, 2009, 170(1): 411-416.
[23] FARIA P, ?RF?O J, PEREIRA M. Activated carbon and ceria catalysts applied to the catalytic ozonation of dyes and textile effluents [J]. Applied Catalysis B: Environmental, 2009, 88(3/4): 341-350.
[24] LI Xu-kai, ZHANG Qiu-yun, TANG Li-li, LU Ping, SUN Feng-qiang, LI Lai-sheng. Catalytic ozonation of p-chlorobenzoic acid by activated carbon and nickel supported activated carbon prepared from petroleum coke [J]. Journal of Hazardous Materials, 2009, 163(1): 115-120.
[25] CHOI H, AL-ABED S, AGARWAL S, DIONYSIOU D. Synthesis of reactive nano-Fe/Pd bimetallic system-impregnated activated carbon for the simultaneous adsorption and dechlorination [J]. Chemistry of Materials, 2008, 20(11): 3649-3655.
[26] RAKNESS L, GORDON G, LANGLAIS B. Guideline for measurement of ozone concentration in the process gas from an ozone generator [J]. Ozone Science and Engineering, 1996, 18(3): 209-229.
[27] BADER H, HOIGNE J. Determination of ozone in water by the indigo method [J]. Water Research, 1981, 15(4): 449-456.
[28] ZHANG Qing-hong, GAO Lian, GUO Jing-kun. Effects of calcination on the photocatalytic properties of nanosized TiO2 powders prepared by TiCl4 hydrolysis [J]. Applied Catalysis B: Environmental, 2000, 26(3): 207-215.
[29] XU C B, HAMILTON S, GHOSH M. Hydro-treatment of Athabasca vacuum tower bottoms in supercritical toluene with microporous activated carbons and metal-carbon composite [J]. Fuel, 2009, 88(11): 2097-2105.
[30] RINALDI A, ABDULLAH N, ALI M, FURCHE A, HAMID S, SU D S, SCHL?GL R. Controlling the yield and structure of carbon nanofibers grown on a nickel/activated carbon catalyst [J]. Carbon, 2009, 47(13): 3023-3033.
Foundation item: Project(40973074) supported by the National Natural Science Foundation of China
Received date: 2009-07-04; Accepted date: 2009-09-29
Corresponding author: LUO Han-jin, PhD, Associate professor; Tel: +86-20-88375960; E-mail: luohj@scut.edu.cn
(Edited by CHEN Wei-ping)
Abstract: A novel heterogeneous catalytic ozonation process in water treatment was studied, with a copper-loaded activated carbon (Cu/AC) that was prepared by an incipient wetness impregnation method at low temperature and tested as a catalyst in the ozonation of phenol and oxalic acid. Cu/AC was characterized using XRD, BET and SEM techniques. Compared with ozonation alone, the presence of Cu/AC in the ozonation processes significantly improves the degradation of phenol or oxalic acid. With the introduction of the hydroxyl radical scavenger, i.e., turt-butanol alcohol (t-BuOH), the degradation efficiency of both phenol and oxalic acid in the Cu/AC catalyzed ozonation process decreases by 22% at 30 min. This indicates that Cu/AC accelerates ozone decomposition into certain concentration of hydroxyl radicals. The amount of Cu(Ⅱ) produced during the reaction of Cu/AC-catalyzed ozonation of phenol or oxalic acid is very small, which shows that the two processes are both heterogeneous catalytic ozonation reactions.
- Catalytic ozonation of phenol and oxalic acid withcopper-loaded activated carbon
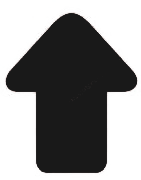