- Abstract:
- 1 Introduction▲
- 2 Regional conditions▲
- 3 Warming and degeneratin...▲
- 4 Increase of thaw hazard...▲
- 5 Vegetation damage and l...▲
- 6 Thermal influences from...▲
- 7 Permafrost environment ...▲
- 8 Suggestions for protect...▲
- 9 Conclusions▲
- References
- Figure
- Fig.1 Location of studied segment of Qinghai–Tibet Highway on Qinghai–Tibet Plateau and frozen ground types
- Fig.2 Thaw hazards developed along QTH: (a) Thaw slumping at Beiluhe Basin; (b) Some small water pits developed at Chumarhe High Plateau
- Fig.3 Comparison of geological profiles in undisturbed (a) and failed (b) area of thaw slumping at K3035 of QTH
- Fig.4 Comparison of geological profiles in undisturbed adjacent terrain (a) and beneath thermokarst pond bottom (b) at K3057 of QTH
- Fig.5 Mean annual values of ground temperature at different depths: (a) In undisturbed area and two failed areas of thaw slumping at K3035 and K3057 of QTH; (b) In adjacent terrain and thermalkarat ponds bottom at K3057
- Fig.6 Failure scale development: (a) Retrogressive scale–time curves of thaw slumping at K3035; (b) Lakeshore retrogression–time curves of thermalkarst pond at K3057
- Fig.7 Service roads at Budongquan and Beiluhe regions: (a) Zigzag service road with about 3 km long passing through vegetative mat from Westside of QTH at Budongquan (K2923 of QTH); (b) Jammed heavy trucks, and cars running on grassland
J. Cent. South Univ. Technol. (2011) 18: 1454-1464
DOI: 10.1007/s11771-011-0861-9
Changes in permafrost environments caused by construction and maintenance of Qinghai-Tibet Highway
LIN Zhan-ju(林战举), NIU Fu-jun(牛富俊), LUO Jing(罗京), LU Jia-hao(鲁嘉濠), LIU Hua(刘华)
State Key Laboratory of Frozen Soil Engineering,
Cold and Arid Regions Environmental and Engineering Research Institute,
Chinese Academy of Sciences, Lanzhou 730000, China
? Central South University Press and Springer-Verlag Berlin Heidelberg 2011
Abstract:
The sideward permafrost along the Qinghai-Tibet Highway (QTH) contains massive ground-ice and is at a relatively high temperature. Under the influence of the steady increase of human activities, the permafrost environment has been changed greatly for a long time. At present, the permafrost becomes warm and rapidly degenerates, including the decline of the permafrost table, rising of the ground temperature, shortening of the length of frozen section, and extension of range of melting region. Some thaw hazards (e.g. thaw slumping and thermokarst pond) have widely occurred along both sides of the roadbed. In addition, due to the incomplete construction management, the vegetation adjacent to the highway is seriously damaged or eradicated, resulting in the land desertification and ecosystem out of balance. The dust, waste and garbage brought by drivers, passengers, maintenance workers, and transportations may also pollute the permafrost environment.
Key words:
1 Introduction
The Qinghai-Tibet Highway (QTH), from Golmud, Qinghai Province in the north, to Lhasa, Tibet Autonomous Regions in the south, is one of the major linear projects in the Qinghai-Tibet Engineering Corridor (QTEC). It (the section from Golmud to Lhasa) was built in 1950s and was a pioneering engineering project in the permafrost regions on the Qinghai-Tibet Plateau (QTP). The asphaltic paving was begun in 1973, and the entire length was paved with an asphalt surface in 1985. In the 1990s and in the early 21st century, it was rehabilitated for three times, including some re-routing works. The recent improvement, which was regarded as the mostly large-scale rehabilitation, was begun in the early 2008, and finished in the end of 2010. During the past nearly 60 years, although it has played an important role to promote the economic development of Qinghai Province and Tibet Autonomous Region, its construction, rehabilitation or improvement, maintenance and operation induced inevitably the changes in permafrost and its environmental problems because roadbed located on the permafrost region with the high ground temperature and high ice-content. On one hand, the existence of the linear engineering has changed the thermal exchange between the ground surface and the atmosphere, then influenced the underlying permafrost environment, resulting in the increase of the ground temperature and acceleration of the process of permafrost degradation [1-3]; on the other hand, in many areas, the surface vegetation and the top soils have been completely removed, or destroyed, and have led to significantly increased water and soil erosion, with extensive and serious thaw hazards along both sides of the QTH. Therefore, the changes in the permafrost environment caused by the construction and maintenance of the QTH are discussed, and other permafrost problems caused by the operation and repairs are also studied. In addition, it is recommended to limit or mitigate damages to the permafrost environments along the QTH. As the Qinghai-Tibet Expressway is under consideration, the influences of the engineering activities on the permafrost environment need to be paid more attention.
2 Regional conditions
The total length of the QTH is 1 160 km, of which 531 km crosses continuous permafrost areas (Fig.1). Approximately 80% of the length exceeds 4 000 m in elevation while a portion of it, about 50 km, exceeds 5 000 m. The mean annual air temperature (MAAT) is below -4 °C, the extremely lowest temperature is up to -30 °C in winter, and the extremely highest temperature is approximately 25 °C in summer. The annual precipitation, concentrated in the period from May to August, is about 50-400 mm. The dry and loose soils which are dominated by alpine steppe soil and alpine meadow soil are disadvantageous to vegetation growth and are difficult to form the protective layer with the multi-root and high-organic matter upon their surfaces. Consequently, they are weak in buffer capacity and resistance to mechanical damage from runoff [4].
Fig.1 Location of studied segment of Qinghai–Tibet Highway on Qinghai–Tibet Plateau and frozen ground types
There is no tree along the QTH, and even the shrub is very difficult to survive. Influenced by the cold climate condition, the vegetation is featured by the simple types, monotone community physiognomy, low-stature (10-15 cm), short growth period, and low-biomass, etc. The sparse alpine grasslands and meadows, such as Stipa purpures and Kpbresia pygmaea, spread on the numerous bare sandy areas. At section where vegetation and soils were severely damaged, it is very difficult to recover to the original conditions. The high elevation, periglacial processes, and cold and arid continental climates have influenced, or in a certain extent, determined the configuration and distribution of the sensitive permafrost environments in this corridor. The permafrost on the QTP is featured by massive ground-ice and high ground temperature. About 275 km, or more than 50%, is over “warm” (-1 to 0 °C) permafrost and 40% is over ice-rich permafrost [5-6]. The fragile permafrost environments have been easily changed, disturbed, or damaged by the construction and other anthropogenic activities.
3 Warming and degenerating of permafrost along QTH
The construction of the QTH changed the thermal exchange between atmosphere and ground surface, resulting in the temperature rising of the permafrost below the roadbed. The observational results of the past 30 years indicate that taliks (perennial thaw layer between the bottom of the seasonally frozen layers and the top of the permafrost table) have formed beneath the roadbed and the length accounted for about 56% of the whole QTH permafrost sections [7]. These sections generally located in the Xidatan Basin, Chumarhe High Plateau, Beiluhe Basin etc, with the mean annual ground temperature (MAGT) greater than -1.5 °C (warm permafrost) and the relatively lower elevations (Table 1). The formation of the taliks signifies that the permafrost underlain roadbed becomes warm and degenerated, and also makes it to form a “thawed channel” below the roadbed [10-11]. Most of the taliks contain free water throughout the year and may cause much more thaw settlements, resulting in the damages of the roadways, especially when the heavy trucks pass in warm seasons. According to the survey to QTH in 1990, the failed sections accounted for about 32% of the total length of the QTH in the permafrost areas [12], and about 80%- 85% roadbed failures were resulted from the permafrost thawing settlements [13].
With the changes of the permafrost environment and the overload running of the automotive vehicles, the thickness of taliks beneath the roadbed has increased by about 30-50 cm during the past decades [3, 11-12, 14]. The development of talik would lead to the increase of seasonally frozen depth, the decline of permafrost table, and the rising of the ground temperatures (Table 2). Some stable or quasi-stable permafrost areas have been extending into the unstable or the extremely unstable permafrost areas. The numerical simulation results show that the sections with taliks will probably extend to all the QTH sections in the permafrost areas if the permafrost degradation will be accelerated ceaselessly during the next 50-100 years [15]. At that time, some low- temperature permafrost areas, such as Kunlun Mountain, Fenghuoshan Mountain and Tanggula Mountain, will develop into severe damage sections. The permafrost degradation along, later to and below QTH is obviously quicker than that of other natural regions. Under the natural state, the permafrost section has shrunk by 0.5- 1 km at the north edge and 1-2 km at the south edge, whereas under the influence of the highway construction and operation, it has shrunk by 5-8 km at the north edge and 9-12 km at the south edge [12, 16-17]. The total length of permafrost sections along the QTH was shortened from 531 to 522 km, with shrinkage at both ends, during the period of 1991-2003 [18-19]. Therefore, the influence from the engineering activities on the permafrost degradation is more serious than that of the natural force.
Table 1 Comparison of mean annual air temperature (MAATs), mean annual ground temperature (MAGTs) and depths of permafrost table positions under natural and asphaltic road surfaces along QTEC (revised from Refs.[8-9])
Table 2 Comparison of permafrost table and ground temperature in 2 m deep at natural ground and toe of slope of QTH
In addition, the QTH covered by the asphalt layer absorbs about 20% more heat than the other surfaces [7]. The asphalt surface also influences the heat exchange between the permafrost and atmosphere because it prevents the evaporation on the surface. The observed results show that the time of thawing of the soil under the asphalt surface is earlier 20-30 d than that under the natural state, and the time of freezing is later about 20 d. On the other hand, the ground temperature under the asphalt road surfaces is 1-2 °C higher than that under the natural condition, and the depth of permafrost table has also an increase of 1.5- 3 m (Table 2).
4 Increase of thaw hazards caused by cutting or surface disturbance
The permafrost was initially met during the construction of the QTH, and the means of permafrost protection were not included in the design process of the QTH in 1950s. In fact, no clear guidelines instructed the engineering construction and also no valid technology measures were used to restrain the possible permafrost variation at that time. The embankment filling came directly from both sides in a range of 50-100 m. These cuttings for construction material seriously damaged the vegetation and turf mulching and disturbed the permafrost beneath it, resulting in the thawing of ice-rich permafrost or melting of massive ground ice. Just because of this, some environment hazards were rooted thereby. They mainly included thaw slumping (Fig.2(a)) in sloping regions, and thermokarst ponds or small water pits (Fig.2(b)) etc. in plain or basin regions. The sections with permafrost hazards accounted for about 40% of the total length of the QTH in the permafrost sections [8].
Fig.2 Thaw hazards developed along QTH: (a) Thaw slumping at Beiluhe Basin; (b) Some small water pits developed at Chumarhe High Plateau
Thaw slumping and thermokarst pond (or water accumulating pit) become two kinds of the representative thaw hazards and widely exist along the QTH and have serious influence on the change in permafrost environment. The thaw slumping is commonly caused by slope toe disturbance in the ice-rich permafrost regions and slumping process would develop year by year once it is formed. The thaw slumping regions caused by the construction of the QTH mainly locate in the Hoh Xil Hill Region (K3014 to 3019), Beiluhe Basin (K3030 to 3060), and southern slope of Fenghuoshan Mountain (K3070 to 3080) (Fig.1), and the total length is about 45 km.
Thermokarst pond is characterized by the landforms that are developed as a result of thawing of the ice-rich permafrost or melting of the massive ground ice [20-21]. Normally, the thawing or melting is resulted from the changes or disturbance of the ground surface due to the digging or rolling of construction vehicles. This not only changes the original ground surface, but also leaves many pits along the way which are subsequently filled with water. Such small water pits are developed into thermokarst ponds after undergoing long-term collapse. Thermokarst ponds and water pits widely locate in the Chumarhe High Plateau, Wudaoliang Basin (a special part of Hoh Xil Hill Region), and Beiluhe Basin, etc. (Fig.1). A detailed statistical data in 2007 show that approximately 69 thermokarst ponds and 234 water pits have been developed at both sides of roadway of QTH from Xidatan Basin to Hoh Xil Hill region, and their areas are about 1.5×104 m2 and 1.0×104 m2, respectively [9]. The current survey in September 2009 shows the surface water areas (including thermokarst ponds and water pits) from Xidatan Basin to Beiluhe Basin have already been up to 3.5×105 m2 because of the disturbance from the current improvement project which started in 2008.
In order to further discuss the thaw hazards caused by the cutting or disturbance influence on the permafrost, two thaw slumping cases (located at K3035 (Fig.2(a)) and K3057 of QTH mileage, respectively) and a thermokarst pond case (located at K3057 (Fig.2(b)) are studied along with corresponding undisturbed areas based on field observation and monitoring (Fig.1).
4.1 Variations in permafrost configuration below failed area
Due to the surface vegetation damage, the disturbed surface absorbs more energy than before, resulting in variations of permafrost configuration. These mainly show as a difference in permafrost table and ice contents. Figure 3 reflects the differences of the geologic profiles of cores between the undisturbed area (approximately 100 m from the slumping area) and the failed area of thaw slumping at K3035 of the QTH. The depth of permafrost table is about 2 m in the undisturbed area, with 15%-20% of it covered by vegetation (Fig.3(a)), whereas it is 2.3 m in the failed area where the surface is exposed (Fig.3(b)). In addition, the thickness of ground- ice in the undisturbed area is 4.0 m, while it is only 1.7 m in the failed area. It is obvious that about 2.3 m-thick ground-ice has been melted with the ongoing of slumping process. Of course, evaporation and sublimation maybe also coexist; but in any case, the decrease of the ground-ice is faitaccompli.
Figure 3(b) also indicates that there is no vegetation and clay in the failed area and a 1 m-thick clay layer under superficial sand and vegetation is replaced by 2 m- thick sand. This is because the failures begin with melting of the ground ice exposed by the cut and are followed with the collapse of the cut wall and soil mass slide. The failure shows as collapse and slide of active layer along the massive ground-ice surface. The vegetations near headwall, clay and upper sand are taken to the failed area or buried with the collapse and slide process. Meanwhile, with the melting of ground ice and seeping of groundwater, fine particles of soil may be taken away and the mixture of coarse soil particles and sand is left on the lower edge of the headwall.
Figure 4 also reflects the differences of the geologic profiles of cores in the undisturbed area (about 100 m from the edge of the lake) and beneath thermokarst pond bottom at K3057 of the QTH. The depth of permafrost table is 1.8 m in the undisturbed area (Fig.4(a)); whereas it is 9.6 m beneath the pond bottom (Fig.4(b)). The difference between the former and the later is up to about 8.0 m. In addition, the thickness of ground-ice in the natural area is 2.9 m; whereas it is completely melted beneath the pond bottom. The above comparison fully illustrates that the presence of the water body has a great influence on the permafrost environment.
Fig.3 Comparison of geological profiles in undisturbed (a) and failed (b) area of thaw slumping at K3035 of QTH
Fig.4 Comparison of geological profiles in undisturbed adjacent terrain (a) and beneath thermokarst pond bottom (b) at K3057 of QTH
4.2 Difference in ground temperatures between in failed and in undisturbed areas
Ground temperature is one of the important indexes that reflect the change of permafrost. The mean annual value of ground temperature at different depths both in the undisturbed and in the failed areas of the thaw slumping at K3035 and K3057 during a year (from August 11, 2002 to August 11, 2003) are shown in Fig.5(a). It is indicated that the temperatures in the undisturbed area are lower than those in the failed areas. The maximum difference of ground temperatures appears at a depth of 2 m, where the ground temperature of natural ground is -3.38 °C, while it is -1.88 °C in the failed area of K3035, and -2.28 °C in the failed area of K3057. On the other hand, by comparisons of the shape of the three curves in Fig.5(a), the one in the failed area at K3035 concaves toward negative temperature within 0-4 m. This feature would cause the permafrost in the area to process degradation.
Figure 5(b) reflects the mean annual value of ground temperatures at different depths both in the undisturbed ground and beneath the thermokarst ponds bottom at two positions (1.0 and 2.5 m in water depth) at K3057 during the past two years (from January 1, 2006 to December 31, 2007). The curves also indicate that the temperatures in undisturbed ground are lower than those beneath ponds bottom. As far as the MAGT is concerned, it is -1.06 °C in undisturbed ground, 4.5 °C beneath the pond with water depth of 2.5 m, and -0.17 °C beneath the pond with water depth of 1.0 m. The differences in deeper water ponds are more obvious than those in shallower water ponds.
Fig.5 Mean annual values of ground temperature at different depths: (a) In undisturbed area and two failed areas of thaw slumping at K3035 and K3057 of QTH; (b) In adjacent terrain and thermalkarat ponds bottom at K3057
4.3 Failure scale development
Both the thaw slumping and the thermokarst pond have a common characteristic, that is, the development of the failure scale. Figure 6(a) shows the extending trend of the headwall of the thaw slumping at K3035 (eight group monitoring points are laid along the seriously slumping area fringe). This indicates that the headwall has retrogressed 5 m in 2002 and nearly 3 m in 2003. The failure in fact occurs mainly from July to October. According to the data from recent three years, the current collapse- extending velocity is less than 5 m/a. Although the velocity slows down, there spread many fissures behind the headwall and there is no evidence that the failure would stop soon.
The area expansion of the thermokarst pond at the K3057 is shown in the Fig.6(b) (nine group monitoring points are laid in the lakeshore). The measured results show that almost all lakeshores have different extent retrogressions from August 2007 to October 2009. The collapsing ranges are all more than 0.5 m, and the maximum value is about 2.8 m. There are many cracks and collapsing blocks within 10 m of the lakeshore edge. These cracks are gradually widened, and developed more rapidly in the warm season. The blocks cut by the cracks tilt toward the lake, and collapse into the water when the ice-rich soil thaw or massive ice melts. Waves help to break up the blocks in the water, and the remnants constitute the lake-bottom sediments.
The permafrost environments in the thaw slumping areas and beneath or around the thermokarst ponds have been disturbed or damaged. The depth of the permafrost table is steadily increased due to the rising of ground temperatures. In the worst case scenario, such as beneath the thermokarst ponds with over 2.5 m water depth, the permafrost has completely thawed [22-23]. Therefore, the influence of thaw hazards on the local permafrost environment is obvious and direct. It is resulted from the fact that massive ground-ice or ice-rich permafrost melts or thaws, the permafrost table declines, the ground temperatures rise and permafrost stability falls, etc.
Fig.6 Failure scale development: (a) Retrogressive scale–time curves of thaw slumping at K3035; (b) Lakeshore retrogression–time curves of thermalkarst pond at K3057
5 Vegetation damage and land desertification
During the first stage of the highway construction in 1950s, due to the insufficient understanding to protection permafrost environment, excavations damaged the natural surface and the vegetative mat along the both sides of QTH. After the asphaltic paving in 1970s, although the protection of permafrost environment has been paid more attention, the construction of embankment still adopted the measures of the borrow soil from both sides adjacent to highway; the fragile permafrost and ecological environment were damaged again following the cut zones (Figs.2(a) and (b)). According to the observation in past several years, there was still no vegetation in these zones. In July 2007, the area and length of the sections of the previous excavation-trace were investigated. The results show that the length where vegetative mat was damaged accounted for about 17% of total permafrost sections and the area reached about 1.6×105 m2 from Xidatan to Fenghuoshan Pass (Table 3).
During the period of the later rehabilitations and current improvement of the QTH, protection of permafrost environment was paid more attention and a series of the engineering measures (e.g. thermosyphons and special structure embankments) were applied to the construction [24]. However, due to the insufficient construction management during the improvement and repairing works started in 2008, the permafrost environment was damaged by construction of the service road. Figure 7 shows that the temporarily service roads were opened on the natural surface (Fig.7(a)). In September 2009, a statistic data showed that the damaged or eradicated vegetation area was up to about 2.5×105 m2, and the length accounted for about 56% of total permafrost section from Xidatan to Fenghuoshan Pass (Table 3). Passing of heavy trucks left many tracks and pits, resulting in grassland damages (Fig.7(b)).
Table 3 Statistics of damaged vegetation area from Xidatan (K2860 of QTH) to Fenghuoshan Pass (K3070 of QTH)
Fig.7 Service roads at Budongquan and Beiluhe regions: (a) Zigzag service road with about 3 km long passing through vegetative mat from Westside of QTH at Budongquan (K2923 of QTH); (b) Jammed heavy trucks, and cars running on grassland
The vegetation along the QTH is dominated by alpine grasslands and meadows. It has a slow growth rate and little height because of the high elevations, rigorous climates, persistent winds and inefficient availability of moisture and nutrients. These natural conditions determine that it is very difficult to recovery once vegetation is damaged. A study shows it will take 20- 30 a for alpine grasslands to recover their ecological structures and biodiversity similar to those of the original conditions, whereas it will take 45-60 a for the alpine meadows [9]. The long-termed exposed surface causes inevitable decline of the permafrost table and increase of the ground temperature.
The damage of the natural surface also accelerates the process of the land desertification, especially in regions with sparse vegetation and strong wind. The desertification not only directly threatens the operation of the highway and railway, and severely influences on the regional ecological environment, but also accelerates the permafrost degradation. The natural vegetation has a good thermal insulation performance, and the existence of the turf mulching is beneficial to the formation of the humus soil. They all have good promotion effects on the development and formation of the permafrost. However, if the vegetation is eradicated and the plants root dies, the soil types may be changed correspondingly. This will alter the process of the heat exchange between the surface and the atmosphere, resulting in evaporation increase, surface temperature rise, and seasonally freezing depth increase, etc. In addition, due to the change of surface water runoff conditions, the water infiltration will lead to the drying in land surface, and the trend of the desertification will increase [25-26].
6 Thermal influences from supporting complexes and residential areas
To ensure the safe operation of QTH, some necessary supporting complexes, such as maintenance stations, parking lots, and residential areas, are indispensable. According to the statistics, there are 29 maintenance squad stations, 4 maintenance sites, and 897 road-menders to serve along the QTH. These buildings may inevitably damage, or completely eliminate the vegetation or natural surface, and impact the thermal regime of permafrost. It may also change the balance conditions of the heat exchange between the buildings and the frozen ground, resulting in the ground warming, steadily increasing of the permafrost table depth, and the permafrost thawing around the building complexes and residential areas. Monitoring data in Fenghuoshan Permafrost Station in 1984 showed that the ground temperature at 5 m depth in heated building was 1.5 °C higher than that outside the heated building. Beneath the dining hall of the transportation station built in 1964 at Wudaoliang, a thaw bulb reached a depth of 7.2 m, and in the residential areas at Tuotuohe Riverside, the permafrost had completely thawed [10]. The thermal flow caused by disturbance of the surface can extend laterally between 5 and 10 m. The rising of ground temperatures and the decline of permafrost table around the building complexes and residential areas are much more rapid than in the undisturbed areas. So, it is proved that the permafrost environments have been severely disturbed. In addition, many workers have housed and lived perennially along the QTH, and liquid and solid waste disposal problems have also the possibility to disturb or damage the permafrost environment.
7 Permafrost environment pollution caused by operation and reconstruction
As a lifeline, the QTH mainly services for transporting living materials (about 90% import and export between Golmud and Lhasa), and about 70% vehicles are heavy trucks. Under the alpine and anoxic environment, the vehicles are easy to go wrong on the road of the frost boiling or troubled sections. Much waste liquid and solid for repairing can be seen in many places, and a mass of solid wastes are also left everywhere along the way. These may induce the permafrost environment pollution. Especially in the period of reconstruction, long-fleets of trucks are blocked at the service road to last for about 2-3 d, resulting in plenty of household garbage. These wastes, such as foamed canteen and plastic bag, are abandoned optionally at both sides of highway.
The soil layer along the QTH is dominated by alpine steppe soil and alpine meadow soil with characteristics of rough texture, low-organic content, and strongly saline alkali. It is determined that the soil layer is thin and very sensitive to the environment change. In addition, strong wind or gale weather is frequently met along the QTH, especially from October to May of the next year. Under windy condition, the soils disturbed by vehicles can easily be blowing dusts. On one hand, they can also be a source of sand-dust storm; on the other hand, these dusts might pollute the local glaciers, resulting in the acceleration of the melting of the glaciers.
8 Suggestions for protection of permafrost environment
During the past several decades, the construction and maintenance of QTH have influenced really, or are influencing the changes in permafrost environment. Therefore, how to protect and control the permafrost environment is an urgent and arduous task. The following are recommendations for limiting or mitigating the changes, disturbances to, and unnecessary destructions of the permafrost environments within or adjacent to the Qinghai-Tibet Corridor.
1) Carry out the principle of the comprehensive treatment and overall protection. Put the protection permafrost environment to an important role the same as the economical construction. According to the results of the environmental assessment in cold regions, all kinds of measures beneficial to the protection permafrost should be esign and ring construction, used to the engineering design and construction as much as possible.
2) Carry out the principle of “the more fill less excavation”. The practice shows that the changes in permafrost environment mainly caused by the cutting or excavation are much more destructive than those caused by the filling. Especially, the large area excavation and damage vegetation should be avoided in massive ground-ice and high-ground temperature permafrost regions.
3) Some drainage facilities, such as drainage ditch, and culvert, should be adopted along the roadbed, ensuring no surface water accumulated along the highway, especially the occurrence of industrial and domestic sewage.
4) The buildings should also be selected at those regions with lower ground temperature, poor-ice contents, and sparse vegetation. Since 1980s, the ventilated foundations have been adopted to buildings. This design is an effective mitigation for controlling differential thaw settlement.
5) Strengthen the construction management and reasonable organize construction, avoiding the artificial destruction, and improving the awareness of permafrost environment protection.
9 Conclusions
1) The natural conditions are rigorous on the QTP because of the high elevations, insufficient oxygen, strong wind, and cold air temperature. The ecological environments in these regions are very sensitive and fragile because of the low precipitation, sparse vegetation, strongly saline alkali soil, and warm continual permafrost. The construction of the QTH has caused some disturbance and damage to the permafrost environment.
2) After the construction of the QTH, especially since the black asphalt pavement in 1973, the absorbing heat of the roadbed has been more than released heat in most sections, resulting in the increase of ground temperature, the decline of permafrost table, the formation of thawing core, and the different degradation degrees of the permafrost in some sections.
3) Permafrost is very sensitive to the changes of the climate, hydrology, and surface conditions etc. Especially in ice-rich permafrost regions, once the vegetation is damaged, or completely eradicated, it is not only very difficult to recovery, but also can cause thawing hazards.
4) The operation and construction of the QTH has also caused some other permafrost environment problems. Although these environment pollutions, such as household garbage, and dust, do not directly result in the change in permafrost environment, their long-term indirect effects, in a certain degree, may also disturb the stability of permafrost.
References
[1] CHEN Gui-chen, HUANG Zhi-wei, LU Xue-feng, PENG Min. Characteristics of wetland and its conservation in the Qinghai Plateau [J]. Journal of Glaciology and Geocryology, 2002, 24(3): 254-259. (in Chinese)
[2] KLEIN J A, HARTE J, ZHAO X Q. Experimental warming causes large and rapid species loss, dampened by simulated grazing, on the Tibetan Plateau [J]. Ecology Letters, 2004, 7(12): 1170-1179.
[3] JIN Hui-jun, ZHAO Lin, WANG Shao-ling, JIN Rui. Modes of degradation and thermal regimes of permafrost along the Qinghai–Tibet Highway [J]. Science in China, 2006, 49(11): 1170-1183. (in Chinese)
[4] LI Bing-yuan, GU Guo-an, LI Shu-de. The series of the comprehensive scientific expedition to the Hoh Xil region-physical environment of Hoh Xil region, Qinghai [M]. Beijing: Science Press, 1996. (in Chinese)
[5] LIU Yong-zhi, WU Qing-bai, ZHANG Jian-ming, TONG Chang-jiang, SHEN Zhong-yan. Study on ground temperature field in permafrost regions of Qinghai-Tibet Plateau [J]. Highway, 2000(2): 4-8. (in Chinese)
[6] WU Qing-bai, CHENG Guo-dong, MA Wei. The impact of climate warming on Qinghai-Tibetan Railroad [J]. Science in China: Series D, 2004, 47(Supp.I): 122-130. (in Chinese)
[7] HUO Ming, WANG Shuang-jie, LI Zhu-long, ZHANG Jin-zhao, CHEN Jian-bing. Study on the complete technology of highway construction in permafrost regions [R]. Xi’an: First Highway Design and Research Institute, 2006. (in Chinese)
[8] WU Qing-bai, LIU Yong-zhi, TONG Chang-jiang. Interactions between the permafrost and engineering environments in the cold regions [J]. Journal of Engineering Geology, 2000, 8(3): 281-287. (in Chinese)
[9] NIU Fu-jun, XU Jian, LIN Zhan-ju, WANG Ping. Engineering activity induced environmental hazards in permafrost regions of Qinghai-Tibet Plateau [C]// KANE D L, HINKEL K M. Proceedings of Ninth International Conference on Permafrost. Faribanks, Alasaka: Institute of Northern Engineering University, 2008: 1287-1292.
[10] WANG Shao-ling. Changes of permafrost and its environmental problems along the Qinghai–Tibet Highway [J]. Journal of Chinese Environmental Science, 1993, 13(5): 344-349. (in Chinese)
[11] JIN Hui-jun, YU Qi-hao, WANG Shao-ling, L? Lan-zhi. Changes in permafrost environments along the Qinghai–Tibet engineering corridor induced by anthropogenic activities and climate warming [J]. Cold Regions Science and Technology, 2008, 53(3): 317-333.
[12] WANG Shao-ling, MI Hai-zhen. Changes of permafrost after the pavement of asphaltic surface along the Qinghai–Tibet Highway [J]. Journal of Glaciology and Geocryology, 1993, 15(4): 566-573. (in Chinese)
[13] CHENG Guo-dong. Research on engineering geology of the roadbed in permafrost regions of Qinghai–Xizang Plateau [J]. Quaternary Sciences, 2003, 23(2): 134-139. (in Chinese)
[14] WU Qing-bai, DONG Xian-fu, LIU Yong-zhi. Response of permafrost to climate change and engineering activity along the Qinghai-Tibet Highway [J]. Journal of Glaciology and Geocryology, 2005, 27(1): 50-54. (in Chinese)
[15] WANG Shao-ling, NIU Fu-jun, ZHAO Lin. Thermal stability of roadbed in permafrost region along the Qinghai–Tibet Highway [J]. Cold Region Science and Technology, 2003, 37(1): 25-34.
[16] NAN Zhuo-tong, GAO Ze-shen, LI Shu-xun, WU Tong-hua. Permafrost Changes in the Northern Limit of Permafrost on the Qinghai-Tibet Plateau in the Last 30 Years [J]. ACTA Geographic SINICA, 2003, 58(6): 817-823. (in Chinese)
[17] ZHU Lin-nan, WU Zi-wang, LIU Yong-zhi. Permafrost Degeneration in the East of Tibetan Plateau [J]. Journal of Glaciology and Geocryology, 1995, 17(2): 120-124. (in Chinese)
[18] WANG Gen-xu, YAO Jin-zhong, GUO Zheng-gang, WU Qing-bai, WANG Yi-bo. Changes of permafrost eco-environments under the influences of human activities, and their significance for the construction of the Qinghai–Tibet Railway [J]. Chinese Science Bulletin, 2004, 49(15): 1556-1564. (in Chinese)
[19] WANG Yi-bo, WANG Gen-xu, CHANG Juan. Impacts of human activities on the permafrost environments on the Qinghai–Tibet Plateau [J]. Journal of Glaciology and Geocryology, 2004, 26(5): 523-527. (in Chinese)
[20] BROWN J, GRAVE NA. Physical and thermal disturbance and protection of permafrost [R]. Hanover NH: U.S. Army Cold Regions Research and Engineering Laboratory (CRREL). 1979.
[21] HINZMAN L D, GOERING D J, LI S, KINNEY T C. Numeric simulation of thermokarst formation during disturbance [C]// CRAWFORD R M M. Disturbance and recovery in Arctic lands: an ecological perspective. Dordrecht, the Netherlands: Kluwer Academic Publishers, 1997: 621.
[22] LIN Zhan-ju, NIU Fu-jun, XU Zhi-ying, XU Jian, WANG Ping. Thermal regime of a thermokarst lake and its influence on permafrost, Beiluhe Basin, Qinghai-Tibet Plateau [J]. Permafrost and Periglacial Processes, 2010, 21: 315-324.
[23] LIN Zhan-ju, NIU Fu-jun, LIU Hua, LU Jia-hao. Hydrothermal processes of alpine tundra lakes, Beiluhe basin, Qinghai-Tibet Plateau [J]. Cold Region Science and Technology, 2010, 65: 446- 455.
[24] NIU Fu-jun, CHENG Guo-dong, XIA Hui-ming, MA Wei. Field experiment study on effects of duct-ventilated railway embankment on protecting the underlying permafrost [J]. Cold Regions Science and Technology, 2005, 45: 178-192.
[25] WANG Shao-ling, JIN Hui-jun, LI Shu-xun, ZHAO Ling. Permafrost degradation on the Qinghai–Tibet Plateau and its environmental impacts [J]. Permafrost and Periglacial Processes , 2000, 11(1): 43-53.
[26] CAO Wen-bing, WAN Li, ZHOU Xun, HU Fu-sheng, LI Zhi-ming, LIANG Si-hai. Hydrogeological environmental impacts of suprapermafrost water in the sources of the Yellow River [J]. Hydrogeology and Engineering Geology, 2003, 30(6): 6-10. (in Chinese)
(Edited by YANG Bing)
Foundation item: Project(KZCX2-YW-Q03-04) supported by the Important Orientation Projects of the Chinese Academy of Sciences; Project(41030741) supported by the National Natural Science of China; Project(2010CB434813) supported by the National Basic Research Program of China
Received date: 2010-09-13; Accepted date: 2010-11-24
Corresponding author: NIU Fu-jun, Professor, PhD; Tel: +86-931-4967263; E-mail: niufujun@lzb.ac.cn
- Changes in permafrost environments caused by construction and maintenance of Qinghai-Tibet Highway
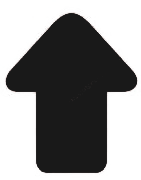