- Abstract:
- 1 Introduction▲
- 2 Experimental proce...▲
- 3 Experimental resul...▲
- 4 Discussion▲
- 5 Conclusions▲
- References
- Figure
- Figure 1 Dimensions of experimental specimen (a) and experimental system (b)
- Figure 2 Layout of acoustic emission sensors
- Figure 3 Relationship between pull-out load, AE counts, AE energy and accumulated AE energy and loading time:
- Figure 4 Relationship between mechanical parameters and pull-out loading rates:
- Figure 5 Relationship between local strain of anchoring interface and pull-out loading rates
- Figure 6 Relationship between AE counts parameters and pull-out loading rates:
- Figure 7 Relationship between accumulated AE energy and pull-out loading rates (a) and between accumulated AE energy before and after maximum pull-out load and pull-out loading rates (b)
- Figure 8 Relationship between AE peak frequency and pull-out loading rates at 1 mm/min (a), 3 mm/min (b), 5 mm/min (c), 7 mm/min (d), 9 mm/min (e) and AE peak frequency distribution (f)
- Figure 9 Relationship between AE events and pull-out loading rates:
- Figure 10 Mohr-Coulomb strength criterion
- Figure 11 Failure characteristics of anchoring interface under different pull-out loading rates:
- Figure 12 Comparison of analytical results and experimental results:
J. Cent. South Univ. (2021) 28: 2052-2066
DOI: https://doi.org/10.1007/s11771-021-4752-4
Effects of different pull-out loading rates on mechanical behaviors and acoustic emission responses of fully grouted bolts
DU Yun-lou(杜云楼)1, 2, 4, FENG Guo-rui(冯国瑞)1, 2, KANG Hong-pu(康红普)3,ZHANG Yu-jiang(张玉江)1, 2, ZHANG Xi-hong(张僖洪)4
1. School of Mining Engineering, Taiyuan University of Technology, Taiyuan 030024, China;
2. Research Center of Green Mining Engineering Technology in Shanxi Province, Taiyuan 030024, China;
3. Mining and Designing Branch, China Coal Research Institute, Beijing 100013, China;
4. Centre for Infrastructural Monitoring and Protection, School of Civil and Mechanical Engineering,Curtin University, Bentley WA 6102, Australia
Central South University Press and Springer-Verlag GmbH Germany, part of Springer Nature 2021
Abstract:
Due to the influence of mining disturbance stress, it is of great significance to better understand the bearing characteristics of fully grouted bolts under different pull-out loading rates. For this purpose, a series of laboratory pull-out tests were conducted to comprehensively investigate the effects of different pull-out loading rates on the mechanical performance and failure characteristics of fully grouted bolts. The results show that the mechanical performance of the anchored specimen presents obvious loading rate dependence and shear enhancement characteristics. With the increase of the pull-out loading rates, the maximum pull-out load increases, the displacement and time corresponding to the maximum pull-out load decrease. The accumulated acoustic emission (AE) counts, AE energy and AE events all decrease with the increase of the pull-out loading rates. The AE peak frequency has obvious divisional distribution characteristics and the amplitude is mainly distributed between 50–80 dB. With the increase of the pull-out loading rates, the local strain of the anchoring interface increases and the failure of the anchoring interface transfers to the interior of the resin grout. The accumulated AE counts are used to evaluate the damage parameter of the anchoring interface during the whole pull-out process. The analytical results are in good agreement with the experimental results. The research results may provide guidance for the support design and performance monitoring of fully grouted bolts.
Key words:
Cite this article as:
DU Yun-lou, FENG Guo-rui, KANG Hong-pu, ZHANG Yu-jiang, ZHANG Xi-hong. Effects of different pull-out loading rates on mechanical behaviors and acoustic emission responses of fully grouted bolts [J]. Journal of Central South University, 2021, 28(7): 2052-2066.
DOI:https://dx.doi.org/https://doi.org/10.1007/s11771-021-4752-41 Introduction
The reinforcement of the roadway surrounding rock is conducive to ensuring the safe mining of coal resources [1-3]. At present, bolt support has become a commonly used reinforcement technique for roadway surrounding rock due to its economic cost, simple installation and good effect. There are three main types of bolt support: end grouted bolts, extended grouted bolts and fully grouted bolts [4]. With the complexity of mining geological conditions, fully grouted bolts have been widely used to reinforce the roadway surrounding rock [5-7]. In order to better realize the support design and performance monitoring of fully grouted bolts, many studies have been conducted to investigate the pull-out capacity and load transfer mechanism of fully grouted bolts, such as theoretical and numerical analysis, laboratory exploration and field verification [8-15]. These studies help us to better understand the fully grouted bolts support technique and promote the development of fully grouted bolts support technique.
In engineering field, due to the influence of mining activities, fully grouted bolts are affected by different stress disturbances [16-18], one of which is different stress loading rates. Many previous studies have focused on the mechanical behavior of rocks under different loading rates [19-21]. LU et al [22] investigated the effects of loading rates on the mechanical properties of coal samples under triaxial compression. YIN et al [23] studied the mechanical behavior of the roof-coal pillar structure under different loading rates and found that the loading rate would affect the mechanical parameters and failure characteristics of coal and rock mass. CHEN et al [24] studied the effect of different loading rates on the Kaiser effect of rock failure. LI et al [25] studied the effect of loading rate on the evolution characteristics of sandstone damage and the results showed that the increase of loading rate would increase the maximum elastic energy before rock failure. ZHANG et al [26] and ZHAO et al [27] studied the effect of loading rate on the morphological characteristics of rock failure fracture surface. MENG et al [28] and MA et al [29] studied the effect of loading rate on the mechanical properties and failure characteristics of rock and found that an increase in loading rate would increase the rock failure degree. CAO et al [30] systematically analyzed the effect of loading rate on the mechanical properties, acoustic emission response and failure characteristics of rock under uniaxial compression.
Through the previous retrospect, the loading rate has a great influence on the mechanical properties and failure characteristics of the rock. However, only limited studies have been conducted on the effect of loading rate on the mechanical properties and failure characteristics of fully grouted bolts. ZHAO et al [31] and CHEN et al [32] found that the pull-out loading rate affected the mechanical performance of the anchoring system. Therefore, it is of great significance to study the effect of loading rate on the mechanical performance and failure characteristics of fully grouted bolts.
Acoustic emission (AE), as a non-destructive testing technique, can effectively evaluate the failure characteristics by capturing acoustic signals. Acoustic emission technique has been widely used to study the structural failure characteristics [33]. SALIBA et al [34] used acoustic emission to study the damage and cracking of reinforced concrete and evaluate its durability. FENG et al [35] used acoustic emission to study the effect of cyclic loading on the failure characteristics of anchoring system. DI et al [36] used acoustic emission to study the failure characteristics of the anchoring interface under different types of bolts. AN et al [37] analyzed the characteristic parameters of acoustic emission during the pull-out process and used the AE parameters to evaluate the damage of the anchoring system. These research results show that the AE characteristics are consistent with the mechanical behavior of the anchoring system during the whole pull-out process and the failure characteristics of the anchoring system can be better evaluated.
A better understanding of the mechanical performance and failure characteristics of fully grouted bolts under different pull-out loading rates is helpful for the support design and performance monitoring. There were few studies on the mechanical performance and failure characteristics of fully grouted bolts under different loading rates. In this paper, the pull-out tests under different pull-out loading rates were conducted to investigate the effects of pull-out loading rates on the mechanical performance and failure characteristics of fully grouted bolts. Meanwhile, the acoustic emission monitoring system and the strain testing system were used to record the response information of the whole pull-out process. The research purpose is to further understand the mechanical performance and failure characteristics of fully grouted bolts and provide scientific guidance for the support design and performance monitoring.
2 Experimental procedure
In order to study the effects of pull-out loading rates on the mechanical performance and failure characteristics of fully grouted bolts, the pull-out tests of short-encapsulated bolt specimens were conducted.
2.1 Specimen preparation
The commonly used threaded steel bolt in coal mines was selected. The bolt length was 200 mm, the bolt diameter was 20 mm and the bolt yield strength is not less than 500 MPa. The bolt rib height was 1.22 mm and the bolt rib spacing was 11.05 mm. The resin grout was a slow resin grout composed of resin and curing agent. The uniaxial compressive strength and shear strength of the slow resin grout were 60 and 35 MPa, respectively. The initial setting time of the slow resin grout at room temperature was 3-5 min. The galvanized steel pipe was used to simulate the surrounding rock. This method has been widely accepted and used in laboratory anchoring experiments [38-39]. The thickness of the galvanized steel pipe was 4 mm, the inner and outer diameters of the galvanized steel pipe were 30 and 38 mm, respectively. The length of the galvanized steel pipe was 100 mm.
The preparation of the experimental specimen was divided into the steps that follow: 1) The slow resin grout was prepared according to a fixed ratio and stirred evenly with an electric drill; 2) The galvanized steel pipe and the bolt were assembled on the plexiglass plate with positioning hole, and the slow resin grout was injected into the galvanized steel pipe; 3) The preparation of the anchored specimen was finished and the specimen was maintained at room temperature for 3 d. It should be pointed out that the outer wall of the galvanized steel pipe was beaten continuously to make the slow resin grout fill the whole galvanized steel pipe during the injection process. Figure 1 shows the dimensions of the experimental specimen and the experimental system.
2.2 Experimental system
The experimental system mainly consisted of three parts. The electro-hydraulic AWA-1000 testing machine manufactured by Jinli Testing Technology Co., Ltd. (Jilin, China) was applied to conduct the pull-out experiment. The CM-2B strain testing instrument manufactured by Xinheng Electronic Technology Co., Ltd. (Qinhuangdao, China) was used to record the strain data. The DS2-8B full information acoustic emission instrument manufactured by Beijing Softland Times Scientific & Technology Co. Ltd. (Beijing, China) was used to collect the acoustic signals produced during the pull-out experiment. In the following, the experimental system is described in detail. During the pull-out experiment, the pull-out testing system, the AE monitoring system and the strain testing system maintained the same data acquisition frequency.
1) Pull-out testing system
The pull-out testing system is composed of a WAW-1000 electro-hydraulic servo testing machine and an auxiliary device. The WAW-1000 electro-hydraulic servo testing machine includes a control system, a loading system and an automatic data acquisition system. The auxiliary device is made of high strength steel plate with a thickness of 20 mm, which was to facilitate the anchored specimen installation. In this study, the displacement loading mode was adopted and the pull-out loading rates were 1, 3, 5, 7 and 9 mm/min, respectively.
2) AE monitoring system
According to the method for laboratory AE monitoring suggested by the International Society for Rock Mechanics and Rock Engineering [40],four acoustic emission sensors with a diameter of 18 mm were arranged on the surface of the anchored specimen. The linking line of sensor 1 and sensor 2 was mutually vertical to that of sensor 3 and sensor 4. The sensors were arranged on the surface of the anchored specimen by magnetic bases. Silicon grease was used as the coupling agent between the AE sensors and the anchored specimen to enhance the connections and to improve the signal transmission. Before the experiment, a pencil lead break testing was performed to ensure that the sensors had high accuracy. The data acquisition frequency was 2.5 MHz. The amplitude threshold was set to 40 dB to shield the noise signals. The layout of the AE sensors is shown in Figure 2.
Figure 1 Dimensions of experimental specimen (a) and experimental system (b)
Figure 2 Layout of acoustic emission sensors
3) Strain testing system
The strain testing system was composed of a CM-2B strain testing apparatus and a strain gauge. Before the experiment, the multimeter was used to check the sensitivity of the strain gauge. The strain gauge was placed in the middle of the anchored specimen surface with cyanoacrylate.
3 Experimental results
In this section, the effects of pull-out loading rates on the mechanical performance and AE responses of fully grouted bolts are analyzed in detail.
3.1 Effects on mechanical performance
The black curves in Figure 3 shows the pull-out load-time characteristics with different pull-out loading rates. The main effects of pull-out loading rates on the mechanical performance are as follows:
1) The general trend of the pull-out load-time curve is basically similar. It can be divided into four stages [41]: I) Chemical debonding stage; II) Micro-cracks development stage; III) Shear slip stage; IV) Friction slip stage. In the chemical debonding stage, the pull-out load increases slowly and non-linearly. The bearing capacity of the anchored specimen mainly depends on the chemical bonding effect between the bolt and the resin grout. In the micro-cracks development stage, the pull-out load increases approximately linearly and gradually reaches the maximum pull-out load. The micro-cracks gradually appear and develop at the anchoring interface. In the shear slip stage, the pull-out load decreases non-linearly. Shear failure occurs at the anchoring interface and the mechanical interlocking effect of the anchoring interface gradually disappears. In the friction slip stage, the pull-out load decreases steadily and the bearing capacity of the anchored specimen mainly depends on the friction effect of the anchoring interface. With the increase of the pull-out loading rates, the slope of the pull-out load-time curve before the maximum pull-out load increases. When enough micro-cracks are formed at the anchoring interface, the anchoring interface fails and the pull-out load decreases rapidly.
2) With the increase of the pull-out loading rates, the maximum pull-out load increases and the time required to reach the maximum pull-out load also decreases, as shown in Figure 4(a). The increase in the pull-out loading rates results in insufficient time for the anchoring interface to release the accumulated energy and accelerates the failure of the anchoring interface. The durability of the anchored specimen is also greatly reduced.
3) With the increase of the pull-out loading rates, the displacement of the maximum pull-out load decreases and the shear stiffness of the anchoring interface increases, as shown in Figure 4(b). The mechanical behavior of the anchoring interface presents obvious loading rate dependence and shear enhancement characteristics.
4) During the whole pull-out process, the local strain of the anchoring interface tends to increase first and then decrease, as shown in Figure 5. With the increase of the pull-out loading rates, the local strain of the anchoring interface increases significantly. The dilatancy effect of the anchoring interface is more obvious due to the increase of the pull-out loading rates.
Figure 3 Relationship between pull-out load, AE counts, AE energy and accumulated AE energy and loading time:
Figure 4 Relationship between mechanical parameters and pull-out loading rates:
Figure 5 Relationship between local strain of anchoring interface and pull-out loading rates
3.2 Effects on acoustic emission responses
During the whole pull-out process, a real-time AE monitoring was conducted to investigate the failure characteristics of fully grouted bolts under different pull-out loading rates. The AE counts, AE energy, AE frequency and AE events were analyzed in detail. The specific AE parameters characteristics are shown in Figure 3. In Figure 3, the red curves represents the AE counts characteristics, the blue and green curves in Figure 3 represent the AE energy and accumulated AE energy characteristics.
3.2.1 AE counts at different pull-out loading rates
AE counts reflect the active degree of acoustic emission during the pull-out process [42]. It can be seen from Figure 3 that the pull-out loading rates have great influence on the acoustic emission responses of the fully grouted bolts. In the chemical debonding stage, the AE counts are relatively small. The AE activity is in a relatively quiet period. However, the rapid chemical debonding of the anchoring interface due to the increase of the pull-out loading rates can cause fluctuations of the AE counts. During the micro-cracks development stage, the AE counts become active due to the development of micro-cracks at the anchoring interface. It is interesting that the AE counts increase significantly near the beginning and ending positions of the micro-cracks development stage, which may provide guidance for the performance monitoring of fully grouted bolts. In the shear slip stage, the AE counts decrease because the micro-cracks at the anchoring interface have fully developed. However, due to the shear failure of the anchoring interface, the AE counts fluctuate significantly. It should be noted that with the increase of the pull-out loading rates, the active degree of the AE counts during the micro-cracks development stage decreases, while the active degree of the AE counts during the shear slip stage increases. This is because the increase in the pull-out loading rates results in insufficient time for the anchoring interface to release the accumulated energy during the pull-out process, which weakens the development of micro-cracks at the anchoring interface. In the friction slip stage, the AE counts is in a low state due to the friction of the anchoring interface.
Figure 6 shows the relationship between the accumulated AE counts, average AE counts and the pull-out loading rates. With the increase of the pull-out loading rates, the accumulated AE counts decrease and the average AE counts increase. This indicates that the increase of the pull-out loading rates can reduce the AE responses of the anchoring interface, but can accelerate the failure process of the anchoring interface [43]. When the pull-out loading rate is small, the energy accumulated in the anchoring interface during the bearing process has enough time to release and the micro-cracks of the anchoring interface can fully develop; when the pull-out loading rate is large, the energy accumulated in the anchoring interface during the bearing process can not be released in time and the micro-cracks of the anchoring interface also can not be fully developed. Moreover, the average AE counts reflect the severity of the failure process of the anchoring interface [37]. The increase of the pull-out loading rates can significantly reduce the durability of the fully grouted bolts and the failure process of the anchoring interface becomes severe. Therefore, with the increase of the pull-out loading rates, the accumulated AE counts decrease and the average AE counts increase.
Figure 6 Relationship between AE counts parameters and pull-out loading rates:
3.2.2 AE energy at different pull-out loading rates
AE energy reflects the intensity of acoustic emission activity and can be used to evaluate the ability of structures to resist failure [44, 45]. It can be seen from Figure 3 that the evolution law of AE energy is basically similar to that of AE counts. In the chemical debonding stage, the AE energy is small. The accumulated AE energy increases slowly. In the micro-cracks development stage, the AE energy increases and the accumulated AE energy shows a non-linear and rapid growth trend. In the shear slip stage, the AE energy gradually decreases. The accumulated AE energy continues to increase, but the growth rate gradually decreases. In the friction slip stage, the AE energy is at a low level and the accumulated AE energy increases steadily. Figure 7 shows the relationship between the accumulated AE energy and the pull-out loading rates. With the increase of the pull-out loading rates, the accumulated AE energy decreases. Moreover, the accumulated AE energy before the maximum pull-out load gradually decreases with the increase of the pull-out loading rates, while the accumulated AE energy after the maximum pull-out load is basically unchanged.
In order to evaluate the effect of the pull-out loading rates on the ability of fully grouted bolts to resist failure, a resistance failure factor was introduced, η=Em/Et, where Em represents the accumulated AE energy before the maximum pull-out load and Et represents the total accumulated AE energy. According to the experimental results,when the pull-out loading rate is 1 mm/min, the resistance failure factor is 0.60; when the pull-out loading rate is 3 mm/min, the resistance failure factor is 0.52; when the pull-out loading rate is 5 mm/min, the resistance failure factor is 0.44; when the pull-out loading rate was 7 mm/min, the resistance failure factor is 0.39; when the pull-out loading rate was 9 mm/min, the resistance failure factor is 0.30. The resistance failure factor decreases significantly with the increase of the pull-out loading rates. This indicates that increasing the pull-out loading rates reduces the ability of fully grouted bolts to resist failure.
Figure 7 Relationship between accumulated AE energy and pull-out loading rates (a) and between accumulated AE energy before and after maximum pull-out load and pull-out loading rates (b)
3.2.3 AE frequency at different pull-out loading rates
Figure 8 shows the AE peak frequency and amplitude characteristics at different pull-out loading rates. During the whole pull-out process, the AE peak frequency has obvious divisional distribution characteristics, which are mainly distributed between 0–200 kHz and 250–325 kHz. The amplitude is mainly distributed between 50–80 dB. Generally, the AE peak frequency increases near the peak shear strength of the rock specimen [46]. Therefore, the higher AE peak frequency mainly appears near the maximum pull-out load, while the lower AE peak frequency mainly appears before and after the maximum pull-out load. With the increase of the pull-out loading rates, the fraction of AE peak frequency in the range of 0-100 kHz gradually decreases, the fraction of AE peak frequency in the range of 100-200 kHz and 200-300 kHz gradually increases and the fraction of AE peak frequency greater than 300 kHz is basically unchanged. With the increase of the pull-out loading rates, the micro-cracks of the anchoring interface can not fully develop, which may affect the distribution characteristics of the AE peak frequency.
Figure 8 Relationship between AE peak frequency and pull-out loading rates at 1 mm/min (a), 3 mm/min (b), 5 mm/min (c), 7 mm/min (d), 9 mm/min (e) and AE peak frequency distribution (f)
3.2.4 AE events at different pull-out loading rates
The AE events reflect the development characteristics of micro-cracks at the anchoring interface during the pull-out process. The three-dimensional (3D) distribution of AE events was investigated in detail, as shown in Figure 9. The color of the sphere represents the occurrence time of the AE event and the size of the sphere represents the energy of the AE event. The distribution of AE events has obvious spatiotemporal characteristics. Under the pull-out load, the micro-cracks of the anchorage interface first appear near the loading end and then gradually expands to the free end. With the increase of the pull-out loading rates, the accumulated AE events decrease. It shows that the increase of pull-out loading rates leads to insufficient development of micro-cracks at the anchoring interface and the proportion of high energy AE events tends to increase.
4 Discussion
4.1 Bearing mechanism at different pull-out loading rates
During the pull-out process, the mechanical behavior of the anchoring interface can be considered to satisfy the Mohr-Coulomb strength criterion [47], as shown in Figure 10. Therefore, the maximum shear stress τm of the anchoring interface under the pull-out load can be expressed as:
(1)
where C represents the cohesion of the anchoring interface; represents the initial normal stress of the anchoring interface;
represents the maximum additional normal stress of the anchoring interface and φ represents the friction angle of the anchoring interface. According to the principle of equivalent stiffness, the additional normal stress
during the pull-out process can be expressed as:
(2)
where K represents the normal confining stiffness of the anchoring body; ε represents the local strain of the anchoring interface. Based on the experimental results, the maximum shear stress τm of the anchoring interface can be acquired [48]:
(3)
where Pm represents the maximum pull-out load; db represents the bolt diameter; l represents the bonding length. Combining Eqs. (1)-(3), the maximum pull-out load can be expressed as:
(4)
When other parameters are constant, the local strain of the anchoring interface directly determines the maximum pull-out load. With the increase of the pull-out loading rates, the local strain of the anchoring interface increases, so the maximum pull-out load of the fully grouted bolts also increases. However, the increase in the pull-out loading rates accelerates the failure of the anchoring interface. The increase of the additional normal stress at the anchoring interface also causes the resin grout and the rock to bear higher compressive stress. Figure 11 shows the failure characteristics of the anchoring interface under different pull-out loading rates. It shows that with the increase of the pull-out loading rates, the failure of the anchoring interface gradually changes from the interval shear failure to the overall shear failure and the failure of the anchoring interface transfers to the interior of the resin grout. This may provide guidance for the bolt support in the high-stress soft rock roadways.
Figure 9 Relationship between AE events and pull-out loading rates:
Figure 10 Mohr-Coulomb strength criterion
4.2 Damage characteristics at different pull-out loading rates
Generally, it is necessary to evaluate the damage degree of fully grouted bolts in the engineering field. The AE monitoring can reflect the mechanical responses of the anchoring interface in real time during the pull-out process. Therefore, the AE parameters, especially the AE counts, can be used to evaluate the damage evolution characteristics of fully grouted bolts [49]. In this paper, the damage parameter was introduced to characterize the failure characteristics of the fully grouted bolts during the bearing process. Based on the accumulated AE counts, the damage parameter can be expressed as:
Figure 11 Failure characteristics of anchoring interface under different pull-out loading rates:
(5)
where Ns represents the accumulated AE counts when the pull-out displacement is at a certain value; Nt represents the total accumulated AE counts when the anchored specimen completely fails. Through analyzing the experimental results, the relationship between the accumulated AE counts N and the pull-out displacement s can be well expressed by the Boltzmann function [50]:
(6)
where A1, A2, B and C are constant that can be determined by experiments. Combining Eqs. (5) and (6), for a specific anchored specimen, the total accumulated AE counts when the anchored specimen completely fails can be considered as a constant. Therefore, the damage parameter D of the anchored specimen at any pull-out displacement s can be acquired:
(7)
Equation (7) shows the relationship between the damage parameter and the pull-out displacement, where M1 and M2 are constant that can be determined by experiments. Figure 12 shows the comparison between the analytical results and the experimental results under different pull-out loading rates. It can be seen from Figure 12 that the damage characteristics of fully grouted bolts can be roughly divided into three stages: I) Fast damage stage. The damage parameter increases rapidly with the increase of the pull-out displacement; II) Stable damage stage. The damage parameter increases steadily with the increase of the pull-out displacement; and III) Slow damage stage. The damage parameter increases slowly with the increase of the pull-out displacement. The analytical results of the damage parameter are in good agreement with the experimental results. This may provide guidance for the performance monitoring of fully grouted bolts.
5 Conclusions
This study aims to investigate the effects of different pull-out loading rates on the mechanical behaviors and failure characteristics of fully grouted bolts. A series of laboratory pull-out tests were conducted, and the mechanical performance, AE responses and failure characteristics were thoroughly analyzed. The main conclusions are as follows:
1) The mechanical behavior of the anchoring interface presents obvious loading rate dependence and shear enhancement characteristics. With the increase of the pull-out loading rates, the maximum pull-out load increases and the corresponding displacement and time both decrease.
2) The AE response is significantly affected by the pull-out loading rates. With the increase of the pull-out loading rates, the accumulated AE counts, accumulated AE energy and accumulated AE events both decrease. Increasing the pull-out loading rates will reduce the ability of the anchoring interface to resist failure and accelerate the failure of the anchoring interface. The AE peak frequency is mainly distributed between 0–200 kHz and 250–325 kHz and the amplitude is mainly distributed between 50–80 dB.
3) With the increase of the pull-out loading rates, the local strain of the anchoring interface increases. The failure of the anchoring interface gradually changes from the interval shear failure to the overall shear failure and the failure of the anchoring interface transfers to the interior of the resin grout.
4) The accumulated AE counts are used to evaluate the damage parameter of the anchoring interface during the pull-out process. The analytical results are in good agreement with the experimental results. The damage evolution characteristics of the anchoring interface can be divided into three stages: fast damage stage, stable damage stage and slow damage stage.
5) The research results may provide guidance for the support design and performance monitoring of fully grouted bolts in coal mine roadways.
Contributors
DU Yun-lou conducted the experiments and wrote the first draft of the manuscript. FENG Guo-rui and KANG Hong-pu provided the concept and edited the draft of manuscript. ZHANG Yu-jiang and ZHANG Xi-hong edited the draft of manuscript.
Conflict of interest
DU Yun-lou, FENG Guo-rui, KANG Hong-pu, ZHANG Yu-jiang and ZHANG Xi-hong declare that they have no conflict of interest.
Figure 12 Comparison of analytical results and experimental results:
References
[1] KANG Hong-pu. Sixty years development and prospects of rock bolting technology for underground coal mine roadways in China [J]. Journal of University of Mining & Technology, 2016, 45(6): 1071-1081. DOI: 10.13247/j.cnki.jcumt.000583. (in Chinese)
[2] YI Kang, KANG Hong-pu, JU Wen-jun, LIU Yue-dong, LU Zhi-guo. Synergistic effect of strain softening and dilatancy in deep tunnel analysis [J]. Tunnelling and Underground Space Technology, 2020, 97: 103280. DOI: 10.1016/j.tust.2020. 103280.
[3] KANG Hong-pu, WANG Guo-fa, JIANG Peng-fei, WANG Jia-chen, ZHANG Nong, JING Hong-wen, HUANG Bingxiang, YANG Bao-gui, GUAN Xue-mao, WANG Zhi-gen. Conception for strata control and intelligent mining technology in deep coal mines with depth more than 1000 m [J]. Journal of China Coal Society, 2018, 43: 1789-1800. DOI: 10.13225/j.cnki.jccs.2018.0634.(in Chinese)
[4] KANG Hong-pu, XU Gang, WANG Biao-mou, WU Yongzheng, JIANG Peng-fei, PAN Jun-feng, REN Huai-wei, ZHANG Yu-jun, PANG Yi-hui. Forty years development and prospects of underground coal mining and strata control technologies in China [J]. Journal of Mining and Strata Control Engineering, 2019, 1(1): 013501. (in Chinese)
[5] ZHENG Xi-gui, FENG Xiao-wei, ZHANG Nong, GONG Lian-yuan, HUA Jin-bo. Serial decoupling of bolts in coal mine roadway supports [J]. Arabian Journal of Geosciences, 2015, 8(9): 6709-6722. DOI: 10.1007/s12517-014-1697-z.
[6] FENG Guo-rui, ZHANG Yu-jiang, QI Ting-ye, KANG Li-xun. Status and research progress for residual coal mining in China [J]. Journal of China Coal Society, 2020, 45(1): 151-159. DOI: 10.13225/j.cnki.jccs.YG19.1280. (in Chinese)
[7] LIN Jian, REN Shuo. Numerical simulation optimization research of bolt profile configuration with resin full-length anchoring [J]. Journal of Mining & Safety Engineering, 2015, 32(2): 273-278. DOI: 10.13545/j.cnki.jmse.2015.02.016. (in Chinese)
[8] MARTIN L B, TIJANI M, HADJ-HASSEN F. A new analytical solution to the mechanical behaviour of fully grouted rockbolts subjected to pull-out tests [J]. Construction and Building Materials, 2011, 25(2): 749-755. DOI: 10.1016/j.conbuildmat.2010.07.011.
[9] CHEN Jian-hang, SAYDAM S, HAGAN P C. An analytical model of the load transfer behavior of fully grouted cable bolts [J]. Construction and Building Materials, 2015, 101: 1006-1015. DOI: 10.1016/j.conbuildmat.2015.10.099.
[10] HE L, AN X M, ZHAO Z Y. Fully grouted rock bolts: An analytical investigation[J]. Rock Mechanics and Rock Engineering, 2015, 48(3): 1181-1196. DOI: 10.1007/s00603-014-0610-0.
[11] MA Shu-qi, ZHAO Zhi-ye, NIE Wen, GUI Yi-lin. A numerical model of fully grouted bolts considering the tri-linear shear bond–slip model [J]. Tunnelling and Underground Space Technology, 2016, 54: 73-80. DOI: 10.1016/j.tust.2016.01. 033.
[12] LI Shu-cai, WANG Hong-tao, WANG Qi, JIANG Bei, WANG Fu-qi, GUO Nian-bo, LIU Wen-jiang, REN Yao-xi. Failure mechanism of bolting support and high-strength bolt-grouting technology for deep and soft surrounding rock with high stress [J]. Journal of Central South University, 2016, 23(2): 440-448. DOI: 10.1007/s11771-016-3089-x.
[13] ZOU Jin-feng, ZHANG Peng-hao. Analytical model of fully grouted bolts in pull-out tests and in situ rock masses [J]. International Journal of Rock Mechanics and Mining Sciences, 2019, 113: 278-294. DOI: 10.1016/j.ijrmms.2018. 11.015.
[14] CUI Jian-guo, ZHANG Chuan-qing, CHEN Jian-lin, YANG Fan-jie, ZHOU Hui, LU Jing-jing. Effect of bolt inclination angle on shear behavior of bolted joints under CNL and CNS conditions [J]. Journal of Central South University, 2020, 27(3): 937-950. DOI: 10.1007/ s11771-020-4342-x.
[15] SAADAT M, TAHERI A. Effect of contributing parameters on the behaviour of a bolted rock joint subjected to combined pull-and-shear loading: A DEM approach [J]. Rock Mechanics and Rock Engineering, 2020, 53(1): 383-409. DOI: 10.1007/s00603-019-01921-6.
[16] KANG H, WU Y, GAO F, JIANG P, CHENG P, MENG X, LI Z. Mechanical performances and stress states of rock bolts under varying loading conditions [J]. Tunnelling and Underground Space Technology, 2016, 52: 138-146. DOI: 10.1016/j.tust.2015.12.005.
[17] ZHANG Hou-quan, MIAO Xie-xing, ZHANG Gui-min, WU Yu, CHEN Yan-long. Non-destructive testing and pre-warning analysis on the quality of bolt support in deep roadways of mining districts [J]. International Journal of Mining Science and Technology, 2017, 27: 989-998. DOI: 10.1016/j.ijmst. 2017.06.028.
[18] TAHMASEBINIA F, ZHANG Cheng-guo, CANBULAT I, VARDAR O, SAYDAM S. Numerical and analytical simulation of the structural behaviour of fully grouted cable bolts under impulsive loading [J]. International Journal of Mining Science and Technology, 2018, 28: 807-811. DOI: 10.1016/j.ijmst.2018.08.012.
[19] WENG Lei, LI Xi-bing, TAHERI A, WU Qiu-hong, XIE Xiao-feng. Fracture evolution around a cavity in brittle rock under uniaxial compression and coupled static-dynamic loads [J]. Rock Mechanics and Rock Engineering, 2018, 51(2): 531-545. DOI: 10.1007/s00603-017-1343-7.
[20] GHASEMI S, KHAMEHCHIYAN M, TAHERI A, NIKUDEL M R, ZALOOLI A. Crack evolution in damage stress thresholds in different minerals of granite rock [J]. Rock Mechanics and Rock Engineering, 2019: 1-16. DOI: 10.1007/s00603-019-01964-9.
[21] CAO Shuai, YILMAZ E, SONG Wei-dong, YILMAZ E, XUE Gai-li. Loading rate effect on uniaxial compressive strength behavior and acoustic emission properties of cemented tailings backfill [J]. Construction and Building Materials, 2019, 213: 313-324. DOI: 10.1016/j.conbuildmat.2019.04. 082.
[22] LU Jun, ZHANG Dong-ming, HUANG Gun, LI Xing, GAO Heng, YIN Guang-zhi. Effects of loading rate on the compound dynamic disaster in deep underground coal mine under true triaxial stress [J]. International Journal of Rock Mechanics and Mining Sciences, 2020, 134: 104453. DOI: 10.1016/j.ijrmms.2020.104453.
[23] YIN Da-wei, CHEN Shao-jie, XING Wen-bin, HUANG Dong-mei, LIU Xing-quan. Experimental study on mechanical behavior of roof-coal pillar structure body under different loading rates [J]. Journal of China Coal Society, 2018, 43(5): 1249-1257. DOI: 10.13225/j.cnki.jccs.2017.1091. (in Chinese)
[24] CHEN Yu-long, ZHANG Yu. Influence of loading rate on the Kaiser effect for different lithological rocks [J]. Journal of China Coal Society, 2018, 43: 959-966. DOI: 10.13225/j.cnki.jccs.2017.1003. (in Chinese)
[25] LI Shu-gang, CHEN Gao-feng, SHUANG Hai-qing, LIN Hai-fei, ZHAO Peng-xiang. Experimental study on effect of loading rate and initial damage on energy evolution of sandstone [J]. Journal of Mining and Safety Engineering, 2019, 36: 373-380. DOI: 10.13545/j.cnki.jmse.2019.02.021. (in Chinese)
[26] ZHANG Qian-bing, ZHAO Jian. Effect of loading rate on fracture toughness and failure micromechanisms in marble [J]. Engineering Fracture Mechanics, 2013, 102: 288-309. DOI: 10.1016/j.engfracmech.2013.02.009.
[27] ZHAO Peng-xiang, LI Shu-gang, HO C H, LIN Hai-fei. Crack propagation and material characteristics of rocklike specimens subject to different loading rates [J]. Journal of Materials in Civil Engineering, 2019, 31(7): 04019113. DOI: 10.1061/ (ASCE)MT.1943-5533.0002768.
[28] MENG Qing-bin, ZHANG Ming-wei, HAN Li-jun, PU Hai, CHEN Yan-long. Experimental research on the influence of loading rate on the mechanical properties of limestone in a high-temperature state [J]. Bulletin of Engineering Geology and the Environment, 2019, 78: 3479-3492. DOI: 10.1007/ s10064-018-1332-4.
[29] MA Hong-fa, SONG Yan-qi, CHEN Shao-jie, LI Xiao-long, FENG Fan. Experimental study on effects of loading rate and sample size on the mechanical and failure characteristics of mudstone [J]. Geotechnical and Geological Engineering, 2020: 1-10. DOI: 10.1007/s10706-019-01182-8.
[30] CAO An-ye, JING Guang-cheng, DING Yan-lu, LIU Sai. Mining-induced static and dynamic loading rate effect on rock damage and acoustic emission characteristic under uniaxial compression [J]. Safety Science, 2019, 116: 86-96. DOI: 10.1016/j.ssci.2019.03.003.
[31] ZHAO Tong-bin, GUO Wei-yao, YIN Yan-chun, TAN Yun-liang. Bolt pull-out tests of anchorage body under different loading rates [J]. Shock and Vibration, 2015, 2015: 121673. DOI: 10.1155/2015/121673.
[32] CHEN Chang-fu, LIANG Guan-ting, TANG Yu, XU You-lin. Anchoring solid-soil interface behavior using a novel laboratory testing technique [J]. Chinese Journal of Geotechnical Engineering, 2015, 37: 1115-1122. DOI: 10.11779/CJGE201506018. (in Chinese)
[33] ZHOU Zi-long, CHENG Rui-shan, CHEN Lian-jun, ZHOU Jing, CAI Xin. An improved joint method for onset picking of acoustic emission signals with noise [J]. Journal of Central South University, 2019, 26(10): 2878-2890. DOI: 10.1007/s11771-019-4221-5.
[34] SALIBA J, MEZHOUD D. Monitoring of steel-concrete bond with the acoustic emission technique [J]. Theoretical and Applied Fracture Mechanics, 2019, 100: 416-425. DOI: 10.1016/j.tafmec.2019.01.034.
[35] FENG Xiao-wei, ZHANG Nong, WEN Zhi-jie. Mechanical responses and acoustic emission properties of bolting system under short encapsulation cyclic thrust tests [J]. International Journal of Fatigue, 2019, 121: 39-54. DOI: 10.1016/ j.ijfatigue.2018.11.026.
[36] DI Bo, WANG Jing-kai, LI Hao-tian, ZHENG Jin-hang, ZHENG Yu, SONG Gang-bing. Investigation of bonding behavior of FRP and steel bars in self-compacting concrete structures using acoustic emission method [J]. Sensors, 2019, 19(1): 159-165. DOI: 10.3390/s19010159.
[37] AN Tie-liang, ZHENG Xi-gui, ZHU Deng-xing, QIAN De-yu, GUO Yu, CAO Jun-cai. Experimental investigation of pretensioned bolts under cyclic loading: Damage assessment using acoustic emission [J]. International Journal of Distributed Sensor Networks, 2019, 15(5): 15501477. DOI: 10.1177/1550147719849354.
[38] BAWDEN W F, HYETT A J, LAUSCH P. An experimental procedure for the in situ testing of cable bolts [J]. International Journal of Rock Mechanics and Mining Sciences & Geomechanics Abstracts, 1992, 29(5): 525-533. DOI: 10.1016/0148-9062(92)92635-P.
[39] HYETT A J, BAWDEN W F, REICHERT R D. The effect of rock mass confinement on the bond strength of fully grouted cable bolts [J]. International Journal of Rock Mechanics and Mining Sciences & Geomechanics Abstracts, 1992, 29(5): 503-524.
[40] ISHIDA T, LABUZ J F, MANTHEI G, MEREDITH P G, NASSERI M H B, SHIN K, YOKOYAMA T, ZANG A. ISRM suggested method for laboratory acoustic emission monitoring [J]. Rock Mechanics and Rock Engineering, 2017, 50(3): 665-674. DOI: 10.1007/s00603-016-1165-z.
[41] DU Yun-lou, FENG Guo-rui, KANG Hong-pu, ZHANG Xi-hong, ZHANG Yu-jiang, SHI Xu-dong, WEN Xiao-ze. Investigation on the mechanical behavior and failure characteristics of fully grouted bolts under tension [J]. Energy Sources, Part A: Recovery, Utilization, and Environmental Effects, 2020: 1-15. DOI: 10.1080/15567036.2020.1829194.
[42] GALLEGO A, BENAVENT-CLIMENT A, SUAREZ E. Concrete-galvanized steel pull-out bond assessed by acoustic emission [J]. Journal of Materials in Civil Engineering, 2016, 28(2): 04015109. DOI: 10.1061/(ASCE)MT.1943-5533.000 1372.
[43] LIANG Zhong-yu, GAO Feng, YANG Xiao-rong, FENG Shu-zhong, LIN Jin-tai. Experimental study of the influence of loading velocity on rock’s acoustic emission signal [J]. Mining Research and Development, 2010, 30(1): 12-14, 95. (in Chinese)
[44] BAI Jin-wen, FENG Guo-rui, WANG Ze-hua, WANG Shan-yong, QI Ting-ye, WANG Peng-fei. Experimental investigations on the progressive failure characteristics of a sandwiched coal-rock system under uniaxial compression [J]. Applied Sciences, 2019, 9(6): 1195. DOI: 10.3390/ app9061195.
[45] QIAN Rui-peng, FENG Guo-rui, GUO Jun, WANG Peng-fei, JIANG Hai-na. Effects of water-soaking height on the deformation and failure of coal in uniaxial compression [J]. Applied Sciences, 2019, 9(20): 4370. DOI: 10.3390/ app9204370.
[46] JIAO Yong-jun, PANG Ming-min, MAO Rui-biao. Peak frequency characteristics of acoustic emission for granite during shear under high triaxial stress [J]. Mining Research and Development, 2017, 37(7): 61-64. (in Chinese)
[47] YOU Chun-an, ZHAN Yu-bao, LIU Qiu-yuan, SUN Lin-lin, WANG Kai-bin. Shear lag-debonding model for anchorage section of prestressed anchor cable [J]. Chinese Journal of Rock and Engineering, 2013, 32(4): 800-806. DOI: 10.3969/j.issn.1000-6915.2013. 04.019. (in Chinese)
[48] MA Shu-qi, NEMCIK J, AZIZ N. An analytical model of fully grouted rock bolts subjected to tensile load [J]. Construction and Building Materials, 2013, 49: 519-526. DOI: 10.1016/ j.conbuildmat.2013.08.084.
[49] TANG Chun-an, XU Xiao-he. Evolution and propagation of material defects and Kaiser effect function [J]. Journal of Seismological Research, 1990, 13(2): 203-213. (in Chinese)
[50] WU Xian-zhen, LIU Jian-wei, LIU Xiang-xin, ZHAO Kui, ZHANG Yan-bo. Study on the coupled relationship between AE accumulative ring-down count and damage constitutive model of rock [J]. Journal of Mining & Safety Engineering, 2015, 32: 28-34, 41. DOI: 10.13545/j.cnki.jmse.2015.01.005. (in Chinese)
(Edited by FANG Jing-hua)
中文导读
不同拉拔加载速率对全长锚固锚杆力学行为和声发射响应的影响
摘要:考虑到采矿扰动应力的影响,更好地理解不同拉拔加载速率下全长锚固锚杆的承载特性具有重要意义。本文采用一系列的实验室拉拔试验研究了不同拉拔加载速率对全长锚固锚杆力学性能和失效特性的影响。结果表明,锚固试件的力学性能表现出明显的加载速率依赖性和剪切增强特性。随着拉拔加载速率的增加,锚固试件的峰值拉拔载荷增大,而峰值拉拔载荷对应的位移和时间却减小,锚固试件的耐久性显著降低。同时,累积的声发射振铃计数、能量和事件数均随着拉拔加载速率的增加而减小。声发射事件的峰值频率呈现出明显的分区集中分布特征,振幅主要分布在50~80 dB的范围内。随着拉拔加载速率的增加,锚固界面的局部应变增大,且锚固界面的失效逐渐由局部剪切失效向整体剪切失效过渡。累积声发射振铃计数可用于评估拉拔过程中锚固界面的损伤特征,且分析结果与实验结果具有较好地一致性。该研究结果可为全长锚固锚杆的支护设计和性能监测提供参考。
关键词:全长锚固锚杆;拉拔试验;加载速率;力学行为;声发射响应;失效特性
Foundation item: Projects(51925402, U1710258, 52004172) supported by the National Natural Science Foundation of China; Project(20201102004) supported by the Science and Technology Department of Shanxi Province, China
Received date: 2020-04-22; Accepted date: 2020-10-20
Corresponding author: FENG Guo-rui, PhD, Professor; Tel: +86-351-6010177; E-mail: fguorui@163.com; ORCID: https://orcid.org/ 0000-0001-5359-8294
Abstract: Due to the influence of mining disturbance stress, it is of great significance to better understand the bearing characteristics of fully grouted bolts under different pull-out loading rates. For this purpose, a series of laboratory pull-out tests were conducted to comprehensively investigate the effects of different pull-out loading rates on the mechanical performance and failure characteristics of fully grouted bolts. The results show that the mechanical performance of the anchored specimen presents obvious loading rate dependence and shear enhancement characteristics. With the increase of the pull-out loading rates, the maximum pull-out load increases, the displacement and time corresponding to the maximum pull-out load decrease. The accumulated acoustic emission (AE) counts, AE energy and AE events all decrease with the increase of the pull-out loading rates. The AE peak frequency has obvious divisional distribution characteristics and the amplitude is mainly distributed between 50–80 dB. With the increase of the pull-out loading rates, the local strain of the anchoring interface increases and the failure of the anchoring interface transfers to the interior of the resin grout. The accumulated AE counts are used to evaluate the damage parameter of the anchoring interface during the whole pull-out process. The analytical results are in good agreement with the experimental results. The research results may provide guidance for the support design and performance monitoring of fully grouted bolts.
- Effects of different pull-out loading rates on mechanical behaviors and acoustic emission responses of fully grouted bolts
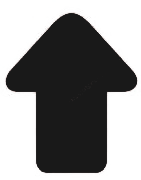