- Abstract:
- 1 Introduction▲
- 2 Experimental▲
- 3 Results and discussion▲
- 4 Conclusions▲
- References
- Figure
- Fig.1 XRD pattern of untreated COPR samples
- Fig.2 SEM photographs of COPR samples before (a) and after (b) being treated by HF acid
- Fig.3 Effect of stirring rate on acid-soluble Cr(VI) leaching efficiency (T=298.15 K; L/S=40; c=6 mol/L; d0=0.105-0.125 mm)
- Fig.4 Effect of leaching temperature on acid-soluble Cr(VI) leaching efficiency (a), plot of leaching kinetics at different temperatures (b) and plot of ln Ka against 1/T (c) (L/S=40; c= 6 mol/L; d0=0.105-0.125 mm; N=600 r/min)
- Fig.5 Effect of HF acid concentration on acid-soluble Cr(VI) leaching efficiency (a), plot of leaching kinetics at different HF acid concentrations (b) and plot of ln Ka against ln c (c) (T=298.15 K; L/S=40; d0=0.105-0.125 mm; N=600 r/min)
- Fig.6 Effect of initial particle size on acid-soluble Cr(VI) leaching efficiency (a), plot of leaching kinetics at different particle sizes (b), plot of Ka against (c) and plot of ln Ka against ln d0 (d) (T=298.15 K; L/S=40; c=6 mol/L; N=600 r/min)
- Fig.7 XRD pattern of treated COPR with HF solution (T= 298.15 K; L/S=40; c=6 mol/L; t=60 min; d0=0.080-0.098 mm; N=600 r/min)
J. Cent. South Univ. Technol. (2011) 18: 399-405
DOI: 10.1007/s11771-011-0710-x
Leaching kinetics of acid-soluble Cr(VI) from chromite ore processing residue with hydrofluoric acid
LI Xiao-bin(李小斌)1, XU Wen-bin(徐文彬)1, 2, ZHOU Qiu-sheng(周秋生)1,
PENG Zhi-hong(彭志宏)1, LIU Gui-hua(刘桂华)1
1. School of Metallurgical Science and Engineering, Central South University, Changsha 410083, China;
2. Shandong Branch, Aluminum Corporation of China Limited, Zibo 255052, China
? Central South University Press and Springer-Verlag Berlin Heidelberg 2011
Abstract:
Leaching kinetics of acid-soluble Cr(VI) in chromite ore processing residue (COPR) using hydrofluoric (HF) acid solution as a leaching agent was investigated for potential remediation of COPR with industrial waste water containing HF. The results show that HF can effectively destabilize the Cr(VI)-bearing minerals, resulting in the mobilization of Cr(VI) from COPR into the leachate. Particle size significantly influences the leaching of acid-soluble Cr(VI) from COPR, followed by leaching time, whereas the effects of HF concentration and leaching temperature are slight and the influence of stirring rate is negligible. The leaching process of acid-soluble Cr(VI) from COPR is controlled by the diffusion through the product layer. The apparent activation energy is 8.696 kJ/mol and the reaction orders with respect to HF concentration and particle size is 0.493 8 and -2.013 3, respectively.
Key words:
leaching; kinetics; hydrofluoric acid; hexavalent chromium; chromite ore processing residue;
1 Introduction
Chromite ore processing residue (COPR) is a solid residue produced by the so-called high lime process. COPR was classified as a hazardous waste for containing 1%-2% hexavalent chromium (Cr(VI)) [1], which is a mutagenic carcinogen. Remediation of COPR is to reduce Cr(VI) to nontoxic trivalent chromium (Cr(III)). However, COPR contains a very complex multi- component system [2], with a variable quantitative composition in each mineral, and has a high pH value, so it is difficult to realize the complete reduction of Cr(VI) to Cr(III). At present, COPR is still mainly deposited. There are two problems observed at COPR disposal sites [3]: one is the persistent leaching of Cr(VI) from COPR and the other is the heaving and uncontrolled expansion. Hence, COPR has become a major geoenvironmental and geotechnical hazard in many urban areas around the world.
Cr(VI) in COPR may be divided into water-soluble Cr(VI) and acid-soluble Cr(VI) in terms of their solubility property. The extraction of acid-soluble Cr(VI) is the key to the remediation of COPR [4]. The majority of acid-soluble Cr(VI) is present in calcium aluminum chromium hydrate (CAC), hydrogarnet, hydrotalcite and ettringite, and a little is in brownmillerite [5-7]. Remediation of COPR by acid-leaching is to destabilize the Cr(VI)-bearing minerals, mobilize the acid-soluble Cr(VI) from COPR into the leachate and subsequently reduce the leached Cr(VI) to Cr(III) by reducing agents in the leachate.
COPR has large pH buffering capacity, with an acid neutralization capacity (ANC) of approximately 13 mol H+ per kilogram dry COPR [5, 8]. Sulfuric acid is usually employed to adjust the pH value of COPR during the hydro-remediation process. Although the sulfuric acid favors the leaching of Cr(VI) in CAC and hydrotalcite [9-10], the uncontrolled expansion of COPR induced by the ettringite formed with a pH value higher than 9, blocks the mass transfer in the leaching process and ultimately impedes the further reduction of Cr(VI) [11-16]. Therefore, it is necessary to search for the substitute of sulfuric acid to treat COPR effectively.
Up to now, less literatures have been involved in treating COPR with hydrofluoric (HF) acid, maybe because HF acid is a virulent reagent, which can burn human’s and animal’s skins and muscles, even erode bones [17-18]. So, it is obviously irrational for the remediation of COPR using merely HF acid. However, if waste water containing HF, such as the industrial effluent discharged from the fluoride salt industry, is used to treat COPR, the pollution of both the industrial waste water and COPR might be controlled.
There is approximately 200 000 t COPR deposited in Xiangxiang county, Hunan Province, China, and there is a fluoride salt factory nearby with annually 600 000 t of waste water containing HF. The average F- concentration of waste water is 0.26-0.53 mol/L and the pH value is 2-4. Therefore, in this work, the leaching kinetics of acid-soluble Cr(VI) from COPR with HF acid as a leaching agent was studied preliminarily in order to find out the rate-limiting step of the leaching process for supplying theory about the next remediation of COPR with this kind of industrial waste water.
2 Experimental
2.1 Materials and reagents
The untreated COPR samples were collected from the COPR disposal site in Hunan Province, China. The elemental composition and XRD pattern of the untreated COPR samples are shown in Table 1 and Fig.1, respectively. The chemical and mineralogical composition of COPR is very complex. The main elements are calcium, magnesium, iron and silicon, and the main minerals are brownmillerite, periclase and calcite. The Cr(VI)-bearing minerals are CAC, hydrogarnet, hydrotalcite and brownmillerite. HF acid is of analytical grade. All solutions were prepared by deionized water.
Tab1e 1 Composition of untreated COPR samples (mass fraction, %)
2.2 Experimental procedure
The untreated COPR samples were ground and screened by sieve with a pore size of 0.9 mm. The COPR samples of <0.9 mm were leached by stirring for 8 h in deionized water in order to leach out almost the water-soluble Cr(VI) from COPR, and the slurry was filtered. The cake was dried at 323.15 K for 24 h, then ground and screened into four different sizes of 0.180- 0.224 mm, 0.105-0.125 mm, 0.098-0.105 mm and 0.080-0.098 mm, in order to examine the possible effect of particle size. The content of acid-soluble Cr(VI) in different sizes of COPR samples is presented in Table 2.
All the experiments were conducted in airtight aerator to avoid the possible damage of HF acid. The COPR samples were leached in a Teflon flask (500 mL) with two openings heated in a water bath and the temperature controlling accuracy was ±0.5 K. For minimizing aqueous loss when the system was heated, a reflux condenser was mounted on the top of one opening. In each batch experiment, 200 mL HF solution of 5, 6, 7, 8, 9 mol/L was added into the flask, respectively. When the temperature of the solution system reached the predetermined value, 5.00 g COPR sample was introduced into the leaching agent and at the same time the mechanical agitator coated with Teflon started working and maintained for a preset reaction period. Then, the slurry was quickly filtered and washed four times with 250 mL boiled deionized water. The cake was dried at 323.15 K for 24 h and conducted for acid-soluble Cr(VI) analyses and X-ray diffraction analyses. All the samples were prepared in duplicate and the average values were reported in this work. The leached efficiency (η) of acid-soluble Cr(VI) is calculated as
(1)
where m0 and m1 are the masses of the untreated or treated COPR sample (g), respectively; w0 and w1 are the contents of acid-soluble Cr(VI) in untreated or treated COPR sample (%), respectively.
Fig.1 XRD pattern of untreated COPR samples
Tab1e 2 Content of acid-soluble Cr(VI) in different sizes of COPR samples
2.3 Physicochemical analysis
The leaching procedures of water-soluble Cr(VI) and acid-soluble Cr(VI) in the untreated and treated COPR samples were performed according to Ref.[19] and Cr(VI) concentration in the leachate was determined according to GB/T 15555.5—1995. The elemental composition except total Cr and Cr(VI) of the untreated COPR samples was measured according to GB 5085.3—2007. Step-scanned XRD data were collected by a D8 (German Bruker) computer-automated diffractometer using Bragg-Brentano geometry.
3 Results and discussion
3.1 Leaching kinetics
The leaching of acid-soluble Cr(VI) from COPR with HF acid is a typical liquid-solid heterogeneous reaction. Taking CAC as an example, the reaction of CAC with HF acid is
Ca4Al2O6(CrO4)·14H2O(s)+14HF(l)=4CaF2(s)+
2AlF3(s)+2AlF3(s)+H2CrO4(l)+20H2O(l) (3)
Simultaneously, the side reactions of HF acid with calcite and periclase are
CaCO3(s)+2HF(l)=CaF2(s)+CO2(g)+H2O(l) (4)
MgO(s)+2HF(s)=MgF2(s)+H2O(l) (5)
There is a solid product to form in each reaction above. According to the scanning electron microscopy (SEM) images of the COPR samples before and after being treated by HF acid (Fig.2), the particle size of COPR is hardly changed, when the acid-soluble Cr(VI) leaching efficiency arrives at 94.61%. Therefore, the leaching process of COPR can be described by the shrinking core model if the shape of COPR particles is nearly regarded as a spherical type. The kinetic model can be determined by one of the following rate-limiting steps: outer diffusion controlled, inner diffusion controlled, chemical reaction controlled and combination controlled step of inner diffusion and chemical reaction [20-21], and the rate-limiting step of the leaching process of the acid-soluble Cr(VI) in COPR further depends on kinetic data.
Fig.2 SEM photographs of COPR samples before (a) and after (b) being treated by HF acid
3.2 Effect of stirring rate
The stirring rate (N) has little influence on the leaching efficiency of acid-soluble Cr(VI) in COPR (Fig.3). When the leaching time (t) is 60 min, Cr(VI) leaching efficiency with a stirring rate of 800 r/min is only 1.07% higher than that with a stirring rate of 600 r/min. This suggests that the leaching process of acid-soluble Cr(VI) is not controlled by the external diffusion [22]. Therefore, all the subsequent experiments were carried out at 600 r/min in order to assure the invariance of this parameter.
Fig.3 Effect of stirring rate on acid-soluble Cr(VI) leaching efficiency (T=298.15 K; L/S=40; c=6 mol/L; d0=0.105-0.125 mm)
3.3 Effect of temperature
As seen in Fig.4(a), the leaching efficiency of acid-soluble Cr(VI) is slightly affected by the leaching temperature. As temperature increases from 298.15 K to 358.15 K, the leaching efficiency of acid-soluble Cr(VI) increases only by 10.16%, which indicates that the leaching process of acid-soluble Cr(VI) from COPR seems not to be controlled by the chemical reaction [21].
In order to obtain the kinetic equation and apparent activation energy (Ea), the experimental data in Fig.4(a) are correlated to various kinetic models for liquid-solid reactions above. As shown in Fig.4(b), the inner-diffusion controlled kinetic equation is found to fit the data best [23]:
(6)
where t is the leaching time (min), c is the HF acid concentration of HF solution (mol/L), d0 is the initial particle size of COPR (mm), V is the solution volume (mL), σ is the molar density of reaction system (mol/m3), De is the diffusion coefficient in porous product layer (m2/s), and Ka is the apparent rate constant (min-1).
Fig.4 Effect of leaching temperature on acid-soluble Cr(VI) leaching efficiency (a), plot of leaching kinetics at different temperatures (b) and plot of ln Ka against 1/T (c) (L/S=40; c= 6 mol/L; d0=0.105-0.125 mm; N=600 r/min)
This suggests that the leaching of acid-soluble Cr(VI) from COPR is controlled by the inner diffusion.
The apparent activation energy was determined based on Arrhenius equation:
(7)
where K0 is the frequency factor (min-1), Ea is the apparent activation energy (kJ/mol), R is the ideal gas constant (8.314 J·mol-1·K-1), and T is the leaching temperature (K).
The plot of ln Ka versus 1/T is linear, as shown in Fig.4(c) and the apparent activation energy and frequency factor (K0) calculated are 8.696 kJ/mol and 6.59×10-2 min-1, respectively. The energy value falls in the range of 4-12 kJ/mol for a typical inner diffusion controlled process, further confirming that the leaching process is inner diffusion controlled [24-25].
3.4 Effect of HF acid concentration
As seen in Fig.5(a), the leaching efficiency of acid-soluble Cr(VI) increases with increasing the HF concentration. However, as HF acid concentration increases from 5 mol/L to 9 mol/L, the leaching efficiency of acid-soluble Cr(VI) with leaching time of 60 min increases only by 6.16%, which indicates that a solid product layer forms during the leaching process, preventing the mobilization of the acid-soluble Cr(VI) from COPR. The kinetic data in Fig.5(a) are also well fitted with Eq.(6) and the results shown in Fig.5(b) also demonstrate that the leaching process is controlled by diffusion through the product layer.
The plot of ln Ka versus ln c is shown in Fig.5(c) and the reaction order of HF acid concentration is calculated to be 0.493 8, indicating a slight dependency of the leaching of acid-soluble Cr(VI) on HF acid concentration.
3.5 Effect of particle size
The particle size of COPR plays an important role in the leaching of acid-soluble Cr(VI) in COPR (Fig.6(a)). As the initial particle size of COPR decreases from 0.180-0.224 mm to 0.080-0.098 mm, the leaching efficiency of acid-soluble Cr(VI) with leaching time of 60 min increases by more than 40%. The kinetic data in Fig.6(a) are also fitted well with Eq.(6) and the results are given in Fig.6(b). The apparent rate constant (Ka) is related to the inverse square of initial particle diameter of COPR (Fig.6(c)), further supporting the conclusion that the leaching process is controlled by diffusion through the product layer. According to ln Ka-ln d0 curve plotted in Fig.6(d), the reaction order with respect to initial particle size of COPR is -2.013 3, which further proves that initial particle size is the remarkable factor influencing the leaching efficiency of acid-soluble Cr(VI) in COPR. So, it is very necessary to take measures to decrease the particle size of COPR for a desirable leaching efficiency of acid-soluble Cr(VI) in the leaching process.
Fig.5 Effect of HF acid concentration on acid-soluble Cr(VI) leaching efficiency (a), plot of leaching kinetics at different HF acid concentrations (b) and plot of ln Ka against ln c (c) (T=298.15 K; L/S=40; d0=0.105-0.125 mm; N=600 r/min)
Fig.6 Effect of initial particle size on acid-soluble Cr(VI) leaching efficiency (a), plot of leaching kinetics at different particle sizes (b), plot of Ka against (c) and plot of ln Ka against ln d0 (d) (T=298.15 K; L/S=40; c=6 mol/L; N=600 r/min)
3.6 Effect of leaching time
As seen in Fig.3, Fig.4(a), Fig.5(a) and Fig.6(a), the effect of leaching time on the leaching efficiency of acid-soluble Cr(VI) is the second only behind that of the initial particle size. It can be seen that the leaching kinetics is fast in the initial period, and slows down in the remaining period, because the solid product layer gradually becomes thicker with time. So, it is necessary to keep leaching time enough or take measures to thin the solid product layer to obtain a desirable leaching efficiency of acid-soluble Cr(VI) in the leaching process.
3.7 Leaching kinetic equation
The apparent rate constant (Ka) in Eq.(6) is related with the reaction system, and is a function of parameters such as reaction temperature, concentration of reagents and particle size. So, Ka can be expressed as [26-27]
(8)
where ci is the bulk fluid concentration (mol/L), α, β and γ are the reaction orders of variable c1, c2 and d0, respectively.
Thus, the leaching of acid-soluble Cr(VI) from COPR with HF solution can be presented by
(9)
3.8 XRD analysis of treated COPR
Fig.7 shows the XRD patterns of the treated COPR with HF. It can be seen that the diffraction peaks of Cr(VI)-bearing minerals, especially the last completely destabilized mineral, brownmillerite, in COPR are not observed. On the other hand, some new phases, such as fluorite and sellaite, are identified, which indicates that HF solution can efficiently destabilize the Cr(VI)-bearing minerals, resulting in mobilizing Cr(VI) in COPR to the leachate.
Fig.7 XRD pattern of treated COPR with HF solution (T= 298.15 K; L/S=40; c=6 mol/L; t=60 min; d0=0.080-0.098 mm; N=600 r/min)
Although all the Cr(VI)-bearing minerals are completely destabilized during the leaching process with HF solution (Fig.7), the acid-soluble Cr(VI) content in the leached COPR still reaches 5.24% of that in the untreated COPR. This Cr(VI) may be absorbed by the amorphous substances [10] or encapsulated by the solid product layer. Therefore, some measures should be taken to remove the rest of acid-soluble Cr(VI) in the treated COPR.
4 Conclusions
1) HF acid solution can effectively destabilize Cr(VI)-bearing minerals and mobilize acid-soluble Cr(VI) from COPR to the solution.
2) The particle size of COPR significantly influences the leaching of acid-soluble Cr(VI), followed by the leaching time, whereas the influence of HF concentration and leaching temperature is slight and the effect of stirring rate is negligible.
3) The results indicate that the shrinking core model is applicable for the leaching process of COPR with HF acid, and the leaching reaction is controlled by the diffusion through the product layer.
References
[1] KOWALSKI Z, GOLLINGER-TARAJKO M. Environmental evaluation of different variants of the chromium compound production model using chromic waste [J]. Waste Management, 2003, 23(8): 771-783.
[2] DEAKIN D, WEST L J, STEWART D I, YARDLEYD B W. Leaching behavior of a chromium smelter waste heap [J]. Waste Management, 2001, 21(3): 265-270.
[3] JAGUPILLA S C, MOON D H, WAZNEA M, CHRISTODOULATOS C, KIM M G. Effects of particle size and acid addition on the remediation of chromite ore processing residue using ferrous sulfate [J]. Journal of Hazardous Materials, 2009, 168(1): 121-128.
[4] JI Zhu. Two key points for the treatment of chromium ore processing residue [J]. Inorganic Chemicals Industry, 2004, 36(5): 1-4. (in Chinese)
[5] GEELHOED J S, MEEUSSEN J C L, HILLIER S, LUMSDON D G, TH0MAS R P, FARMER J G, PATERSON E. Identification and geochemical modeling of processes controlling leaching of Cr(VI) and other major elements from chromite ore processing residue [J]. Geochimica et Cosmochimica Acta, 2002, 66(22): 3927-3942.
[6] HILLIER S, ROE M J, GEELHOED J S, FRASER A R, FARMER J G, PATERSON E. Role of quantitative mineralogical analysis in the investigation sites contaminated by chromite ore processing residue [J]. Science of the Total Environment, 2003, 308(1): 195-210.
[7] HILLIER S, LUMSDON D G, BRYDSON R, PATERSON E. Hydrogarnet: A host phase for Cr(VI) in chromite ore processing residue (COPR) and other high pH wastes [J]. Environmental Science and Technology, 2007, 41(6): 1921-1927.
[8] TERRY P A. Characterization of Cr ion exchange with hydrotalcite [J]. Chemosphere, 2004, 57(7): 541-546.
[9] FARMER J. PATERSON E, BEWLEY R J F, GEELHOED J S, HILLIER S, MEEUSSEN J C, LUMSDON D G, THOAMS R P, GRAHAM M C. The implications of integrated assessment and modeling studies for the future remediation of chromite ore processing residue disposal sites [J]. Science of the Total Environment, 2006, 360(1): 90-97.
[10] TINJUM J M, BENSON C H, EDIL T B. Mobilization of Cr(VI) from chromite ore processing residue through acid treatment [J]. Science of the Total Environment, 2008, 391(1): 13-25.
[11] DERMATAS D, CHRYSOCHOOU M, MOON D H, GRUBB D G, WAZNE M, CHRISTODOULATOS C. Ettringite-induced heave in chromite ore processing residue (COPR) upon ferrous sulfate treatment [J]. Environmental Science and Technology, 2006, 40(18): 5786-5792.
[12] MOON D H, DERMATAS D, WAZNE M, SANCHEZ A M, CHRYSOCHOOU M, GRUBB D G. Swelling related to ettringite crystal formation in chromite ore processing residue [J]. Environmental Geochemistry and Health, 2007, 29(4): 289-294.
[13] MOON D H, WAZNE M, DERMATAS D, CHRISTODULATOS C, SANCHEZ A M, GRUBB D G, CHRYSOCHOOU M, KIM M G. Long-term treatment issues with chromite ore processing residue (COPR): Cr6+ reduction and heave [J]. Journal of Hazardous Materials, 2007, 143(3): 629-635.
[14] MOON D H, WAZNE M, JAGUPILLA S C, CHRISTODOULATOS C, KIM M G, KOUTSOSPYROS A. Particle size and pH effects on remediation of chromite ore processing residue using calcium polysulfide (CaS5) [J]. Science of the Total Environment, 2008, 399(1/2/3): 2-10.
[15] WAZNE M, JAGUPILLA S C, MOON D H, CHRISTODOULATOS C, KIM M G. Assessment of calcium polysulfide for the remediation of hexavalent chromium in chromite ore processing residue (COPR) [J]. Journal of Hazardous Materials, 2007, 143(3): 620-628.
[16] WAZNE M, MOON D H, JAGUPILLA S C, CHRISTODOULATOS C, DERMATAS D, CHRYSOCHOOU M. Remediation of chromite ore processing residue using ferrous sulfate and calcium polysulfide [J]. Geoscience Journal, 2007, 11(2): 105-110.
[17] HATZIFOTIS M, WILLIAMS A, MULLER M, PEGG S. Hydrofluoric acid burns [J]. Burns, 2004, 30(2): 156-159.
[18] SP?LER F, FRENTZ M, F?RST M, KURZ H, SCHRAGE N F. Analysis of hydrofluoric acid penetration and decontamination of the eye by means of time-resolved optical coherence tomography [J]. Burns, 2008, 34(4): 549-555.
[19] PAN Jin-fang, FENG Xiao-xi, ZHANG Da-nian. Study the forms of chromium of chromite ore processing residue [J]. Shanghai Environmental Science, 1996, 15(3): 15-17. (in Chinese)
[20] CHI Ru-an, TIAN Jun, GAO Hong, ZHOU Fang, LIU Min, WANG Chun-wen. Kinetics of leaching flavonoids from pueraria lobatu with ethanol [J]. Chinese Journal of Chemical Engineering, 2006, 14(3): 402-406.
[21] FENG Qi-ming, SHAO Yan-hai, OU Le-ming, ZHANG Guo-fan, LU Yi-ping. Kinetics of nickel leaching from roasting-dissolving residue of spent catalyst with sulfuric acid [J]. Journal of Central South University of Technology, 2009, 16(3): 410-415.
[22] SUN Hui-lan, YU Hai-yan, WANG Bo, MIAO Yu, TU Gan-feng, BI Shi-wen. Leaching dynamics of 12CaO·7Al2O3 [J]. The Chinese Journal of Nonferrous Metals, 2008, 18(10): 1920-1925. (in Chinese)
[23] LI Hao-ran, FENG Ya-li, LUO Xiao-bin, WANG Hong-jie, DU Zhu-wei. Leaching kinetics of extraction of vanadium pentoxide from clay mineral [J]. Journal of Central South University: Science and Technology, 2008, 39(6): 1181-1184. (in Chinese)
[24] ABDEL-AAL E A. Kinetics of sulfuric acid leaching of low grade zinc silicate ore [J]. Hydrometallurgy, 2000, 55(3): 247-254.
[25] LOZANO L J, JUAN D. Leaching of vanadium from spent sulfuric acid catalysts [J]. Minerals Engineering, 2001, 14(5): 543-546.
[26] FAN Xing-xiang, PENG Jin-hui, HUANG Meng-yang, ZHANG Shi-min, ZHANG Li-bo, GUO Sheng-hui, YANG Kun-bin. Study on kinetics of oxidative leaching of chalcopyrite in the presence of silver ion [J]. Precious Metals, 2005, 26(3): 15-20. (in Chinese)
[27] MOHAMMAD S S, DAVOOD M, MEHDI O I. Kinetics of sulfuric acid leaching of cadmium from Cd-Ni zinc plant residues [J]. Journal of Hazardous Materials, 2009, 163(2): 880-890.
(Edited by YANG Bing)
Foundation item: Project(2009FJ1009) supported by Major Program of Hunan Provincial Science and Technology, China; Project(2005CB6237) supported by the National Basic Research Program of China
Received date: 2010-03-10; Accepted date: 2010-07-01
Corresponding author: ZHOU Qiu-sheng, Associate Professor, PhD; Tel: +86-731-88830453; E-mail: qszhou@mail.csu.edu.cn, csqszhou@163.com
- Leaching kinetics of acid-soluble Cr(VI) fromchromite ore processing residue with hydrofluoric acid
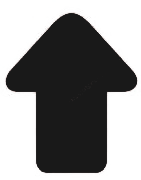