Trans. Nonferrous Met. Soc. China 24(2014) 1674-1682
Cooling slope casting to produce EN AW 6082 forging stock for manufacture of suspension components
Yucel BIROL1, Seracettin AKDI2
1. Department of Metallurgical and Materials Engineering, Dokuz Eylul University, Buca, Izmir, Turkey
2. R&D Centre, AYD Steering and Suspension Parts, Selcuklu, Konya, Turkey
Received 25 October 2013; accepted 7 January 2014
Abstract: The potential of cooling slope casting process to produce EN AW 6082 forging stock for the manufacture of EN AW 6082 suspension components was investigated. EN AW 6082 billets cast over a cooling plate offer a fine uniform structure that can be forged even without a separate homogenization treatment. This is made it possible by the limited superheat of the melt at the start of casting and the fractional solidification that occurs already on the cooling plate. Suspension parts forged from cast and homogenized billets with or without Cr all showed a uniform structure, and the hardness reached HV 110 after the standard artificial ageing treatment.
Key words: aluminium alloys; forging; cooling slope casting; heat treatment
1 Introduction
Forging is an attractive forming process to produce high-precision near-net shape parts with excellent surface qualities with a minimum number of finishing operations [1]. The ever increasing need for light weight solutions in the transportation industry provides aluminium forgings a good prospect since any reduction of the unsprung masses offers significant additional advantages [1]. In addition to light weight, aluminium forgings provide superior structural rigidity, higher strength and fatigue resistance as compared to cast and machined parts. High-per formance aluminium parts such as control arms and steering columns have thus become standard in most passenger cars.
The most extensively used aluminium forging alloy is the age-hardenable EN AW 6082 because of its excellent combination of mechanical properties and corrosion resistance [2]. The EN AW 6082 forging stock is conventionally produced with the extrusion of direct chill cast ingots as this appears to be the most convenient way to reduce the diameter of the ingot to forging stock dimensions. Friction and high shear strains in the contact zone between the billet and the extrusion die lead to small recrystallised surface grains that tend to grow abnormally when exposed to high temperatures [3-6]. This is a major concern since 6082 forgings are almost always submitted to a solution heat treatment before ageing. Such a heterogeneous section with coarse grains on the surface not only impairs the surface quality but also degrades the service performance of the forging.
There has been some interest recently to replace the extruded forging stock with cast billets of the same diameter since the latter not only avoids coarse grained sections but also reduces the production costs by 15%-20% as compared to the extruded bars [7-12]. However, cast stock is not available in the market in diameters below 100 mm. Hence, alternating simple methods to produce cast forging stock may be of interest. One potential method is the cooling slope (CS) casting process that has been widely investigated in recent years to produce feedstock for semi-solid processing [13,14]. CS casting provides uniform equiaxed grains, with much less resistance to plastic flow [15,16]. The objective of this inves tigation is to explore the potential of CS casting process to produce 6082 forging stock in the manufacture of EN AW 6082 automotive suspension components.
2 Experimental
The EN AW 6082 alloys with the composition shown in Table 1 were melted in an induction furnace at 750 °C. The melt was then allowed to cool to 700 °C. The CS casting involved pouring the molten alloy over a 50 mm wide and 300 mm long, inclined steel plate into a permanent mould (Fig. 1). The cooling plate was inclined at 45°. The round billet thus obtained had a diameter of 42 mm and a length of 400 mm. The surface of the plate was coated with a thin layer of boron nitride in order to avoid sticking of the molten alloy on the plate surface.
Table 1 Chemical composition of EN AW 6082 billets used in present investigation (mass fraction, %)
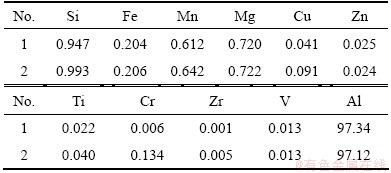
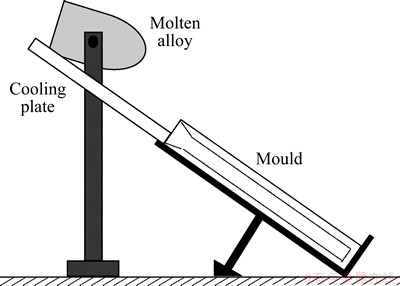
Fig. 1 Schematic illustration of cooling slope casting unit
One set of the cast billets were homogenized at 570 °C for 8 h to dissolve coarse eutectics and Mg2Si particles formed during solidification and to obtain a fine distribution of dispersoids to control recrystallization during processing. They were subsequently cooled to room temperature at an average rate of 400 °C/h to achieve a delicate balance between the precipitation and solute saturation. The cast and homogenized billets were preheated to 500 °C and were forged on a 1600 t forging press into suspension parts as shown in Fig. 2. The forged components were solutionized at 520 °C for 4 h and quenched in water before they were artificially aged at 180 °C for 8 h.
Samples sectioned from the cast and homogenized billets were prepared with standard metallographic techniques: ground with SiC paper, polished with 3 μm diamond paste and finished with colloidal silica. They were examined after etching with a 0.5% HF solution using an Olympus BX51M model optical microscope. The X-ray diffraction (XRD) patterns were recorded with a Shimadzu XRD 6000 diffractometer equipped with Cu Ka radiation. Sections from the cast, extruded, forged and heat treated components were anodized in Barker’s solution, 5 mL HBF4 (48%) in 200 mL water, and then examined with an optical microscope under polarized light. Hardness was measured across these sections with a microhardness tester under a load of 2.94 N and with a dwell time of 20 s.
3 Results and discussion
The microstructure and the grain structure of the CS cast EN AW 6082 billet No. 1 are shown in Figs. 3(a), (b) and (c), respectively. The CS casting has produced fine, equiaxed dendrites. This made it possible by the limited superheat of the melt at the start of casting and the limited amount of solidification that has started already on the cooling plate. The temperature drops below the liquidus temperature when the molten alloy flows over the cooling plate and a(Al) crystals start to nucleate soon after. These crystals are detached from the cooling plate, trapped in the flowing melt and are collected in the mould at the bottom of the cooling plate. When the melt transfer is over, the mould is filled with molten alloy with a very small fraction of a(Al) crystals. Solidification in the permanent mould involves growth of these a(Al) crystals into small equiaxed rosettes.
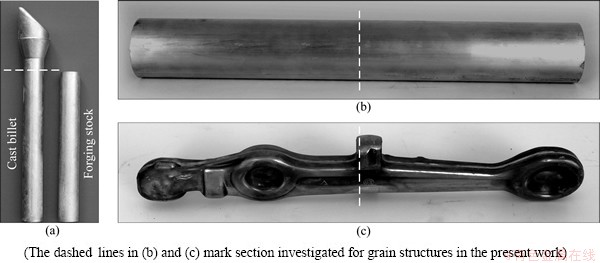
Fig. 2 CS cast billet (a), forging stock (b) and control arm (c) produced from cast and homogenized EN AW 6082 billet
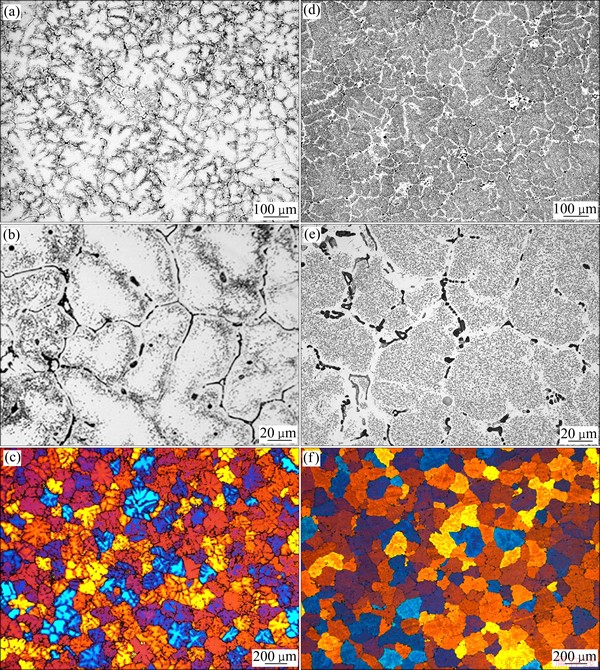
Fig. 3 Microstructures (a, b, d, e) and grain structures (c, f) of cast (a-c) and homogenized (d-f) EN AW 6082 billet without Cr (No.1)
The dendrite boundaries are decorated with plate-like compound particles (Fig. 3(b)) identified with XRD to be cubic a-AlFeMnSi (Fig. 4(a)). The nonuniform distribution of the precipitates across the matrix implies dendritic segregation in the form of coring (Figs. 3(b) and (c)). However, coring is limited owing to an equiaxed dendritic structure smaller than typically encountered in the conventional permanent mould casting processes. The small dark particles near the boundaries are the cubic b-Mg2Si precipitates that form during cooling after solidification (Fig. 3(b)). Precipitate-free zones have formed near the boundaries as much of Si is bound in the interdendritic a-AlFeMnSi particles. The grain structure is fine with an average diameter of (117±19) μm, and is uniform across the entire section (Fig. 3(c)). The cast stock is inferred from an average hardness of HV (57.6±1.1) to be slightly harder than the fully soft state. This is attributed to the solid solution hardening owing to the solutionizing of a considerable fraction of Mg and Si in the aluminium matrix. The uniform hardness profile across the section of the cast billet implies further that the CS-cast forging stock is largely strain-free (Fig. 5(a)).
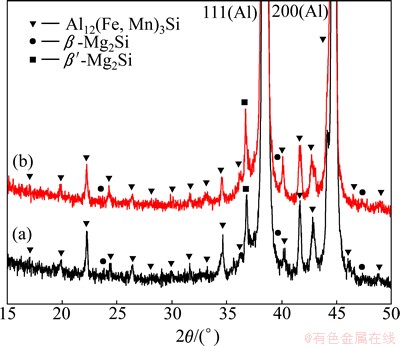
Fig. 4 XRD spectra of cast (a) and homogenized (b) EN AW 6082 billet without Cr (Alloy No.1)
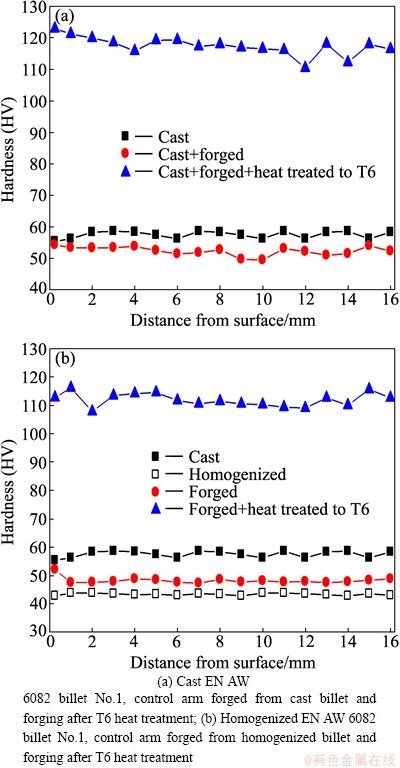
Fig. 5 Hardness measurements across section
Microstructural changes after the homogenization treatment are typical of 6082 alloys exposed to high temperatures (Figs. 3(d)-(f)). The interdendritic network of the AlFeMnSi compound is replaced by stringers of discrete spherical particles during soaking at 570 °C [3]. The latter were identified by XRD analysis to be a-AlFeMnSi particles, suggesting that there has been no transformation of the interdendritic phase at high temperatures (Fig. 4(b)). This is attributed to the Mn element in the present alloy which is known to stabilize the cubic a phase [17]. The coring is removed as evidenced by a uniform distribution of Mg2Si precipitates across the matrix grains (Fig. 3(e)). Solutionizing during soaking has apparently improved the post-soaking precipitation capacity. With an average hardness of HV (43.5±0.4), the homogenized billets are relatively softer than the cast billets in spite of a copious precipitation across the section (Fig. 5(b)). It is thus fair to claim that the precipitates formed during post soaking cooling are not of the hardening variety. The hardness drop is best accounted for by the relaxation of the partial supersaturation of the aluminium matrix with Mg and Si.
The cast structure of the EN AW 6082 billet with 0.13% Cr consists of equiaxed a(Al) dendrites with plate-like cubic a compound particles at the dendrite boundaries and Mg2Si precipitates near the boundaries (Figs. 6(a) and (b)). The interdendritic particles were found by EDS analysis to contain, in addition to Fe, Mn and Si, considerable Cr as expected and are thus identified as ac-Al12(Fe,Mn,Cr)3Si (Fig. 7). The cast grain size is relatively smaller, (78±13) μm. The ac particles are very effective in pinning grain boundaries (Fig. 6(c)). The population of b-Mg2Si precipitates in the homogenized samples is also higher in the 6082 alloy with Cr than its counterpart without Cr, owing to the propensity of the ac dispersoids in the former (Figs. 6(d) and (e)). The latter serve as favourable sites for b-Mg2Si nucleation and thus encourage precipitation activities. The hardness profiles across the section of the cast billet with Cr are very similar to those measured in the 6082 without Cr (Fig. 8(a)). The average hardness values of the 6082 alloy with Cr are HV (56.8±1.4) and HV (46.5± 0.7), in the as-cast state and after homogenization, respectively, evidencing softening upon homogenization (Fig. 8(b)).
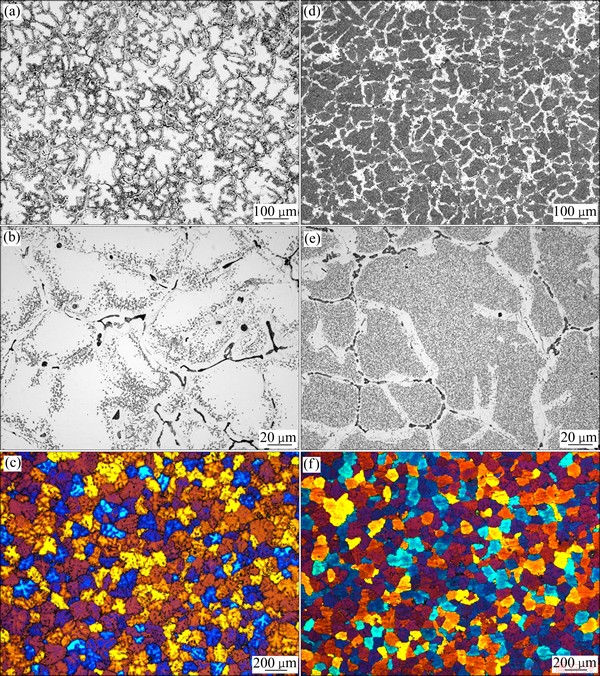
Fig. 6 Microstructures (a, b, d, e) and grain structures (c, f) of cast (a-c) and homogenized (d-f) EN AW 6082 billets with Cr (No.2)
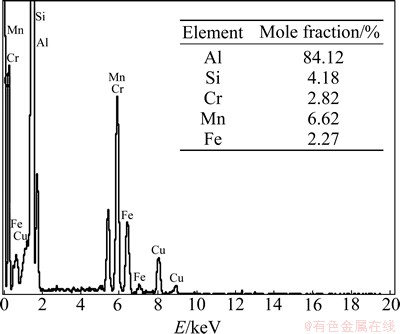
Fig. 7 Typical EDS spectrum and analysis of ac particles in cast EN AW 6082 billet with Cr (No.2)
The as-cast and homogenized EN AW 6082 billets were pre-heated to 500 °C and forged into the suspension component shown in Fig. 2(c). The grain structures across the sections of the suspension components forged from the cast as well as the homogenized billets are shown in Fig. 9. The plastic strain introduced by forging can be estimated fairly accurately from the change in grain shape across the section of the forged component. The grains are nearly spherical at the surface of the forged component. The frictional conditions are believed to be responsible for the relatively limited plastic flow near the surface. Conditions in the hot forming processes with aluminium alloys are often regarded as sticking friction due to the high pressure and temperature involved [18]. Aluminium is fixed to the surface of the tool in this particular state of friction and deformation takes place only in the interior of the billet. The shape factor of the grains increases as we move from the surface to the centre of the section where the maximum shape factor is estimated to be approximately 6. Considering that the grains are equiaxed before and elongated after forging, the effective plastic strain that leads to a nearly 6-fold increase in the length of the grains is estimated to be approximately 1. This is largely consistent with the strain distribution obtained from the finite element analysis.
While the alignment of the matrix grains near the centre evidences substantial plastic flow during the forging operation, there is hardly any increase in the hardness across the section of the forgings produced from cast and homogenized stock with or without Cr (Figs. 5 and 8). Lack of strain hardening across the section suggests that any deformation hardening introduced during hot forging is recovered via dynamic restoration processes.
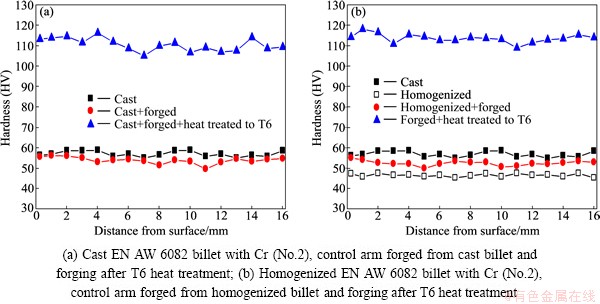
Fig. 8 Hardness measurements across section
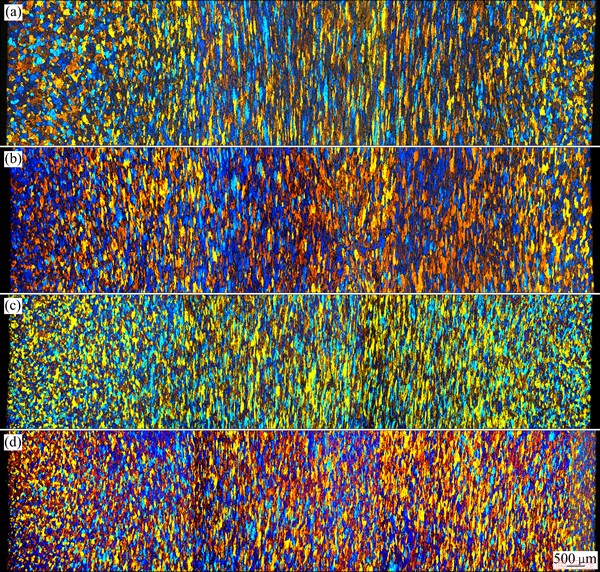
Fig. 9 Grain structures across section of control arm forged from cast (a) and homogenized (b) EN AW 6082 billet without Cr (No.1) and from cast (c) and homogenized (d) EN AW 6082 billet with Cr (No.2)
The grain structures of the components forged from the cast and the homogenized billets are largely retained after the solution heat treatment (Fig. 10). Coarse surface grains, the predominant feature on the surface of the component forged from the extruded EN AW 6082 stock [12], are not encountered (Fig. 11). The very small grains on the surface of the extruded stock are very susceptible to grow at high temperatures and undergo grain growth during the solution heat treatment. Such coarse surface grains impair the surface quality, but the boundary between the coarse surface and much smaller interior grains is weak and thus threatens the performance of suspension parts under dynamic loads. It is fair to conclude from Fig. 11 that the response of the CS cast billet to the forging process is substantially different from that of the extruded stock, but is nearly identical to the direct chill (DC) cast counterpart.
The strain energy retained in the suspension component forged from CS cast billet apparently does not suffice to kick of a recrystallization process during the solution heat treatment. There is no evidence for abnormal grain growth either, due to the strain-free state of the component after forging and to the relatively big grains that are much less susceptible to grain growth. Cr, which makes a very favourable impact on the section grain structures of the forging when the extruded material is forged under exactly the same conditions, does not appear to be essential when CS cast stock is employed. The very fine and uniform equiaxed structure of the cast billet produced with the CS casting process also reduces the impact of the homogenization treatment to a minimum. Suspension parts forged from cast and homogenized billets with and without Cr all age harden to over HV 110 after the standard artificial ageing treatment (Figs. 5 and 8).
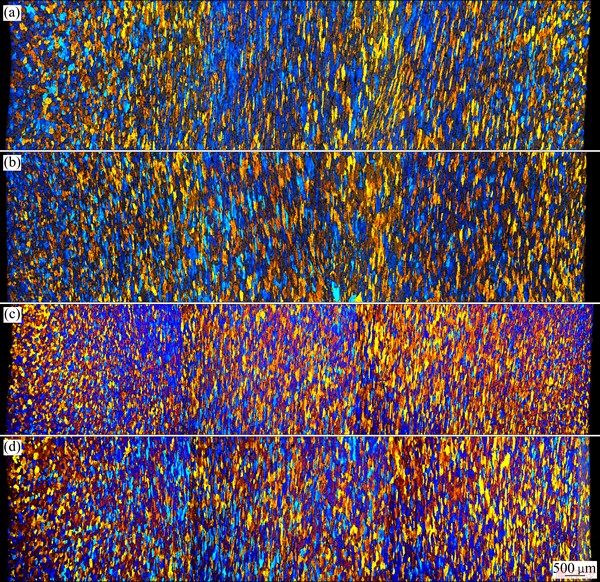
Fig. 10 Grain structures across section of control arm forged from cast (a) and homogenized (b) EN AW 6082 billet without Cr (No.1) and from cast (c) and homogenized (d) 6082 billet with Cr (No.2), after solution heat treatment
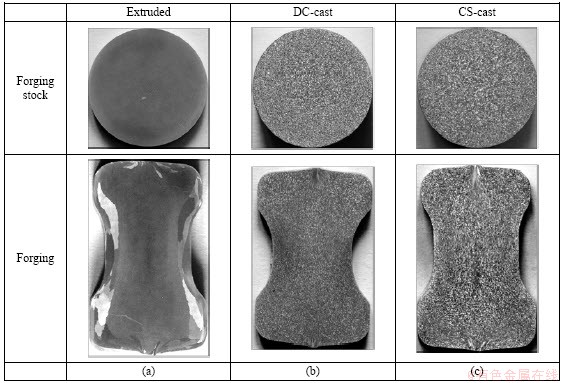
Fig. 11 Grain structures across sections of round bars produced by extrusion (a), DC casting (b) and CS casting (c) and forgings produced from these bars
4 Conclusions
Cooling slope casting offers features that are very attractive in the EN AW 6082 billets to be employed as forging stock for the manufacture of suspension components. CS cast billets enjoy a very fine uniform structure that can be forged even without a separate homogenization treatment, thanks to the limited superheat of the melt at the start of casting and the fractional solidification that occurs already on the cooling plate. The response of such a billet to the forging process is profoundly different from that of the extruded stock which almost always suffers coarse grains at the surface of the forging. The addition of Cr, which makes a very favourable impact on the section grain structures of the forging when the extruded material is forged under exactly the same conditions, does not appear to be essential when CS cast stock is employed. Suspension parts forged from cast and homogenized billets with and without Cr all age harden to over HV 110 after the standard artificial ageing treatment.
Acknowledgements
It is a pleasure to thank Mr. F. ALAGEYIK from MRC and Mr. Onur ILGAZ from AYD for their help with the experimental part of the work.
References
[1] KUHLMAN G W. ASM handbook: Forging of aluminum alloys [M]. Vol. 14, 9th ed. ASM, 1988, 244.
[2] DAVIS J R. ASM specialty handbook: Aluminum and aluminum alloys [M]. Materials Park, OH: ASM International, 1996.
[3] PARSON N, BARKER S, SHALANSKI A, JOWETT C. Control of grain structure in Al-Mg-Si extrusions [C]// Proc 8th Int Aluminum Extrusion Technology Seminar. Orlando 1, 2004: 11-22.
[4] van GEERTRUYDEN W H, BROWNE H M, MISIOLEK W Z, WANG P T. Evolution of surface recrystallization during indirect extrusion of 6xxx aluminum alloys [J]. Metal Mater Trans A, 2005, 36: 1049-1056.
[5] SWEET E D, CARAHER S K, DANILOVA N V, ZHANG X. Effects of extrusion parameters on coarse grain surface layer in 6xxx series extrusions [C]//Proc 8th Int Aluminum Extrusion Technology Seminar. Wauconda, IL, USA, 2004: 115-126.
[6] BIROL Y. The effect of processing and Mn content on the T5 and T6 properties of AA6082 profiles [J]. J Mater Proc Tech, 2006, 173: 84-91.
[7] JENSRUD O, PEDERSEN K, SYVERTSEN F. Automotive sector: The castforge potentiality [J]. Alluminio & Leghe, 2011, 5: 69-74.
[8] DAVIS J L, ROCZYN H G, BRUSKI R. Advanced continuous casting for direct-forged aluminium parts [C]// Proc European Metallurgical Conference. EMC, Dresden, Germany, 2005: 727-760.
[9] KIM H R, SEO M G, BAE W B. A study of the manufacturing of tie-rod ends with casting/forging process [J]. J Mater Proc Tech, 2002, 125-126: 471-476.
[10] PLONKA B, KLYSZEWSKI A, SENDERSKI J, LECH GREGA M. Application of Al alloys in the form of cast billet as stock material for the die forging in automotive industry [J]. Arc Civil Mech Eng, 2008, 8: 149-156.
[11] ANDERSON M, BRUSKI GROSZKIEWICZ R D, WAGSTAFF B. Aluminum cast house technology [C]//Light Metals 2001. TMS, 2001: 185.
[12] BIROL Y, ILGAZ O. Effect of cast and extruded stock on the grain structure of EN AW 6082 alloy forgings [J]. Mater Sci Tech, 2014, 30: 860-866.
[13] HAGA T, NAKAMURA R, TAGO R, WATARI H. Effects of casting factors of cooling slope on semisolid condition [J]. Transactions of Nonferrous Metals Society of China, 2010, 20: s968-s972.
[14] ZHAO Z Y, GUAN R G, WANG X, LI Y, DONG L, LEE C S, LIU C M. Microstructure formation mechanism and properties of AZ61 alloy processed by melt treatment with vibrating cooling slope and semisolid rolling [J]. Metals and Materials International, 2013, 19: 1063-1067.
[15] BIROL Y. A357 thixoforming feedstock produced by cooling slope casting [J]. J Mater Proc Tech, 2007, 186: 94-101.
[16] BIROL Y. Cooling slope casting and thixoforming of hypereutectic A390 alloy [J]. J Mater Proc Tech, 2008, 207: 200-203.
[17] MONDOLFO L F. Aluminum alloys: Structure and properties [M]. Butterworths, London, 1976.
[18] SAHA P. Aluminum extrusion technology [M]. Materials Park, OH: ASM International, 2000.
采用冷却倾斜板铸造方法生产悬架零部件用EN AW 6082铝合金锻坯
Yucel BIROL1, Seracettin AKDI2
1. Materials Institute, Marmara Research Centre, TUBITAK, Gebze, Kocaeli, Turkey;
2. R&D Centre, AYD Steering and Suspension Parts, Selcuklu, Konya, Turkey
摘 要:对采用冷却倾斜板铸造方法生产EN AW 6082铝合金锻坯用于制备悬架零部件的可行性进行研究。采用冷却倾斜板铸造方法生产的EN AW 6082铝合金坯料具有细小的均匀组织,可直接用于锻造生产而不需要另外的均匀化处理。这可能是因为铸造开始时采用较小的过热和冷却倾斜板上发生较多的凝固所致。对采用冷却倾斜板铸造和均匀化处理的含或者不含Cr的EN AW 6082铝合金坯料,通过锻造生产的悬架零部件均具有均匀的组织,经过标准的人工时效处理后硬度可达到HV110。
关键词:铝合金;锻造;冷却倾斜板铸造;热处理
(Edited by Sai-qian YUAN)
Corresponding author: Yucel BIROL; E-mail: yucel.birol@deu.edu.tr
DOI: 10.1016/S1003-6326(14)63240-4