
Rapid manufacture of Ni-based superalloy components
by three-dimensional printing
LIU Jian-xin(刘建新), H. A. KUHN
ProMetal Division, The Ex One Company, 8075 Pennsylvania Avenue, Irwin, PA 15642, USA
Received 28 July 2006; accepted 15 September 2006
Abstract: The challenge to rapid manufacturing high performance metal components is how to consolidate the uncompressed powder preforms to full or near full density without shape distortion. A new approach developed by ProMetal was proposed, in which a bimodal powder, typically a coarse prealloyed powder blended with a fine metal powder was used to build green preforms by three-dimensional printing and then sintered at a temperature above the solidus temperature of the coarse prealloyed powder and below the melting temperature of the fine powder particles or the solidus temperature if the fine powder is a prealloyed powder as well. This approach was successfully applied to sinter Ni-based superalloy 718 preforms, which were built through three dimensional printing into near full density.
Key words: Ni-based superalloy; rapid manufacture; three-dimensional printing
1 Introduction
During the past two decades, rapid prototyping developed into a successful technology which allows construction of geometries that are impossible to form by conventional processes. However, the ultimate challenge from rapid prototyping to rapid manufacturing is in the materials aspect which is perhaps the least developed area of the technology [1]. That is, even though the shapemaking technology is unlimited in terms of geometric complexity, the printed shapes must be thermally processed to develop useful properties.
Three-dimensional printing (3DP) is one of solid free-form fabrication technologies, which is very similar to an ink-jet printer. Instead of ink, a binder ‘glue’ is printed into the powder layer following a computer pattern that is obtained by applying a slicing algorithm to the CAD solid model. The result of 3DP processing is a ‘green’ perform, consisting of about 60%(volume fraction) metal powder, about 10%(volume fraction) binder to hold metal powder together, and the remainder is pore space. The green preform is somewhat fragile and must undergo a thermal process to achieve desirable mechanical properties and density.
Because the porosity level of a green perform is much higher than that of green compacts made by conventional powder consolidation methods, a liquid phase must be involved in the thermal process to attain high density. The traditional liquid phase sintering relies on liquid formation in mixed elemental or prealloyed powders by melting one of the components, or by the formation of a eutectic phase[2]. A variant to this is supersolidus liquid phase sintering, in which prealloyed powders are heated to temperatures between the solidus and the liquidus to attain a semi-solid structure [3-4].
Supersolidus liquid phase sintering is an attractive approach for processing prealloyed powders. Densifica- tion is normally rapid; even coarse powders show rapid densification since they fragment into individual grains [5]. However, densification depends on the liquid content, which, in turn, depends on the sintering temperature. If the sintering temperature is too low, the liquid quantity is insufficient for the desired densification. On the other hand, excess liquid forms if the sintering temperature is too high. This results in the formation of an intergranular network of deleterious brittle phases, exaggerated grain growth, pore coalescence, swelling, distortion, and slumping [6]. From process control considerations, a small increase in liquid fraction with an increase in temperature is desired. However, in practice such sintering systems are rare. Therefore, the widespread use of supersolidus liquid phase sintering is limited since the conditions for densification are often close to those resulting in shape distortion [5-8].
The present work relates to a new approach developed by ProMetal [9], in which a bimodal powder, typically a coarse prealloyed powder blended with a fine metal powder is used to build green preforms by 3DP and then sintered at a temperature above the solidus temperature of the coarse prealloyed powder and below the melting temperature of the fine powder particles or the solidus temperature if the fine powder is a prealloyed powder as well. A bimodal powder blended with a coarse powder and a fine powder gives improved packing density[2,10]. During heating, the fine powder undergoes solid phase sintering since the fine powder has high sinterability[11]. When the sintering temperature is reached, the coarse prealloyed powder undergoes supersolidus liquid-phase sintering, reduces the rigidity of the system, and rapid densification occurs in response to the sintering stress. Because solid sintering and supersolidus liquid phase sintering occur in sequence, we refer to this approach as solid-supersolidus liquid phase sintering. In solid-supersolidus liquid phase sintering, only the grains in the coarse prealloyed powder are disintegrated by forming liquid along the grain boundaries; then the liquid squeezed out wets in- terparticle contacts and penetrates into the small particles in the voids between the coarse prealloyed particles. Fig.1 illustrates the differences in densification mech- anisms between the supersolidus liquid phase sintering and solid-supersolidus liquid phase sintering.
As an example, in this study, the solid-supersolidus liquid phase sintering approach was used to sinter nickel-based superalloy 718 components built via three dimensional printing process.
2 Experimental
The prealloyed powder used for the test was 718 master alloy powder manufactured by carpenter powder products, Bridgeville, PA. Each element in the master alloy powders was 1.25 times of the composition of the standard Inconel 718 alloy. Table 1 lists the designed compositions of prealloyed 718 master alloy powder.
Because of the non-equilibrium nature of the prealloyed powder it is difficult to accurately predict the melting behavior using phase diagrams. Hence, a simultaneous thermal analysis was employed to determine the solidus and liquidus temperatures. The measurement was carried out on NETZSCH STA 409C simultaneous thermal analyzer with a heating rate of 10 ℃/min under vacuum. The differential scanning calorimetric curve of the master alloy powder has an endotherm with an onset temperature of 1 205.7 ℃ and an offset temperature of 1 230.8 ℃, respectively, the former corresponds to the solidus temperature of 718 master alloy powder. The standard Inconel 718 alloy has a melting range of 1 260-1 336 ℃ and a density of 8.22 g/cm3.
The fine powder was carbonyl nickel powder (CNP). The carbonyl nickel powder (UT-3PM Grade) was provided by Chemalloy Company, Bryn Mawr, PA, with an average particle size of 8.4 μm.
The prealloyed powder was screened to two particle size ranges for initial test. One powder has a particle size range between 45 μm and 90 μm and another is less than 45 μm. The former is encoded as 718 MA (coarse) and the latter is 718 MA (fine). Those two different particle sized 718 master alloy powders were blended with 20%(mass fraction)CNP. 718 MA (fine)+ CNP indicates a powder mixture of 80%718 MA (fine) powder and 20% CNP, and 718 MA (coarse)+CNP includes 80% 718 MA (coarse) powder and 20% CNP. The powders were weighed on a laboratory balance and blended in a Turbula blender for 30 min. The final compositions of both powder mixtures are similar to those of Inconel 718 alloy.
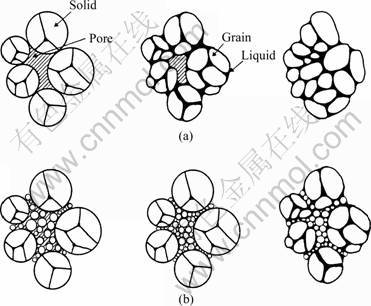
Fig.1 Illustration of difference between supersolidus liquid phase sintering(a) and solid-supersolidus liquid phase sintering(b)
Table 1 Chemical composition of prealloyed powders(mass fraction, %)

The two blended powders were poured into a cylindrical brass container and tapped (5 strikes) to give uniform packing and weighed on a laboratory balance with accuracy of 0.01 g for apparent density me- asurement. The 718 MA (coarse) powder (single modal) encoded as 718 MA (coarse) was measured for comparison. The apparent density was calculated from the mass of powder filling a brass container divided by the volume of the container, which has a standard volume of 25 cm3 and mass of 103.78 g. The apparent density of 718 MA (fine)+CNP is 3.89 g/cm3, that of 718 MA (coarse)+CNP is 4.26 g/cm3, and that of 718 MA (coarse) is 3.55 g/cm3. Blended powder packs to higher densities than single modal powder.
The powders were then poured into cylindrical crucibles (2.54 cm inner diameter), respectively and tapped (5 strikes) to let them settle down. Sintering was carried out at the temperatures from 1 100 to 1 285 ℃ for 60 min under vacuum. The sintered densities were measured by the Archimedes method. For comparison, the same procedure was performed on 718 MA (coarse) powder.
3 results and discussion
Figs.2-4 show the sintering shrinkages, sintered densities, and fractional densities vary with sintering temperature, respectively. Note that the shrinkage data are not very accurate since shrinkage is strongly affected by the initial packing density of the powder in the crucible. Therefore, the explanation below is based on Figs.3 and 4.
Below 1 150 ℃, the blended powders have a higher density because carbonyl nickel powder significantly impacts the sintering response at lower temperatures. Even though a low temperature sintering test was not performed, it is believed that the solid phase sintering of carbonyl nickel powder starts at a temperature below 800 ℃ [12]. 718 MA (fine)+CNP powder and 718 MA (coarse)+CNP powder sintered at 1 100 ℃ for 60 min attain densities of 4.56 g/cm3 and 4.53 g/cm3, respec- tively, while 718 MA (coarse) powder , a powder without the blended carbonyl nickel powder, did not sinter up to temperature of 1 150 ℃. The 718 MA (coarse) powder preforms sintered at 1 100 and 1 150 ℃ can be pulverized easily by hand.
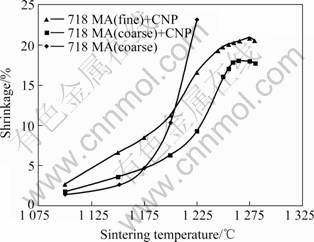
Fig.2 Shrinkages vs sintering temperature
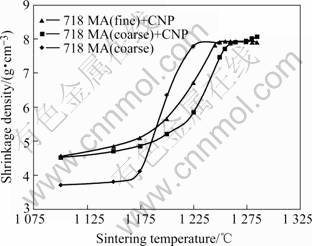
Fig.3 Sintered densities vs sintering temperature
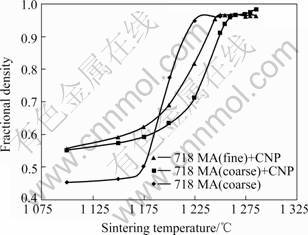
Fig.4 Fractional sintered densities vs sintering temperature
At a temperature between 1 150 and 1 175 ℃, close to the solidus temperature of 718 MA powder, all three powders begin rapid densification because a small amount of liquid is formed. Once liquid forms, 718 MA (coarse) powder densifies faster than the blended powder. At 1 200 ℃, the density of 718 MA (coarse) powder is higher than that of the blended powders because 718 MA (coarse) powder has more liquid fraction. Fig.5 shows the optical microstructures of 718 MA (coarse) powder sintered at 1 225 ℃ for 60 min. It is found that a eutectic phase forms along the grain boundaries.
Fig.6 shows the optical microstructures of 718 MA (fine)+CNP powder sintered at 1 250 and 1 280 ℃ for 60 min and Fig.7 shows the optical microstructures of 718 MA (coarse)+CNP powder sintered at 1 255 and 1 280 ℃ for 60 min, respectively. After sintering, the fine CNP particles are no longer distinct because of grain growth. The sintered 718 MA (fine)+CNP powder has smaller grain size than 718 MA (coarse)+CNP powder. The porosity is uniform and seems to be low. Grain boundaries are clean except a slight eutectic phase forms in the microstructure of 718 MA (coarse)+CNP powder.
Sintering a green preform with a density of 3.89 g/cm3 to full density, 8.22 g/cm3, requires a sintering shrinkage as high as 22.1%, for a preform with a green density of 4.26 g/cm3 the sintering shrinkage is up to 19.7%, and with a green density of 3.55 g/cm3 the sintering shrinkage attains to 24.4%. In practice, no powder attains theoretical shrinkage for sintering to full density before distortion. The highest shrinkage for 718 MA (fine)+CNP powder is 21%, for 718 MA (coarse)+ CNP powder is 18%, and for 718 MA (coarse) powder is less than 22%. Correspondingly, the highest sintered density is 7.95 g/cm3 or fractional density of 0.97 for both blended powders and less than 7.78 g/cm3 or fractional density of 0.95 for 718 MA (coarse) powder. The lowest temperatures at which the highest sintered densities are obtained are 1 250 ℃ for 718 MA (fine)+ CNP powder and 1 260 ℃ for 718 MA (coarse)+CNP powder. The distortion onset temperature for both blend- ed powders is 1 280 ℃, as shown in Fig.4. Therefore, the sintering window for 718 MA (fine)+CNP powder is 30 ℃ and for 718 MA (coarse)+CNP powder is 20 ℃. The sharp densification and quick distortion indicate that 718 MA (coarse) powder has a narrow sintering window or the sintering window may not exist (distortion occurs at a temperature below 1 225 ℃ since the powder sintered at this temperature was distorted), as shown in Fig.4.
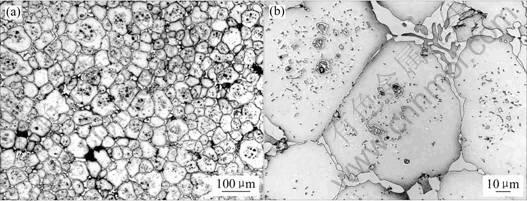
Fig.5 Optical microstructures of 718 MA (coarse) powder sintered at 1 225 ℃ for 60 min: (a) Lower magnification; (b) Higher magnification
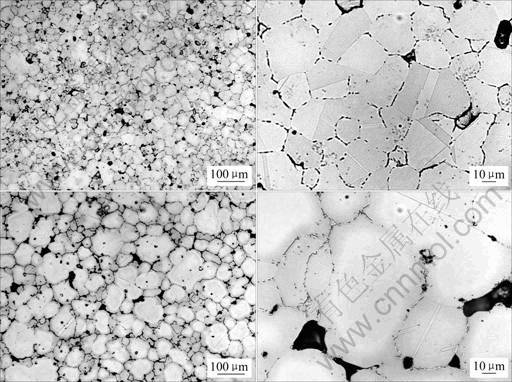
Fig.6 Optical microstructures of 718 MA (fine)+CNP powder sintered at different temperatures for 60 min: (a), (b) 1 250 ℃; (c), (d) 1 280 ℃
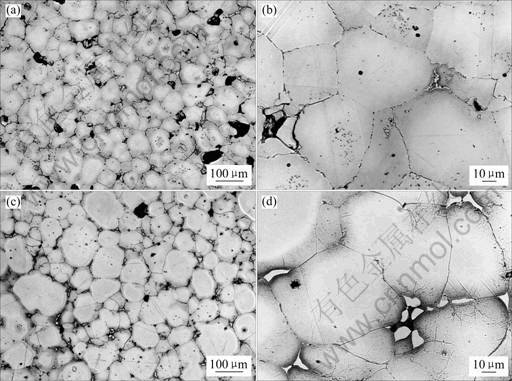
Fig.7 Optical microstructures of 718 MA (coarse)+CNP powder sintered at different temperatures for 60 min: (a), (b) 1 255 ℃; (c), (d) 1 280 ℃
4 Conclusions
1) A low density green preform made from coarse prealloyed powder blended with fine metal powder can be sintered to high density via a thermal process termed solid-supersolidus liquid phase sintering, which provides a larger sintering window.
2) The fast densification onset temperature is influenced by the particle size while the distortion onset temperature is solely dependent on the composition of the powder. Therefore, using fine powder can further enlarge the sintering window, which is important for processing in industry scale.
References
[1] DEKKER C. Manufacturing Engineering, 2002, 128(7): 58-60.
[2] GERMAN R M. Liquid Phase Sintering[M]. New York: Plenum Press, 1985.
[3] GERMAN R M. Inter J Powder Metall, 1990, 26(1): 23-35.
[4] LUND J A, BUTTERS R G, WEAVER C H. Powder Metall Inter, 1972, 4(4): 173-174.
[5] WRIGHT C S, OGEL B, LEMOISSON F, BIENVENU Y. Powder Metall, 1995, 38: 221-229.
[6] YU C H, SUZUKI M, SHIBATA H, EMI T. Mater Trans Japan Inst Metals, 1996, 37: 1251-1257.
[7] LAL A, LIU J, SHOALES G A, GERMAN R M. Advances in Powder Metallurgy & Particulate Materials, 1998, 3: 12.33-12.43.
[8] LIU J, LAL A, GERMAN R M. Acta Materialia, 1999, 47: 4615-4626.
[9] LIU J, RYNERSON M. Blended Powder Solid-Supersolidus Liquid Phase Sintering[P]. US P 6746506. 2004.
[10] GERMAN R M. Particle Packing Characteristics[M]. Princeton: Metal Powder Industries Federation, 1989.
[11] GERMAN R M. Sintering Theory and Practice[M]. New York: John Wiley and Sons, Inc, 1996.
[12] GRUBE G, SCHLECHT H. Sintering of metal powder and properties of metal compacts[J]. Z Electrochemie, 1938, 44: 367-374.
(Edited by CHEN Wei-ping)
Corresponding author: LIU Jian-xin; E-mail: Jason.liu@prometal.com; Jason.liu@exone.com