- Abstract:
- 1 Introduction▲
- 2 Materials and methods▲
- 3 Results and discussion▲
- 4 Conclusions▲
- References
- Figure
- Fig.1 Layout of bioleaching experimental apparatus
- Fig.2 Copper and lead extraction after 20 d of bioleaching in acclimation phase
- Fig.3 Effect of shaking speed on cell growth
- Fig.4 Copper extraction from chalcopyrite at different shaking speeds
- Fig.5 Effect of glucose, yeast extract and peptone on copper extraction
- Fig.6 Sulfuric acid consumption during bioleaching of chalcopyrite in stirred tank reactor
J. Cent. South Univ. Technol. (2007)04-0474-05
DOI: 10.1007/s11771-007-0092-2
Bioleaching of chalcopyrite by mixed culture of moderately thermophilic microorganisms
WU Chang-bin(邬长斌)1,2, ZENG Wei-min(曾伟民)2, ZHOU Hong-bo(周洪波)2,3, FU Bo(符 波)2,
HUANG Ju-fang(黄菊芳)3, QIU Guan-zhou(邱冠周)2,3, WANG Dian-zuo(王淀佐)3
(1. China Ocean Mineral Resources Research and Development Association, Beijing 100860, China;
2. School of Resource Processing and Bioengineering, Central South University, Changsha 410083, China;
3. Key Laboratory of Biometallurgy, Ministry of Education, Changsha 410083, China)
____________________________________________________________________________
Abstract:
A mixed culture of moderately thermophilic microorganisms was enriched from acid mine drainages(AMDs) samples collected from several sulphide mines in China, and the bioleaching of chalcopyrite was conducted both in shake flask and bioreactor. The results show that in the shake flask, the mixture can tolerate 50 g/L chalcopyrite after being acclimated to gradually increased concentrations of chalcopyrite. The copper extraction increases obviously in bioleaching of chalcopyrite with moderately thermophilic microorganisms supplemented with 0.4 g/L yeast extract at 180 r/min, 74% copper can be extracted in the pulp of 50 g/L chalcopyrite after 20 d. Compared with copper extractions of mesophilic culture, unacclimated culture and acclimated culture without addition of yeast extract, that of accliniated culture with addition of yeast extract is increased by 53%, 44% and 16%, respectively. In a completely stirred tank reactor, the mass fraction of copper and total iron extraction reach up to 81% and 56%, respectively. The results also indicate that it is necessary to add a large amount of acid to the pulp to extract copper from chalcopyrite effectively.
Key words:
____________________________________________________________________________
1 Introduction
Acidithiobacillus ferrooxidans, Acidithiobacillus thiooxidans and Leptospirillum ferrooxidans, which grow normally between 28 and 40 ℃, are the classical bioleaching microorganisms used in the hydrometallurgical field. Currently, the mesophilic microorganisms have been applied successfully in the leaching of gold, copper, zinc and uranium[1-4]. However, the rate of bioleaching is not so desirable, which is partly due to the fact that the bioleaching-microorganisms cannot absolutely adapt to the complicated leaching circumstances, such as high concentrations of heavy metals, poisoned arsenic ion, and high temperature. Especially, the accumulated heat produced from mineral oxidation causes high temperature in the reactor and some zones in leaching heap, which inhibits the growth of mesophilic microorganisms. On the other hand, bioleaching with mesophilic microorganisms always leads to low bioleaching rate, poor metallic extraction, and it cannot leach some refractory concentrate as chalcopyrite efficiently. All above difficulties make the wide application of bioleaching technique unpractical[5]. Leaching sulphide mines with thermophiles not only highly improves the reaction kinetics, but also avoids excessive passivation of chalcopyrite that hinders the durative bioleaching [6-8]. Since the majorities of extreme thermophiles surviving above 60 ℃ belong to archaea that always lacks typical cell wall and cannot survive in high pulp density that results in strong stirring shear. At present, the application of extreme thermophiles in industry is difficult. Compared with the extreme thermophilic microorganisms, the moderately thermophilic microorganisms can tolerate higher pulp density and have some industrial applications in bioleaching of sulphide minerals[9].
Chalcopyrite is the primary sulphide mineral, which accounts for 70% of copper resource in China. Currently, bioleaching of chalcopyrite has not been applied in industry due to the low leaching speed and poor copper extraction. Since the domestic copper resource is going to be deficient and the demand of copper is increasing continuously, the development of bioleaching process of chalcopyrite is becoming urgent. There are several semi-industrial trials of chalcopyrite bioleaching with moderately thermophilic microorganisms in overseas[10]. In China only a few studies involve in the application of moderately thermophilic microorganisms in bio- leaching[11-12]. Therefore, in this study, a mixed culture of moderately thermophilic microorganisms was enriched from acid mine drainages(AMDs) of several chalcopyrite mines in China and the bioleaching performance of this enrichment was studied. All these are expected to develop a potential bioleaching process for recovering copper from chalcopyrite.
2 Materials and methods
2.1 Microorganisms enrichment
Acid mine drainages(AMDs) from several chalcopyrite mines in China were collected and enriched. The medium used for enrichment consisted of the following compounds: (NH4)2SO4 3.0 g/L, Na2SO4 2.1 g/L, MgSO4?7H2O 0.5 g/L, K2HPO4 0.05 g/L, KCl 0.1 g/L, Ca(NO3)2 0.01 g/L. 10 g/L chalcopyrite was added as the energy source; the samples were enriched at 48 ℃, initial pH 2.0.
2.2 Mineral components
The ore sample was collected from Meizhou in Guangdong Province, China, with diameter less than 75 μm. The composition of the mineral sample is shown in Table 1, indicating that the mineral mainly consists of chalcopyrite and galena.
Table 1 Composition of ore sample
2.3 Acclimation experiments
The acclimation experiments were carried out in shake flasks at 48 ℃. The moderately thermophilic microorganisms incubated with 10 g/L chalcopyrite pulp were filtrated through filter paper, and then inoculated to the above mentioned culture medium with 12 g/L chalcopyrite. 10 mol/L sulfuric acid was used to adjust pH value of the culture medium to 2.0. In the early stage, sulfuric acid was used to adjust the pH to 2.0 for acid consumption; after that, when pH drops to about 1.3 and the cell density reaches more than 108 cell/mL, the cells were collected by removing residual ore solid through filtration, and inoculated in 16 g/L chalcopyrite pulp. Then repeat the acclimation process by increasing the pulp density (21, 28, 37 and 50 g/L) of chalcopyrite. After 90 d, the moderately thermophilic microorganisms obtained high tolerance to 50 g/L chalcopyrite pulp density. At the same time, the controlled experiment of leaching 50 g/L chalcopyrite with unacclimated microorganisms was carried out. After being cultured for 20 d, aliquot of bioleaching liquid was sampled to analyze concentrations of Cu2+ and Pb2+.
2.4 Bioleaching experiments in shake flasks
The tests were carried out in 250 mL shake flasks. In each test, 135 mL culture medium containing 50 g/L chalcopyrite was inoculated with 15 mL culture acclimated in 50 g/L pulp density. The incubation was performed at 48 ℃ and initial pH value of 2.0. The cultures were incubated in rotary shakers at different shaking speeds (140, 160, 180, 200 and 220 r/min). At the optimum shaking speed, glucose, yeast extract and peptone were supplemented with different concentrations (0, 0.4, 0.8, 1.0 and 1.2 g/L). At the optimum shaking speed and optimum addition of organic compounds, the performance of chalcopyrite bioleaching with acclimated moderately thermophilic microorganisms, unacclimated moderately thermophilic microorganisms and mesophilic microorganisms (A. ferrooxidans and A. thiooxidans)were compared. After 20 d of bioleaching, the concentrations of Cu2+, Fe3+ and total iron were analyzed.
2.5 Bioleaching experiments in reactor
Bioleaching experiments were carried out in 3 L glass cylindrical reactor with a mechanic stirrer at 500 r/min. As can be seen in Fig.1, the reactor was placed in a thermostatic bath to keep the constant temperature of 48 ℃. 1 800 mL medium and 0.4 g/L yeast extract were added into the reactor; 200 mL seed culture was inoculated. The experiments were performed at initial pH value of 2.0. The acid consumption was compensated by addition of 10 mol/L sulfuric acid to keep pH value around 2. Distilled water was added to the reactor through a peristaltic pump in order to compensate for evaporation losses. At the end of bioleaching experiments, liquid samples were clarified for the determination of concentrations of Cu2+, Pb2+, Fe3+ and total iron.
Fig.1 Layout of bioleaching experimental apparatus
1-pH meter;2-Glass cylindrical reactor;3-Stirrer;4-Peristaltic pump;5-Thermostat water bath;6-Distilled water
2.6 Analytical methods
The ore sample components were analyzed by X-ray diffractometry. Copper, lead and total iron concentrations in solution were determined by atomic absorption spectrophotometer. Ferrous iron concentration in solution was analyzed by titration with potassium dichromate. The pH value was measured with pH S-3C acid meter. Free cells in solution were observed and counted under an optical microscope.
3 Results and discussion
3.1 Acclimation experiments
Fig.2 shows the copper and lead extraction in acclimation experiments under different pulp densities. As the pulp density increasing, copper concentration improves correspondingly, from 3.1 g/L at 12 g/L pulp density to 7.9 g/L at 50 g/L pulp density. However, the copper extraction efficiency decreases continuously, from 93% at 12 g/L pulp density to 50% pulp density of at 50 g/L. In the whole acclimation process, lead extraction is very low,when pulp density is 50 g/L, the leached lead concentration is only 1.25 mg/L .
Fig.2 Copper and lead extraction after 20 d of bioleaching in acclimation phase
The increase of copper concentration in solutions of different acclimation experiments is due to the increase of pulp density. The decrease of copper extraction rate may be due to that the thermophiles cannot survive in high copper concentration and high pulp density (the extremely low Pb2+ concentration would not be toxic to culture). The quite low concentration of lead may be explained by the fact that galena is refractory at low pH value, and Pb2+ combines with SO42- to form PbSO4 deposition [13]. Compared with the unacclimated culture that only dissolves 30.6% copper in 50 g/L pulp density, the acclimated culture is of rather high tolerance to high pulp density and extracted more copper from chalcopyrite (54%).
3.2 Effect of shaking speed on bioleaching
Figs.3 and 4 show the copper extraction and the free cell densities in shake flasks under different shaking speeds. When shaking speeds are 140 and 160 r/min, the microorganisms grow slowly and the free cell densities are 4.7×107 and 7.1×107 cell/mL, respectively, which results in low copper extraction. At 180 r/min, the maximum biomass is obtained and the copper extraction is quite high (8.1 g/L). When shaking speed increases to 200 and 220 r/min, the growth of microorganisms is restrained. At 220 r/min, the free cell density is only 0.7×107 cells/mL, which results in the lowest copper extraction (4.6 g/L). The results indicate that the increase of shaking speed can enhance mass transfer and thus increase dissolved oxygen and pulp diffusion. However, too rapid revolution results in strong shear, which will restrain the growth of microorganisms, and lead to low copper extraction.
Fig.3 Effect of shaking speed on cell growth
Fig.4 Copper extraction from chalcopyrite at different shaking speeds
3.3 Effect of organic compounds on bioleaching
It has been reported that the addition of appropriate organic compounds could enhance the growth of moderate thermophiles[14-15]. In order to study the effect of organic compounds on the growth and the chalcopyrite bioleaching performance of the moderate thermophiles, three kinds of typical organic compounds glucose, yeast extract and peptone at different concentrations were investigated. The results are shown in Fig.5. After the addition of glucose, the cell density in solution soon decreases (data not shown), which results in low copper extraction. When the concentration of glucose is more than 0.8 g/L, the copper extraction is only about 25%. On the contrary, the addition of appropriate yeast extract and peptone promote copper extraction obviously. When the yeast extract concentrations are 0.4 and 0.8 g/L, the copper extractions both achieve to 10.2 g/L. Compared with the controlled culture without addition of organic compound, the copper extraction rate of the culture with addition of yeast extract increases by 25%. The addition of peptone also leads to increase of dissolved copper. When the peptone addition was 0.4 g/L, the copper extraction rate increases by 20%, reaching 9.65 g/L .However, the addition of too much organic compound would restrain the dissolution of chalcopyrite (Fig.5).
Fig.5 Effect of glucose, yeast extract and peptone on copper extraction
The above results suggest that in the industry, the addition of some nitric organic compounds such as yeast extract and peptone could promote the copper extraction.
3.4 Comparison of chalcopyrite bioleaching capability of mesophiles and moderate thermophiles
In order to investigate the chalcopyrite oxidation capability of the moderate thermophiles, chalcopyrite bioleaching experiments with mesophiles and moderate thermophiles were performed. The tests were carried out in shake flasks under different conditions (Table 2), with initial pH value of 2.0 and pulp density of 50 g/L. After being leached for 20 d, the concentrations of leached copper, total iron and Fe3+ in culture liquid were analyzed and shown in Table 2.
Table 2 Results of chalcopyrite bioleaching in shake flask under different conditions
The acclimated moderately thermophilic microorganisms with 0.4 g/L yeast extract addition shows the strongest capability of leaching chalcopyrite; the copper extraction rate is 74% (10.5 g/L). The acclimated mixed moderately thermophilic microorganisms without addition of yeast extract can leach 8.24 g/L of copper, quite higher than that of the mixed moderately thermophilic microorganisms without acclimation (4.26 g/L), which indicates that the acclimation improves the chalcopyrite leaching capability of the thermophiles. The concentration of copper leached by the mixed mesophilic microorganisms(A. ferrooxidans and A. thiooxidans)is 2.98 g/L, quite lower than that of moderately thermophilic microorganisms. All experiments have low total iron extraction, especially for Fe3+, this may be due to the fact that Fe3+ has partly transformed into jarosite and precipitated, which can be observed when the pulp turns into yellow in the later stage of bioleaching. Table 2 also shows that the culture with high concentration of total iron and Fe3+ has high copper concentration. For example, in the culture inoculated with acclimated mixed moderately thermophilic microorganisms with addition of yeast extract, Fe3+concentration is 2.10 g/L, while copper concentration is 10.5 g/L, both are the highest. Since most of copper is leached by Fe3+ rather than by microorganisms directly, the high concentration of Fe3+ can increase the copper extraction from chalcopyrite.
3.5 Bioleaching experiment in reactor
In order to investigate the potential application in industry, chalcopyrite bioleaching experiment with the acclimated moderately thermophilic mixtures was performed in a completely stirred tank reactor. Meanwhile, the acid consumption was studied. The experiment was performed under the conditions of 48 ℃, initial pH 2.0, and 50 g/L chalcopyrite.
The results show that copper and total iron extractions are improved compared with shake flask experiments. Copper extraction increases from 74% to 81%, and total iron extraction increases from 51% to 57%. Compared with shake flask experiments, 2.87 g/L Fe3+ in the leachate from the reactor is relatively high, which is favorable to copper leaching. The leached lead is 25.2 mg/L, which is quite low and coincides with the results of shake flask experiments.
The mass transfer is enhanced obviously in the reactor as it is completely mixed. This would be beneficial to providing more dissolved oxygen and carbon dioxide and more completed mixture of pulp than those in rotary shaker.
In the experiment, the sulfuric acid consumption is necessary, because the oxidation of chalcopyrite consumes lots of acid, the reaction is shown as follows[6, 16].
CuFeS2+ O2+4H+ → Cu2+ +Fe2+ + 2S0 +2H2O (1)
Acid is also consumed during the oxidation of the released ferrous iron to ferric iron, which is catalyzed by microorganisms during the bioleaching of chalcopyrite.
4Fe2+ + O2+ 4H+ → 4Fe3+ +2H2O (2)
On the other hand, acid will be produced later during the oxidation of sulphur and the hydrolysis of ferric iron to ferric species such as Fe(OH)2+and Fe(OH)2+. Thus the pH value will decrease eventually.
As can be seen in Fig.6, in the first 48 h, a little amount of acid is consumed; from 48 to 132 h, the acid consumption increases quickly, especially from 84 to 108 h, the acid consumption rate approaches the maximum, which is 0.087 g /h on an average; from 132 to 156 h, the acid consumption rate decreases rapidly, and the total consumption of sulfuric acid achieves to the maximum, which is 9.42 g. After 168 h, the pH value decreases to 1.3, this indicates that the amount of acid-production is more than acid-consumption.
Fig.6 Sulfuric acid consumption during bioleaching of chalcopyrite in stirred tank reactor
In the whole process of reactor-bioleaching, the amount of acid consumption is quite large. And the results show that the addition of large amount of acid is necessary for efficient bioleaching of chalcopyrite.
4 Conclusions
1) A moderately thermophilic culture specific to bioleaching of chalcopyrite is obtained from AMDs of several sulphide mines in China. After being acclimated, the mixed thermophiles can tolerate 50 g/L chalcopyrite and dissolved 74% copper from chalcopyrite with the addition of 0.4 g/L yeast extract at 180 r/min in the shake flask, showing stronger chalcopyrite bioleaching capability than mesophiles as A. ferrooxidans and A. thiooxidans.
2) In the stirred tank reactor, the copper extraction of chalcopyrite by the mixed thermophiles further increases to 81%. Furthermore, the addition of lots of acid to the pulp is necessary for efficient bioleaching of chalcopyrite.
References[1] FALCO L, POGLIANI C, CURUTCHET G. A comparison of bioleaching of covellite using pure cultures of Acidithiobacillus ferrooxidans and Acidithiobacillus thiooxidans or a mixed culture of Leptospirillum ferrooxidans and Acidithiobacillus thiooxidan [J]. Hydrometallurgy, 2003, 71(1/2): 31-36.
[2] BEVILAQUA D, LEITE A L L C, GARCIA O. Oxidation of chalcopyrite by Acidithiobacillus ferrooxidans and Acidithiobacillus thiooxidans in shake flasks[J]. Process Biochemistry, 2002, 38(4): 587-592.
[3] SHI Shao-yuan, FANG Zhao-heng. Bioleaching of marmatite flotation concentrate by Acidithiobacillus ferrooxidans[J]. Hydrometallurgy, 2004, 75(1/4): 1-10. (in Chinese)
[4] MAGDALENA G, PAUL C. FRONGER K. Pyrite oxidation by Acidithiobacillus ferrooxidans at various concentrations of dissolved oxygen[J]. Chemical Geology, 2006, 225(1/2):16-29.
[5] PETERSEN J, DIXON D G. Thermophilic heap leaching of a chalcopyrite concentrates[J]. Minerals Engineering, 2002, 15(11): 777-785.
[6] SANDSTORM A, PETERSSON S. Bioleaching of a complex sulphide ore with moderate thermophilic and extreme thermophilic microorganism[J]. Hydrometallurgy, 1997, 46(1/2): 181-190.
[7] GOMES E, BALLESTER A, GONZALEZ F, et al. Leaching capacity of a new extremely thermophilic microorganism, Sulfolobus rivotinct[J]. Hydrometallurgy, 1999, 52(5): 349-366.
[8] GOMEZ E, BALLESTER A, lAZQUEZ M L, et al. Silver-catalysed bioleaching of a chalcopyrite concentrate with mixed cultures of moderately thermophilic microorganisms[J]. Hydrometallurgy, 1999, 51(1): 17-46.
[9] EHRLICH H L. Past, present and future of biohydrometallurgy[J]. Hydrometallurgy, 2001, 59(2): 127-134.
[10] TIPRE D, DAVE S. Bioleaching process for Cu-Pb-Zn bulk concentrates at high pulp density[J]. Hydrometallurgy, 2004, 75(2): 37-43.
[11] DENG Jing-shi, RUAN Ren-man, WEN Jian-kang. Present situation and prospects in bioleaching of sulphides by moderate thermopiles[J]. Multipurpose Utilization of Mineral Resources, 2002, 4(2): 33-38. (in Chinese)
[12] LAN Xing-hua. New development of biological leaching for extraction of gold and base metals[J]. World Nonferrous Metals, 2002(5): 28-32. (in Chinese)
[13] GU Guo-hua, HU Yue-hua, QIU Guang-zhou. Electrochemistry of galena in hight alkaline flotation[J]. Mining and Metallurgical Engineering, 2002, 22(1): 52-55. (in Chinese)
[14] JOHNSON D B, NAOKO O, KEVIN B H. Differentiation and identification of iron-oxidizing acidophilic bacteria using cultivation techniques and amplified ribosomal DNA restriction enzyme analysis[J]. Journal of Microbiological Methods, 2005, 60(3): 299-313.
[15] ROBERTSON W J, MKINNUEN P H, PLUMB J. Moderately thermophilic iron oxidizing bacteria isolated from a pyretic coal deposit showing spontaneous combustion[J]. Minerals Engineering, 2002, 15(4): 815-822.
[16] GOMEZ C, FIGUEROA M, MUNOZ J. A study of bioleached chalcopyrite surfaces in presence of Ag+ by voltammetric methods[J]. Mineral Engineering, 1997, 10(1): 111-116.
______________________
Foundation item: Project(50621063, 40646029) supported by the National Natural Science Foundation of China; Project (2004CB619204) supported by the National Basic Research Program
Received date: 2007-01-15; Accepted date: 2007-03-22
Corresponding author: ZHOU Hong-bo, PhD, associate professor; Tel: +86-731-8877216; E-mail: zhouhb@mail.csu.edu.cn
(Edited by CHEN Wei-ping)
- Bioleaching of chalcopyrite by mixed culture of moderately thermophilic microorganisms
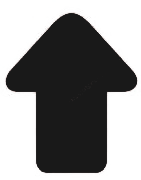