J. Cent. South Univ. (2017) 24: 1275-1280
DOI: 10.1007/s11771-017-3532-7

WO3 nanofibers on ACF by electrospun for photo-degradation of phenol solution
TONG Hai-xia(童海霞)1, 2, TIAN Xiong(田雄)1, 2, WU Dao-xin(吴道新)1, 2,
WANG Cheng-feng(王成峰)1, 2, ZHANG Qiao-li(张巧丽)1, 2, JIANG Zhao-hui(蒋朝辉)1, 2
1. Chemical and Biologic Engineering Institute, Changsha University of Science and Technology, Changsha 410114, China;
2. Hunan Provincial Key Laboratory of Materials Protection for Electric Power and Transportation,
Changsha University of Science and Technology, Changsha 410114, China
Central South University Press and Springer-Verlag Berlin Heidelberg 2017
Abstract: Electrospun WO3 nanofibers were fabricated by coaxial electrospinning and directly annealing WCl6/polyvinylpyrrolidone (PVP) nanofibers on activated carbon fibres (ACF), and characterized by scanning electron microscopy (SEM) and X-ray diffractometry (XRD). The most suitable condition for electrospinning is the mass ratio of WCl6 to PVP 0.6, appropriate amount DMF and ethanol, a voltage of 28 kV, the reception distance of 15 cm, the humidity range within 10% and 20% and the moving rate of the pump 0.001 mm/s. The photocatalytic activities of WO3 nanofibers were evaluated by the photo-degradation of phenol solution under the irradiation of 500 W xenon lamp. The results showed that, the sizes of the fibers are about 100 nm, and after being photodegraded for 210 min, the concentration of phenol decreased from 20.05 mg/L to 8.60 mg/L. Thus, the photo-degradation rate of WO3 nanofibers for phenol solution is 2.87 mg/(L·h).
Key words: WO3; nanofibers; electrospinning; photo-degradation; phenol solution
1 Introduction
The utilization of photocatalytic semiconductors to decompose organic pollutants under the illumination of solar light has been considered as one of the popular approaches to address the increasing global concern of environmental remediation [1, 2]. Titanium dioxide (TiO2) has been the most studied photocatalytic material so far [2]. However, in view of the more substantial utilization of the visible light spectrum in solar light than that of TiO2, tungsten trioxide (WO3) can be one of the most suitable alternatives due to its smaller band gap (about 2.4-2.8 eV) relative to TiO2 (about 3.2 eV) [1-3], stable physicochemical properties and strong resilience to photo-corrosion effects [1].
WO3 is a kind of n-type metal oxide semiconductor. And to date, the applications of WO3 are very wide, such as electrochromics,photochromics, gasochromics etc [4-6]. And as well, WO3 acts as a photocatalyst for photo-splitting water, solar cell and photocatalytic or photoelectrocatalytic degradating organic pollutants [7-9].
Since photocatalytic reactions mainly take place on the surface of catalysts, a high surface-to-volume ratio is of great significance for increasing the decomposition rate of organic pollutions. Compared with nanopowder and thin films, nanofibers have higher surface-to-volume ratio, even which are expected to solve the problems of the poor recuperability and reutilization limitation for nanopowder and low contact area for thin films. Electrospinning is a mature method to produce continuous fibers with diameters ranging from tens to hundreds of nanometers [10-12], and some papers have reported the preparation of WO3 nanofibers or their composites by electrospinning for the application in photodegradating methyl orange, xanthate and rhodamine-B [13-15].
In this work, the WO3 nanofibers are prepared by a novel and simple approach—coaxial electrospining combined with a sol-gel process using WCl6 as the material, and the photocatalytic activity was explored by the degradation of phenol. The preparation of metals doped WO3 nanofibers are under way.
2 Experimental
2.1 Materials and instruments
WCl6 (Belgium, ACROE, excellent level of pure), polyvinylpyrrolidone (PVP, 360000, Missouri, Sigma- Aldrich, AR), Anhydrous ethanol (AR, Reagent Factory of Hunan Normal University), N, N-dimethylformamide (DMF AR, Hunan Huihong Reagent Co., Ltd.); phenol (AR, Chongqing East Sichuan Chemical Company Limited); ACF (Jiangsu Kejing Carbon Fiber Co., Ltd.) were used.
1003-type electrostatic spinning equipment (Tianjin Dongwen High-pressure Power Plant), Rigaku TTRⅢ- type X-ray diffraction instrument (operating voltage 20-40 kV, operating current 10-300 mA), JSM-5600LV- type scanning electric microscope, CEL-HXF300-type xenon lamp parallel light source (Beijing Zhong Jiao Jin Yuan Technology Co., 50 W, 320-2500 nm), and 6890 N-type gas chromatograph (Agilent Co.) were used.
2.2 Preparation of electrospun WO3 nanofibers
The precursors of WO3 with different WCl6/PVP mass ratios of 0.43, 0.5, 0.6, 0.7, 1.0 were prepared respectively as follows: 1.000 g PVP as the template was dissolved in the mixture of 5 mL ethanol and appropriate amount DMF, which was titled solution A; A certain mass of WCl6 was dissolved in appropriate amount ethanol followed by vigorous stirring at the room temperature for several hours, which was titled solution B.Solution A and solution B were mixed evenly. The precursor solution of the electrospun WO3 nanofibers was obtained. Subsequently, the mixture was ejected with a stainless steel capillary with a voltage of 28 kV. The distance between the capillary and collector was 15 cm. The ACF which was washed with ultra-pure water and dried at 105 °C for 3 h was used as a collector. The humidity range was set within 10%-20%. The moving rate of the pump is 0.001 mm/s. The electrospun WCl6/PVP fibers with ACF were annealed in the muffle furnace to obtain WO3 nanofibers. The calcining process is shown in Fig. 1 [16].
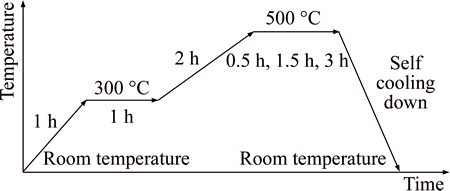
Fig. 1 Temperature-controlled process of calcination
2.3 Characterization of nanofibers
X-ray diffraction (XRD) was used to check the coexistence of different crystal phases on a Rigaku TTRⅢ-type X-ray diffraction instrument (operating voltage 20-40 kV, operating current 10-300 mA). The diffractograms were recorded with Cu Ka1 radiation (l=0.154056 nm)over a 2θ range from 10° to 90°. A plumbaginous counter with monochromator was used. The scanning electric microscope (SEM) was used to investigate the surface morphology of the electrospun WO3 precursor and WO3 nanofibers. The SEM images were performed on a JSM-5600LV-type scanning electric microscope.
2.4 Photo-activity tests
The photocatalytic oxidation activities of the as-prepared samples were evaluated by the degradation of phenol in aqueous solution under the irradiation of CEL-HXF300-type xenon lamp parallel light source with the output power of 50 W. And the parameters of CEL-HXF300-type xenon lamp parallel light source are given in Table 1. The reacting temperature was controlled at (25±2) °C.
Table 1 Parameters of CEL-HXF300-type xenon lamp parallel light source
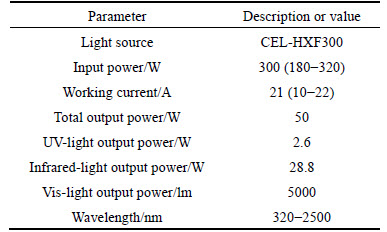
The photo-catalytic reaction was performed with an outside irradiation reactor. A mixture of catalyst (about 10 mg) on the surface of ACF (the size of 0.7 cm×1.5 cm), phenol solution (50 mL, with an initial concentration of 20 mg/L) were put into the reactor (70 mL). The concentration of phenol was measured by gas chromatograph.
The blank experiment and the dark absorption test for the photocatalysts were carried out.
3 Results and discussions
3.1 SEM analysis of catalysts
3.1.1 Influence of WCl6/PVP mass ratio on surface morphology of electrospun WO3 precursor
The SEM images of the precursor of WO3 with different WCl6/PVP mass ratios of 0.43, 0.5, 0.6 and 0.7 are shown in Figs. 2(a), (b), (c) and (d), respectively. From Fig. 2, when the mass ratio is 0.43, the fiber-like structures of the precursor of WO3 are not clear; when the ratio is up to 0.5, the fiber-like structures are clearer than those of 0.43. However, the fibers stick together, and there are no clear and bright fiber structures. When the ratio is up to 0.6, the fiber-like structures are clear and bright, and the sizes are several nanometers. When the ration is up to 0.7, the fiber-like structures disappear, and the flocculent porous structures are obtained. When the ratio is up to 1.0, the mixture cannot form into fibers from the capillary. Thus, the optimum WCl6/PVP mass ratio is 0.6.
3.1.2 Influence of standing time on surface morphology of WO3 precursor
The SEM images of the electrospun WO3 precursor with the standing time of 0 h and 8 h are shown in Figs. 3(a) and (b), respectively. From Fig. 3(a), the fiber- like structures of the electrospun WO3 precursor are clear, and the sizes are more than 100 nm. When the standing time is 8 h, the fiber-like structures collapse, and the possible reason is that WCl6 is sensitive to moisture.
3.1.3 Influence of calcination project on surface morphology of electrospinning WO3 precursor
Figures 4(a), (b) and (c) show the SEM images of electrospun WO3 calcined at 500 °C for 0.5 h, 1.5 h and 3 h, respectively. From Fig. 4(a), when the thermostatical time is 0.5 h, the morphology of fibers with a few nodules can be seen, the diameter of which is more than 100 nm. As shown in Fig. 4(b), when the thermostatical time is 1.5 h, the nodules disappear, and the fibers are only obtained. From Fig. 4(c), when the thermostatical time is 3 h, the fibers are destroyed, and powder is obtained. The particles aggregate whose sizes are more than 200 nm. Thus, the optimum thermostatical time is 1.5 h, which ensures sufficient crystallinity and the fibers’formation without significant grain growth. When the thermostatical time is 0.5 h, the WO3 fibers may not satisfy the requirement; however,a longer thermostatical time may lead to the fibers fracture and the crystal grain growth or agglomeration.
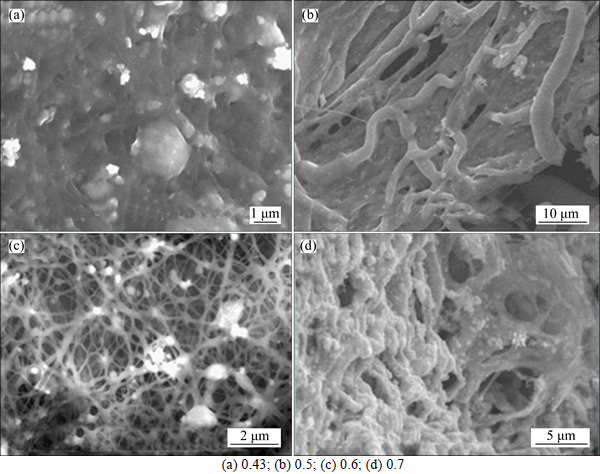
Fig. 2 SEM images of electrospun WO3 precursor with different WCl6/PVP mass ratios:
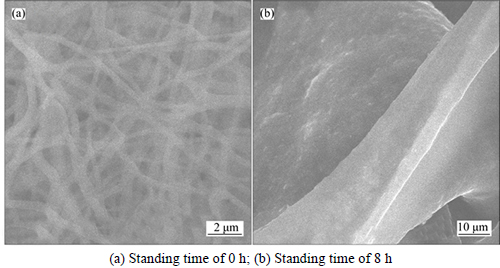
Fig. 3 SEM images of precursor of WO3 nanofibers:
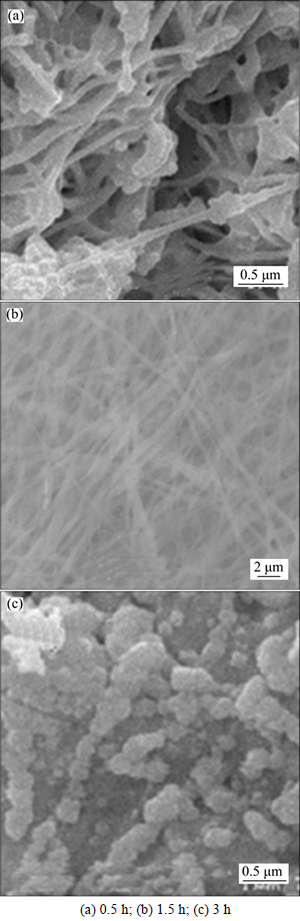
Fig. 4 SEM images of WO3 calcined for different time:
3.2 XRD analysis of WO3 nanofibers
Figure 5 shows the XRD pattern of WO3 nanofibers calcined for 1.5 h. From the pattern, some distinct peaks of WO3 can be observed, and all of the diffraction peaks coincide with the standard card file (JCPDS 05-0363). In the XRD pattern of WO3 nanofibers, the reflections at 22.8° (110), 33.6°(111), 41.5°(300) and 54.7°(202) are well-indexed with WO3, and they own good crystal growth and stable structure.
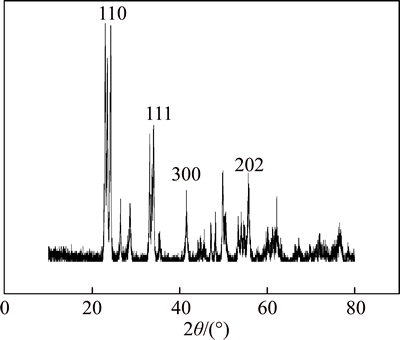
Fig. 5 XRD pattern of WO3 nanofibers
3.3 Influence of calcination on ACF and WO3 nanofibers
Figure 6 shows the pictures of the precursor of WO3 nanofibers loaded on ACF and WO3 nanofibers loaded on ACF after being calcined at 500 °C. From Fig. 6(a), the precursor of WO3 nanofibers is well-distributed on the surface of ACF just like a thin layer of gauze. After being calcined at 500 °C for 1.5 h, the areas of the precursor of WO3 nanofibers and the ACF are reduced obviously, and the area reduced ratio of the precursor is larger than that of ACF.However, the WO3 nanofibers and the ACF have not been broken down after being calcined. For the reduction of ACF surface area, there are maybe two reasons: one is the burning of the carbon, and the other is the collapse of the channels in ACF. In order to determine which reason caused the reduction of the area of the ACF after calcination, another experiment was carried out. A piece of ACF was calcined at 500 °C in N2 for 1.5 h, and the area was reduced greatly. The result was shown in Fig. 7.
Figure 7 shows the pictures of ACF and ACF calcined at 500 °C in N2 for 1.5 h. From Fig. 7, it can be concluded that the decrease of the area of ACF mainly results from the collapse of the channels in ACF calcined at high temperature.
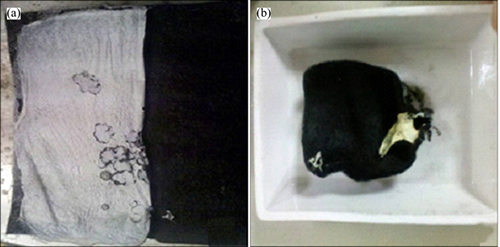
Fig. 6 Pictures of precursor of WO3 nanofibers loaded on ACF (a) and WO3 nanofibers loaded on ACF after being calcined at 500 °C (b)
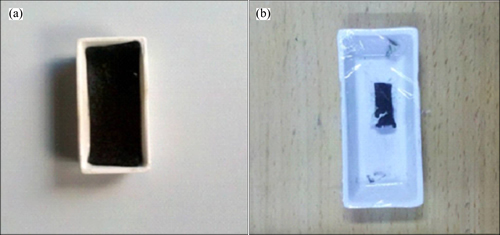
Fig. 7 Pictures of ACF (a) and ACF calcined at 500 °C in N2 for 1.5 h (b)
3.4 Results of photo degradation of phenol
Table 2 gives the concentrations of phenol at different illuminating time, and the photocatalytic degradation performances of phenol in the presence of WO3 nanofibers shown in Fig. 4(b) is presented in Fig. 8. The initial concentration of phenol is 20.05 mg/L, and after being illuminated for 210 min, the concentration of phenol decreases to 8.60 mg/L, then with the time prolonging, it almost does not change. Thus, the rate of photo degradation is 2.87 mg/(L·h). There are many factors affecting the reaction rate of the photocatalytic degradation, such as catalyst, light intensity, concentration of organic pollutes, pH value, temperature, and added oxidant and salt [17]. The ways to improve the reaction efficiency are under way.
Some researchers have noticed that when the band structure of the semiconductor meets the requirement of redox potential of the organics, the light-generated electrons (e), the holes (h+), hydroxyl radicals
and superoxide anion radicals
play important roles respectively in the photocatalytic oxidation process [18, 19], especially the hydroxyl radical
[20]. On the other hand, the surface morphology of the photocatalyst and the adsorption capacity of the supporter also play important roles in the reaction rate of the photocatalytic degradation.
Table 2 Relationship of concentrations of phenol and time of illumination
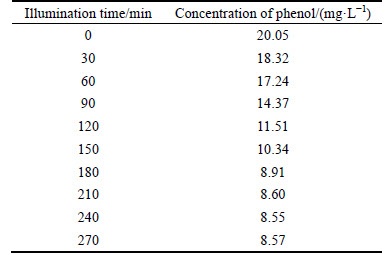
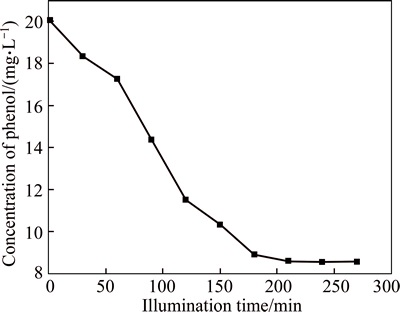
Fig. 8 Phenol decomposition performances for WO3 nanofibers on ACF
4 Conclusions
The electrospun WO3 nanofibers are obtained, and the appropriate mass ratio of WCl6/PVP is 0.6. The electrospun WO3 precursor is not suitable to be exposed in the air because WCl6 is sensitive to moisture. After being calcined at 500 °C for 1.5 h, the surface areas of WO3 nanofibers and ACF are reduced obviously.When the initial concentration of phenol is 20.05 mg/L, the concentration decreases to 8.60 mg/L after it is illuminated for 210 min. Thus, the rate of photo degradation is 2.87 mg/(L·h).
References
[1] ZHENG Hai-dong, OU J Z, STRANO M S, KANER R B, MITCHELL A, KALANTAR-ZADEH K. Nanostructured tungsten oxide-properties, synthesis, and applications [J]. Advanced Functional Materials, 2011, 21(12): 2175-2196.
[2] ZHANG Huan-jun, CHEN Guo-hua, BAHNEMANN D W. Photoelectrocatalytic materials for environmental applications [J]. Journal of Materials Chemistry, 2009, 19(29): 5089-5121.
[3] MORALES W, CASON M, AINA O, de TACCONI N R, RAJESHWAR K. Combustion synthesis and characterization of nanocrystalline WO3 [J]. Journal of the American Chemical Society, 2008, 130(20): 6318-6319.
[4] LEFTHERIOTIS G, KOUBLI E, YIANOULIS P. Combined electrochromic-transparent conducting coatings consisting of noble metal, dielectric and WO3 multilayers [J]. Solar Energy Materials and Solar Cells, 2013, 116: 110-119.
[5] NALIN M, POIRIER G, RIBEIRO S J L, MESSADDEQ Y, CESCATO L. Glasses in the SbPO4-WO3 system [J]. Journal of Non-Crystalline Solids, 2007, 353: 1592-1597.
[6] CHEN Shang-hui, LUO Jian-yi, TAN Hui-dong, CHEN Jian, DENG Shao-zhi, XU Ning-sheng. Study of self-heating phenomenon and its resultant effect on ultrafast gasochromic coloration of Pt-WO3 nanowire films [J]. Sensors and Actuators. B: Chemical, 2012, 173: 824-832.
[7] RONCONI F, SYRGIANNIS Z, BONASERA A, PRATO M, ARGAZZI R, CARAMORI S, CRISTINO V, BIGNOZZI C. Modification of nanocrystalline WO3 with a dicationic perylene bisimide: Applications to molecular level solar water splitting [J]. Journal of the American Chemical Society, 2015, 137(14): 4630-4633.
[8] LI Wen-zhang, JIN Guan-hua, HU Hai-shi, LI Jie, YANG Ya-hui, CHEN Qi-yuan, Phosphotungstic acid and WO3, incorporated TiO2, thin films as novel photoanodes in dye-sensitized solar cells [J]. Electrochimica Acta, 2015, 153: 499-507.
[9] GIRISH K S, KOTESWARA RAO K S R. Tungsten-based nanomaterials (WO3 & Bi2WO6): Modifications related to charge carrier transfer mechanisms and photocatalytic applications [J]. Applied Surface Science, 2015, 355: 939-958.
[10] YANG Yu-guo, LIU Bing, ZHANG Yuan-yuan, LV Xian-shun, LEI Wei, WANG Xu-ping. Fabrication and luminescence of BiPO4: Tb3+/Ce3+ nanofibers by electrospinning [J]. Superlattices & Microstructures, 2015, 90: 227-235.
[11] MERLINI C, PEGORETTI A, ARAUJO T M, RAMOA SILVIA D A S, SCHREINER WIDO H, BARRA Guilherme-mariz de-oliverira. Electrospinning of doped and undoped-polyaniline/poly (vinylidene fluoride) blends [J]. Synthetic Metals, 2016, 213: 34-41.
[12] FORMENTI S, CASTAGNA R,
R, BERTARELLI C, FRANCESCO B V. The relevance of extensional rheology on electrospinning: the polyamide/iron chloride case [J]. European Polymer Journal, 2015, 75: 46-55.
[13] SUI Chun-hong, GONG Jian, CHENG Tie-xin, ZHOU Guang-dong, DONG Shun-fu. Fabrication of tungsten oxide microfibers with photocatalytic activity by electrospinning from PVA/H3PW12O40 gel [J]. Applied Surface Science, 2011, 257(20): 8600-8604.
[14] MATTHEWS J A, WNEK G E, SIMPSON D G, BOWLIN G L. Electrospinning of collagen nanofibers [J]. Biomacromolecules, 2002, 3(2): 232-238.
[15] KATTA P, ALESSANDRO M, RAMSIER R D, CHASE G G. Continuous electrospinning of aligned polymer nanofibers onto a wire drum collector [J]. Nano Letters, 2004, 4(11): 2215-2218.
[16] LENG Ji-yan, XU Xiu-juan, L
Ning, FAN Hui-tao, ZHANG Tong. Synthesis and gas-sensing characteristics of WO3 nanofibers via electrospinning [J]. Journal of Colloid and Interface Science, 2011, 356(1): 54-57.
[17] LIU Rong-long. Study on photocatalytic degradation of aqueous phenol solution with nano TiO2 membrane. [D]. Chongqing: Chongqing University, 2004. (in Chinese)
[18] CHEN Fen, LIU Hong-qi, BAGWASI S, SHEN Xing-xing, ZHANG Jin-long. Photocatalytic study of BiOCl for degradation of organic pollutants under UV irradiation [J]. Journal of Photochemistry and Photobiology. A:Chemistry. 2010, 215: 76-80.
[19] JO Jeong-hueon, ERNEST T, KIM Kwang-joo. Treatment of TNT red water by layer melt crystallization [J]. Journal of Hazardous Materials, 2014, 275: 185-192.
[20] SONG Hai-yan. The preparation and catalytic performance of novel photocatalytic materials [D]. Anhui: University of Science and Technology of China, 2006. (in Chinese)
(Edited by YANG Bing)
Cite this article as: TONG Hai-xia, TIAN Xiong, WU Dao-xin, WANG Cheng-feng, YANG Dao-wu, XIA Jiao-yun. WO3 nanofibers on ACF by electrospun for photo-degradation of phenol solution [J]. Journal of Central South University, 2017, 24(6): 1275-1280. DOI: 10.1007/s11771-017-3532-7.
Foundation item: Project(15B003) supported by the Outlayed-up Youth Project of Hunan Provincial Department of Education, China; Project(KY201623) supported by the Housing and Urban Construction Department of Hunan Province, China; Project(2016CL02) supported by the Hunan Provincial Key Laboratory of Materials Protection for Electric Power and Transportation, China; Project(11405013) supported by the National Natural Science Foundation of China
Received date: 2016-06-25; Accepted date: 2016-12-01
Corresponding author: TONG Hai-xia, Professor, PhD; Tel: +86-731-85258733; Fax: +86-731-85258733; E-mail: tonghaixia@126.com; daoxinwu@ 126.com