
Structure and property of mesoporous molybdenum/carbon
co-doped brookite titania
L? Xiao-meng(吕晓萌)1, LIU Jun(刘 军)2, ZHANG Hui(张 辉)1, DING Jian-lin(丁建林)1, XIE Ji-min(谢吉民)1
1. School of Chemistry and Chemical Engineering, Jiangsu University, Zhenjiang 212013, China;
2. School of Environment, Jiangsu University, Zhenjiang 212013, China
Received 10 August 2009; accepted 15 September 2009
Abstract: Phase-pure brookite TiO2 was prepared by hydrothermal method using Ti(SO4)2 as precursor. From the point of energy compensation, molybdenum/carbon co-doped brookite TiO2 photocatalysts were prepared in the presence of dissolved glucose and ammonium molybdate. The samples were characterized by thermoanalysis, UV-vis diffuse reflectance spectroscopy (UV-vis/DRS), X-ray diffractometry (XRD), nitrogen adsorption and transmission electron microscopy (TEM). The photocatalytic activities of the as-prepared samples were tested on the photodecoloration of rhodamine B (Rh B) under simulated solar-light irradiation. The wavelength of light adsorption of co-doped samples is red shifted and the intensity of light adsorption increases highly in visible-light region compared with the single-doped samples. Thermal stability of brookite phase is improved greatly after co-doping, indicating that the co-doping facilitates the phase stabilization. Co-doping can improve the photodecoloration activity of composite brookite TiO2.
Key words: co-doping; brookite titania; photocatalysis
1 Introduction
Recently, great efforts have been made to modify the band structure of TiO2 to shift the absorption edge toward the visible light region[1-3]. As a result, monodoping with 3D transitional metals or nonmetals has been explored expensively to improve its photocatalytic activity. However, the monodoped systems create partially occupied impurity bands which act as the recombination center and proper synthesis method and parameters must be considered[4-5]. The modification of TiO2 by co-doping was an effective method to improve the photocatalytic activity, especially the co-doping of 3D transitional metals and nonmetals, which had attracted much attention[6-10]. KUBACKA et al[11] synthesized W and N-doped TiO2 anatase-based materials with both unprecedent high activity and selectivity in the gas-phase partial oxidation of aromatic hydrocarbons using sunlight as excitation energy and molecular oxygen as oxidant. Nano-particles of TiO2 co-doped with N and Fe(III) showed greatly-enhanced vis-photocatalytic activities[12]. Based on the first- principles band structure calculations and analysis of the band-edge wave function characteristics, passivated (Mo+C) co-doped TiO2 is proposed as strong candidate for photoelectrochemical(PEC) hydrogen production through water splitting[13], because it reduces the band gap to the ideal visible-light region, but does not affect much the position of conduction band minimum(CBM). These results indicate that co-doped TiO2 with nonmetals and transition metal ions may be a new promising photocatalyst.
In this work, molybdenum and carbon co-doped brookite titania photocatalysts were prepared by hydrothermal synthesis. Structure and photo absorption property of the prepared samples were characterized in detail by combined means of XRD, UV-vis spectroscopy, and DTA. The photocatalytic activity of brookite TiO2 was investigated via bleaching rhodamine B (Rh B) under Xe (1 000 W) lamp irradiation.
2 Experimental
2.1 Nanoparticles synthesis
All chemicals used in this study were of analyticalgrade and used without further purification. Distilled water was used in all experiments. Titanium sulfate was transferred into Teflon-lined autoclaves, which was then sealed into a stainless steel tank for hydrothermal treatment at 180 ℃. A series of hydrothermal time of 2, 12, 24, 36, 48, and 60 h were used. Then, the reactor was cooled to room temperature naturally, and the resulted product was collected and washed with deionized water and dried at 80 ℃ in air. The amount (5%-15%, molar fraction) of glucose or ammonium molybdate was added into 60 mL of deionized water in a 100 mL autoclave for hydrothermal treatment at appropriate temperature to prepare single or co-doped samples.
2.2 Characterization
Purity and crystallization of the samples were characterized by powder X-ray diffraction on a D/max–γA X-ray spectrometer (Rigaku, Japan) at 40 kV and 200 mA with monochromatic Cu Kα (λ=1.5418 ?) radiation. Transmission electron microscopy (TEM) images were taken on a TECNAI12 TEM instrument (Philips). UV-vis diffusion reflectance spectra of the samples were measured by using an UV-2401 (Shimadzu) UV-vis spectrophotometer. The thermo- analysis was conducted on STA 449C thermal analyzer (Netzsch, German).
2.3 Photocatalytic evaluation
A 1 000 W Xe lamp was used in the photocatalytic experiments, which was placed in a cooling trap for maintaining the constant temperature by water circulation. Aqueous solution of Rh B with sample was placed in photochemical reactor and purged with air at a fixed flow rate throughout the experiments. The solutions with samples were equilibrated in dark for 20 min before irradiation. Samples were collected per 30 min and centrifuged for UV-vis analysis.
3 Results and discussion
Fig.1 shows XRD patterns of samples prepared under different hydrothermal conditions. Hydrothermal time greatly influences the structure of samples. Sample for 2 h was partly crystallized and tended to perfect crystallite with the increase of hydrothermal time. Increasing the hydrothermal time produced enhancement in crystallinity of pure brookite, as evidenced by an increase in the sharpness and intensity of reflection at 2θ=32.8?. The appropriate temperature selected in the following experiment was 24 h.
Fig.2 shows XRD patterns of samples prepared with different dopants. Characteristic peaks at 25.3?, 30.7?, 40.1?, 48.0?, 55.2?, and so were observed for all other diffraction patterns in Fig.1 and 2, which are identical to the standard card (JCPDS 12-1360). The prepared samples were of phase pure brookite TiO2 with high crystallinity. Doping has little effect on the structure of samples. No molybdenum oxide peak or other peak can be observed in the XRD pattern. However, doping influences the cell parameters of TiO2. The grain sizes and lattice parameters for the samples are listed in Table 1. It is clear that lattice parameters of co-doped sample have changed and become much more different from those of single-doped sample.
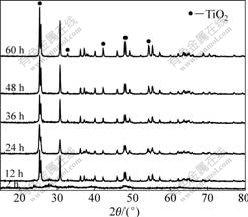
Fig.1 XRD pattern of samples prepared under different hydrothermal conditions
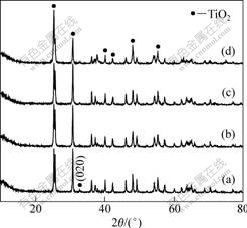
Fig.2 XRD patterns of samples: (a) Blank; (b) 5%Mo; (c) 5%C; (d) 5%(Mo+C)
Table 1 Physical property of samples with 5% dosage
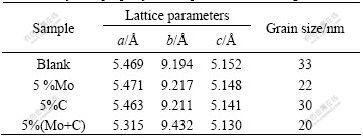
The crystal sizes estimated from the (120) peaks show that the crystallites are 33, 22, 30, and 20 nm for undoped TiO2, Mo-doped (5%) brookite TiO2, C-doped (5%) brookite TiO2 and co-doped (5%) brookite TiO2, respectively.
The UV-vis diffusion reflectance spectra (UV-vis/DRS) of the samples are shown in Fig.3. Strong absorption in the ultraviolet region ascribed to the band-band transition can be observed clearly for all the samples. The absorption edge of doped sample moved toward longer wavelength in comparison with that of blank sample, indicating that the band gap was narrowed after doping. However, different dopant and dosage influence the intensity of absorption in visible light region, i.e., the greater the amount of dosage, the higher
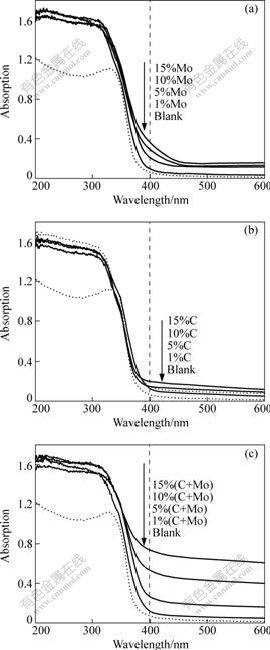
Fig.3 UV-vis spectra of samples: (a) Mo-doping; (b) C-doping; (c) (C+Mo)-doping
the intensity of visible light absorption (Figs.3(a) and (b)). Effect of molybdenum as dopant on light absorption was greater than carbon. In addition, it has been proven that, when the titanium ions in the TiO2 lattice were substituted by carbon, no visible-light response was observed[4]. Thus, we believe that the substitution of O ions in the TiO2 lattice by C atoms leads to visible light absorption.
Intensity of co-doping was more obvious than single-doping, leading to higher absorption intensity in visible light region but no change in UV light region (Fig.3(c)). The reason may be that C-doping degrades the band gap of TiO2, but Mo-doping maintains the charge equilibrium of system. The synergistic effect of co-doping favors the electron transfer between valence band and conductive band, which leads to higher intensity of visible light absorption.
The N2 adsorption-desorption isotherms and pore size distribution curves of the resulted photocatalyst samples are shown in Fig.4 and Fig.5. The pore size distribution was calculated from desorption branch of
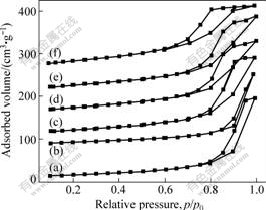
Fig.4 Absorption-desorption isothermal curves of samples: (a) 5%C; (b) 15%C; (c) 5%Mo; (d) 5%(Mo+C); (e) 15%Mo; (f) 15%(Mo+C)
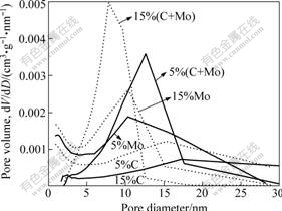
Fig.5 Pore size distribution of samples
nitrogen isotherms by Barret-Joyner-Halenda (BJH) method using the Halsey equation. In Fig.4, the physioadsorption isotherms can be classified as type Ⅳ with hysteresis loops in the IUPAC classification, which was characteristic of mesoporous materials. The Brunauer-Emmett-Teller (BET) specific surface areas of C-doped (5%) TiO2, C-doped (15%)TiO2, Mo-doped (5%) TiO2,Mo-doped (15%) TiO2,co-doped (5%) TiO2 and co-doped (15%) TiO2 were 60.3, 48.3, 79.2, 93.2, 88.0 and 119.4 m2/g, respectively. The higher crystallinity can reduce bulk defects, while the high surface area can probably facilitate the mass transfer of reactants or reaction intermediates. Therefore, it is expected that the high surface area and high crystallinity of these products can enhance the photocatalytic activity. Obviously, in the nitrogen adsorption-desorption isothermal curves, there is a hysteresis loop at 0.6<p/p0<1 in the isotherms of the samples, corresponding to filling of mesopores produced by the agglomeration of primary particles in the resulted samples. The pore size distributions of the resulted samples showed considerable difference (Fig.5). The distribution of average pore size varied from 7.8 to 17.4 nm.
Fig.6 shows the TEM image of the co-doped (5%) sample. The image shows that sample consists of small particulate with a size of around 20 nm, which agrees with the XRD results calculated by the Scherrer equation.
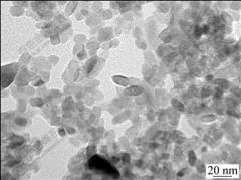
Fig.6 TEM image of co-doping (5%) sample
From the above analysis, we know that by hydrothermal heat treatment at 180 ℃ for 24 h, the obtained samples were brookite TiO2, which was reported to be less thermally stable than rutile TiO2[14-15]. However, doped samples are comparatively thermally stable at higher temperature. The transformation of brookite to rutile, an exothermic process with high activation energy, begins at 619 ℃ for undoped sample, 860 ℃ for 5% co-doped sample and 934 ℃ for 15% co-doped sample (Fig.7). From the DTA curves, the most important information is that the co-doping induces the phase stabilization.
The photocatalytic activity of brookite samples was investigated by determining the remaining concentration of Rh B. Fig.8 shows the photodecoloration ratio of Rh B (10 mg/L) under Xe lamp irradiation for different samples. Prior to the simulated solar-light irradiation, the adsorption equilibrium between the Rh B solution and photocatalysts had been reached. When the lamp was switched, the photodecoloration reaction of Rh B was initiated. It was found that the photolysis of Rh B without photocatalysts was negligible under simulated solar light irradiation. For C-doping samples, there was optimum doping concentration. The photo-decoloration rate increased up to 95 % for C-doped (10%) sample and then decreased with C dosage increasing. The reason may be that carbon has two less valence electrons than oxygen, and the substitution of C on the O site acts as a double acceptor. Hence, the carbon may act as hole-electron center with increasing C dosage. For Mo-doped samples, the photodecoloration ratio decreased with increasing Mo dosage and achieved 90% for Mo-doped (15%) sample. Since the 4d orbital energy is close to that of Ti 3d, when Ti is replaced by Mo, the defect levels are resonant with the conduction band.
It is known that the photocatalytic process mainly takes place on the surface of catalysts and involves comprehensive competing reactions. Inhibiting the
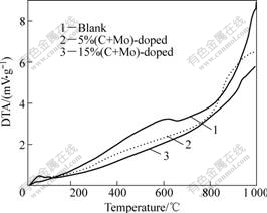
Fig.7 DTA curve of different samples
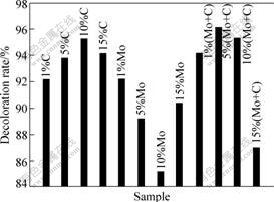
Fig.8 Comparison of photocatalytic decoloration activity
undesirable electron-hole pair recombination is important to enhance the photocatalytic activity because it can improve the ability to produce hydroxyl radical group ·OH, which is possibly beneficial for oxidation of Rh B. As seen from Fig.8, the optimum dosage of co-doped samples is higher than that of single-doped samples. This is because Mo introduces only a shallow donor level below the CBM, and the coupling of C makes its energy higher.
4 Conclusions
1) Phase-pure and structural stable mesoporous nano crystalline (Mo+C)-doped brookite TiO2 have been obtained through a direct solution-phase using Ti(SO4)2 as precursor. The samples revealed that the absorption in visible light region of co-doped samples increased obviously and absorption intensity was enhanced highly, which facilitated light harvesting.
2) The photocatalytic activities of the samples were tested on the decoloration of Rh B under simulated solar-light irradiation. The passivated (Mo+C)-doped brookite TiO2 was proved to be a strong candidate for decoloration organic pollutants in visible-light region.
References
[1] AMBRUS Z, BAL?ZS N, ALAPI T, WITTMAN G, SIPOS P, DOMBI A, MOGYOR?SI K. Synthesis, structure and photocatalytic properties of Fe(Ⅲ)-doped TiO2 prepared from TiCl3 [J]. Appl Catal B: Environ, 2008, 81(1/2): 27-37.
[2] YIN S, KOMATSU M, ZHANG Q W, SAITO F, SATO T. Synthesis of visible-light responsive nitrogen/carbon doped titania photocatalyst by mechanochemical doping [J]. J Mater Sci, 2007, 42(7): 2399-2404.
[3] GU D E, YANG B C, HU Y D. V and N co-doped nanocrystal anatase TiO2 photocatalysts with enhanced photocatalytic activity under visible light irradiation [J]. Catal Commun, 2008, 9(6): 1427-1476.
[4] HUANG Y, HO W K, LEE S C, ZHANG L Z, LI G S, YU J C. Effect of carbon doping on the mesoporous structure of nanocrystalline titanium dioxide and its solar-light-driven photocatalytic degradation of NOx [J]. Langmuir, 2008, 24(7): 3510-3516.
[5] ZHENG R Y, LIN L, XIE J L, ZHU Y X, XIE Y C. State of doped phosphorus and its influence on the physicochemical and photocatalytic properties of P-doped titania [J]. J Phys Chem C, 2008, 112(39): 15502-15509.
[6] SHEN X Z, LIU Z C, XIE S M, GUO J. Degradation of nitrobenzene using titania photocatalyst co-doped with nitrogen and cerium under visible light illumination [J]. J Hazard Mater, 2009, 162(2/3): 1193-1198.
[7] YANG X, XU L L, YU X D, GUO Y H. One-step preparation of silver and indium oxide co-doped TiO2 photocatalyst for the degradation of rhodamine B [J]. Catal Commun, 2008, 9(6): 1224-1229.
[8] SRINIVASAN S S, WADE J, STEFANAKOS E K, GOSWAMI Y. Synergistic effects of sulfation and co-doping on the visible light photocatalysis of TiO2 [J]. J Alloys Compd, 2006, 424(1/2): 322-326.
[9] CONG Y, ZHANG J L, CHEN F, ANPO M, HE D N. Preparation, photocatalytic activity, and mechanism of nano-TiO2 co-doped with nitrogen and iron (Ⅲ) [J]. J Phys Chem C, 2007, 111(28): 10618-10623.
[10] LING Q C, SUN J Z, ZHOU Q Y. Preparation and characterization of visible-light-driven titania photocatalyst co-doped with boron and nitrogen [J]. Appl Surf Sci, 2008, 254(10): 3236-3241.
[11] KUBACKA A, BACHILLER-BAEZA B, COL?N G, FEM?NDEZ-GARC?A. W, N-Codoped TiO2-anatase: A sunlight-operated catalyst for efficient and selective aromatic hydrocarbons photo-oxidation [J]. J Phys Chem C, 2009, 113(20): 8553-8555.
[12] HAO H Y, ZHANG J L. The study of iron (Ⅲ) and nitrogen co-doped mesoporous TiO2 photocatalysts: Synthesis, characterization and activity [J]. Microporous Mesoporous Mater, 2009, 121(1/3): 52-57.
[13] GAI Y Q, LI J B, LI S S, XIA J B, WEI S H. Design of narrow-gap TiO2: A passivated codoping approach for enhanced photoelectrochemical acivity [J]. Phys Rev Lett, 2009, 102: 036402.
[14] GATESHKI M, YIN S, REN Y, PETKOV V. Titania polymorphs by soft chemistry: Is there a common structural pattern? [J]. Chem Mater, 2007, 19(10): 2512-2518.
[15] YIN S, YAMAKI H, KOMATSU M, ZHANG Q W, WANG J S, TANG Q, SAITO F, SATO T. Preparation of nitrogen-doped titania with high visible light induced photocatalytic activity by mechanochemical reaction of titania and hexamethyleneteramine [J]. J Mater Chem, 2003, 13(12): 2996-3001.
(Edited by YANG Hua)
Foundation item: Project(SH2009003) supported by Social Development Foundation of Zhejiang Province, China; Project supported by Special Foundation for Senior Talents of Jiangsu University, China; Project supported by Jiangsu Enviroumental Materials and Environmental Engineering Key Laboratory Jiangsu Province, China
Corresponding author: L? Xiao-meng; Tel: +86-511-88791708; Fax: +86-511-88791800; E-mail: laiyangmeng@163.com