J. Cent. South Univ. (2017) 24: 2172-2179
DOI: https://doi.org/10.1007/s11771-017-3626-2

Coupled effect of viscosity enhancing admixtures and superplasticizers on rheological behavior of cement paste
YUAN Qiang(元强), LIU Wen-tao(刘文涛), WANG Chao(王超),
DENG De-hua(邓德华), LIU Zan-qun(刘赞群), LONG Guang-cheng(龙广成)
School of Civil Engineering, National Engineering Laboratory for High Speed Railway Construction,Central South University, Changsha 410075, China
Central South University Press and Springer-Verlag GmbH Germany 2017
Abstract: The coupled effect of viscosity enhancing admixtures (VEA) and superplasticizer (SP) on the rheological behavior of cement paste was investigated in this work. Two types of VEAs, including hydroxypropyl methylcellulose (HPMC) and Welan gum, and two types of SPs, i.e. polycarboxylate (PCA) and polynaphthalenesulfonate (PNS) were used as admixtures for cement paste. Rheological curves of cement paste and simulated pore solution containing VEA and SP were tested. Simulated pore solution test results show that molecules of different SPs may generate different effects on the viscosity of VEA solutions. Hershel-Bulkley (H-B) model was used to fit rheological curve of cement paste. Strong interaction between PNS and HPMC was observed in this work.
Key words: rheology; cement; viscosity enhancing admixture; superplasticizer
1 Introduction
Viscosity enhancing admixture (VEA) is an important chemical admixture used to modify rheological behavior of cement-based materials, such as self-compacting concrete, anti-washout concrete, shotcrete, and grout for post tension duct. With increasing demand of self-compacting concrete (SCC), the use of VEA is becoming quite common. According to the composition of concrete, SCC can be classified into powder-type SCC, VEA-type SCC and combined- type SCC [1]. The stability of the latter one depends on VEA, which is often used in combination with superplastisizer (SP) in cement-based materials. On one hand, VEA increases the viscosity of liquid phase, and stabilizes the suspension. On the other hand, SP disperses powder in liquid phase. Thus, a highly dispersed and stable suspension can be achieved by the combined action of VEA and SP. This is desired for highly flowable cement-based materials [1–4].
The dispersed mechanisms of SP are quite well understood. The adsorption of molecular of SP onto the cement particles generates a well-dispersed system by the action of electro-static repulsion and steric repulsion singly or collectively. Polynaphthalene type superplasticizer is a typical electro-static one, and polycarboxylates type superplasticizer is a representative steric repulsion one [5].
Mailavaganam categorized anti-washout admixtures and pumping aid into five classes according to their actions in concrete, among which water-soluble high molecular polymer is most often used [6]. It increases the viscosity of suspension by increasing the viscosity of water. KAWAI [7] classified water-soluble polymers as natural, semi-synthetic and synthetic polymers.
Natural polymers include starches Welan gum (WG), gum arbic, etc. Semi-synthetic polymers include cellulose-ether derivatives, such as hydroxypropyl methyl cellulose (HPMC), and carboxy methyl cellulose (CME). Synthetic polymers include polyermers based on ethylene. The mechanism of action of a VEA depends on the type and concentration of polymer in use. KHAYAT [8] summarized the mechanism of action into three classes: 1) Adsorption. The long-chain polymer molecules adsorb and fix part of the mixing water, and thereby swelling. This increases the viscosity of the mixing water and that of the cement-based materials. 2) Association. Molecules in adjacent polymer chains can develop attractive forces, thus causing a gel formation and an increase in viscosity. 3) Intertwining. At low shear rates and high polymer concentration, the polymer chains can intertwine and entangle, resulting in an increase in viscosity. Such entanglement can disaggregate and the polymer chains can align in the direction of the flow at high shear rates, hence resulting in shear thinning behavior.
The combined use of SP and VEA has become a quite common technique for cement-based materials, SARIC-CORIC et al [3] evaluated the rheological properties, stability, setting, hydration, strength and pore size distribution of grout made with 0.4 (water to cenent ratio) containing VEA and SP (polynaphtalene sulfonate (PNS) based and polymelamine sulfonate (PMS) based). The results showed that pastes prepared with PMS based SP exhibited higher yield value and viscosity than those prepared with PNS-based high-range water-reducing admixture (HRWR), and discussion on this result is not given. SAKATA et al [2] developed a new type of admixture combining with Welan gum and superplasticizer, based on their coupled effect on rheological behavior of cement paste. Several other publications dealt with this issue [4, 9, 10]. However, the interactions between SP and VEA are not well documented.
Adsorption of high molecular polymer onto the cement particles has always been one of the key issues of the area of cement. When VEA and SP are added into cement paste together, there exists a competitive adsorption of polymers at the surface of cement particles [11, 12]. It has a great influence on the rheological behavior of cement paste. However, due to the limitation of testing technique, the adsorption of different types of polymers on the surface of cement cannot be quantified respectively. Adsorption is most often measured by solution depletion method which assumes that the difference of polymer concentrations before and after contact with cement is due to adsorption of the polymers onto the surface of cement particles. Polymer concentration of the aqueous solution obtained by centrifugation is analyzed by total organic carbon (TOC), which cannot distinguish relative concentration of mixed polymer.
Apart from adsorption of polymers, the viscosity of interstitial solution plays an important role in the rheological behavior of cement-based materials. Interstitial solution of fresh cement mixture containing VEA and SP consists of various ions (OH–, Ca2+, SO42–, K+, Na+), macromolecules of VEA and SP. To the best of our knowledge, the interactions among these species and their effect on the rheological behavior of mixture are seldom reported. In this work, the effects of inorganic ions and high polymer molecules on the rheological behavior of interstitial solution were studied. Rheological behaviors of cement pastes containing different high polymer molecules were also evaluated.
2 Experimental
2.1 Materials
The cement was a type P·II 52.5R Portland cement, complied with the requirements of Chinese standard GB175–2007. The chemical and physical properties of cement are given in Tables 1 and 2.
Table 1 Chemical and physical properties of cement (mass fraction, %)

Table 2 Physical properties
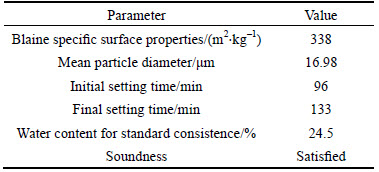
A superplasticizer of polynaphthelene sulfonate (PNS) with solid content of 30% and specific gravity of 1.11, as well as a polycarbonxylate (PCA) with solid content of 30% and specific gravity of 1.07 were used in this investigation. Water in the SPs was accounted in the mixing water. VEAs of HPMC and Welan gum were used in this work, which are solid type. Deionized water was used. Chemicals used in this work were analytical reagent.
Experiments were carried out in two groups. The effect of simulated pore solutions and SP (PCA and PNS) on the rheological behavior was studied in group 1. OH–, Ca2+, SO42–, K+ and Na+ are main ions of pore solution of cement paste, among which OH–, K+ and Na+ are the dominated ones. Thus, chemical of KOH and NaOH were employed to prepare simulated pore solution. Simulated pore solutions of low alkalinity (NaOH 25 mol/m3 and KOH 80 mol/m3) and high alkalinity (NaOH 200 mol/m3 and KOH 500 mol/m3) were prepared [13, 14], as shown in Table 3. The rheological behavior of pore solutions containing different SPs and VEAs were tested, testing samples are listed in Table 3.
In group 2, rheological behavior of cement pastes containing SPs and VEAs were tested. In order to evaluate the effect of VEAs on the rheological behavior of cement paste with solid volume fraction of 0.47, the dosage rate of SPs is fixed at 3% (by the mass of cement) which is a little higher than its saturation point, while varying the dosage rate of VEAs from 0% to 0.08% (by the mass fraction of cement), as shown in Table 4.
Table 3 Compositions of aqueous solutions (Unit: g)
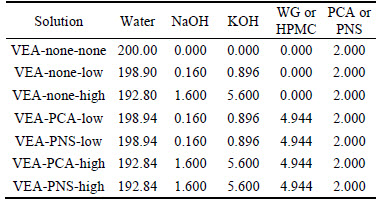
Table 4 Parameters of mixed cement paste
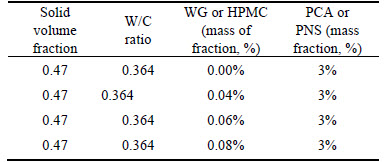
2.2 Mixing procedure
Welan gum or HPMC were first added into deionized water, and then stirred until a homogenous solution was obtained. Since the swelling and dissolution of Welan gum or HPMC need time, and the solutions with Welan gum and HPMC show time-dependent viscosity, prepared solution with Welan gum or HPMC were sealed and stored for 24 h before use.
For group 1 test, the weighted KOH and NaOH were first added into deionized water, and then stirred until a homogenous simulated pore solution was obtained. VEA solution was added into prepared simulated pore solution while stirring for 18 min, then SP was introduced, and stirred for another 2 min.
All cement mixtures were prepared in a 1 L high-shear mixer rotating at approximately 360 r/min. The temperature of materials and mixer were controlled to keep the temperature of pastes at (20±1) °C. Water and VEA solution and SP solution were first introduced and followed by cement into the bowl of mixer rotating at speed of 60 r/min for 30 s, and the mixture was mixed at the speed of 120 r/min for 90 s and followed by a low speed mixing for another 30 s.
2.3 Rheological test
Rheology was measured using a “RheoPlus QC” coaxial cylinder rotary rheometer. The type of rotator is ST22-4V-40 and the inside sizes of outer tube is d42 mm×118 mm. The temperature of the samples was controlled by a water bath at (20±1) °C during test. The rheological curves of pastes were tested immediately after mixing. The rheological test protocol for paste consists of shearing the paste at a high shear rate of 200 s-1 for 60 s to ensure complete structural breakdown of the structure. The flow curve was then determined by increasing the shear rate from 0 to 200 s-1 during 300 s. The rheological test for solution consists of logarithmic increase of shear rate ranging from 0 to 100 s-1, and then to 0 s–1.
3 Results and discussion
3.1 Rheological behaviour of aqueous solution of VEAs
3.1.1 Effect of alkalinity
The effect of alkalinity on the rheological behaviour of aqueous solutions of VEAs is given in Fig. 1. Both Welan gum and HPMC aqueous solutions present an obvious shear thinning pattern, but show different responses to alkalinity. Viscosity of HPMC aqueous solutions decreases with the increase in alkalinity, whereas viscosity of Welan gum aqueous solutions slightly increases with the increase in alkalinity. For example, at the shear rate of 100 s–1, the viscosity of HPMC aqueous solutions is 1.260 Pa·s, the addition of high alkalinity results in the decrease in viscosity of 64% to 0.455 Pa·s; whereas the viscosity of Welan gum aqueous solutions is 0.931 Pa·s, the addition of high alkalinity results in the decrease in viscosity of 12% to 0.819 Pa·s. It can be concluded that alkalinity affects the rheological behavior of HPMC aqueous solution much more significantly than that of Welan gum aqueous solution.
3.1.2 Effect of SPs
The effect of SPs on the rheological behaviour of aqueous solutions of VEAs is given in Fig. 2. It can be observed that PCA has minor effect on rheological behavior of aqueous solutions of VEAs, whereas PNS shows different effects. The addition of PNS causes slight change in rheological behavior of aqueous solutions of Welan gum. However, the addition of PNS significantly changes the rheological behavior of aqueous solutions of HPMC. The viscosity of aqueous solutions of HPMC with PNS is 20 times as that without PNS at low shear rate.
3.1.3 Coupled effect of SPs and alkalinity
The coupled effect of SPs and alkalinity on the rheological behaviour of aqueous solutions of VEAs is given in Fig. 3. It can be observed that combinations of SPs and alkalinity have minor effect on the rheological behaviour of aqueous solutions of Welan gum. PNS has most significant influence on rheological behavior of aqueous solutions of HPMC. High alkalinity can decrease the impact.
3.1.4 Discussion
Welan gum is an anionic polysaccharide, a non-gelling polysaccharide [15], and consists of pentasaccharide repeating unit, β-1, 3-D-glucopyranosyl, β-1, 4-D-glucuronopyranosyl, β-1, 4-D-glucopyranosyl and β-1, 4-L-rhamnopyranosyl, and a single monosaccharide side-chain at O-3 of the 4-linked glucopyranosyl. The monosaccharide may be either l-rhamnopyranosyl or l-mannopyranosyl in the approximate ratio molar 2:1, and about half of the repeat units or more have acetyl and glyceryl substituents [15, 16].
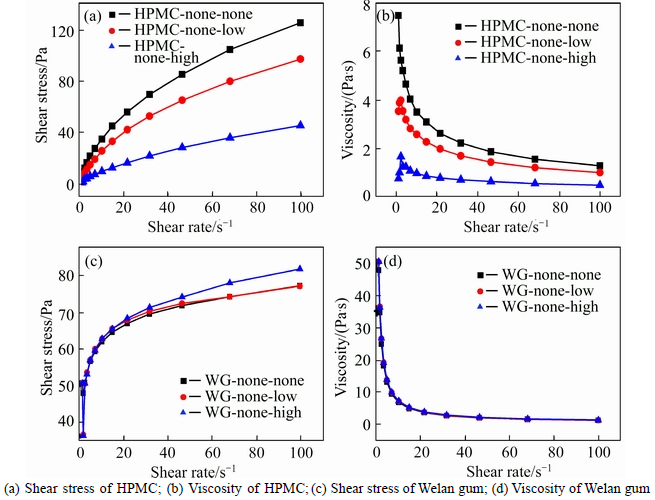
Fig. 1 Effect of alkalinity on rheological behavior of VEAs aqueous solution:
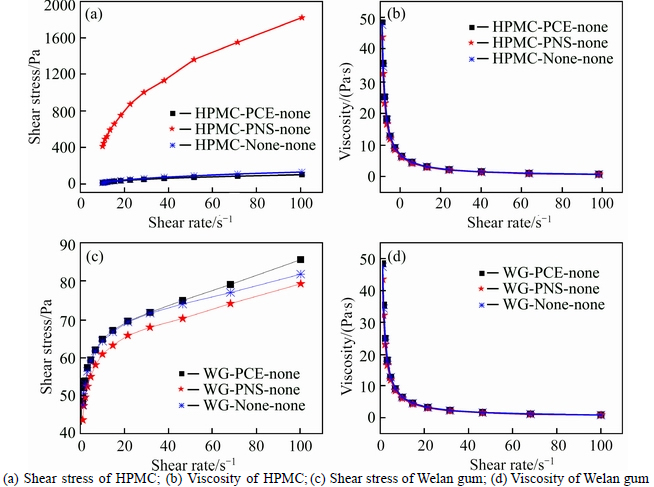
Fig. 2 Effect of SPs on rheological behavior of VEAs aqueous solutions with low alkalinity:
The rheological behavior of Welan gum solutions has rarely been reported [17]. XU et al [17] carried out studies on the effect of concentrations, temperatures and salinities on rheological behavior of Welan gum solutions. Shear thinning behavior of Welan gum solutions was also observed, and is related to the orientation of macromolecules along the stream line of the flow [18]. At the low shear rate, the stretching polysaccharide molecules intertwined to form aggregates, resulting in high viscosity, while high shear rate destroys aggregates and dispersing molecules arranged along the flow direction, resulting in low apparent viscosity [19]. This agrees with the results obtained in this work. Another similar trend is also observed by XU et al [17] that viscosity of Welan gum solutions decreases in the presence of inorganic salt, and change little with salt concentration. The electrostatic repulsion between charged groups of macromolecules is screened by inorganic cations, making macromolecules to adopt a more compact conformation. In addition, inorganic ions have a strong hydration, and can compact the hydrated layer around polysaccharide molecules, resulting in the decline in viscosity.
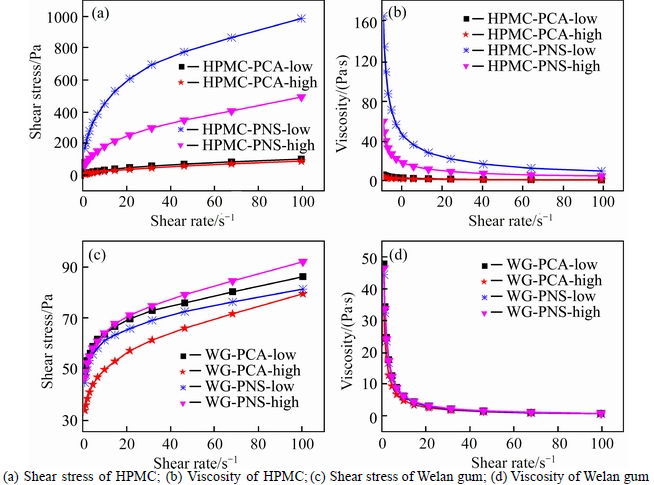
Fig. 3 Coupled effect of SPs and alkalinity on rheological behavior of VEAs aqueous solutions:
PCA is a comb-like molecular structure composed of a carboxylic acid main chain and long polyoxyethylene side chains. It develops dispersion effect for cement paste by adsorption on cement particle or cement hydration products and producing the steric hindrance effect on cement particles. PCA has little influence on the rheological behavior of Welan gum. PNS consists of long-chain anionic surfactants with a large number of polar groups in the hydrocarbon chain. When adsorbed on cement particles, the surfactant imparts a strong negative charge. Both PCA and PNS have minor effect on rheological behavior of Welan gum solution. Compared to PCA, PNS decreases the viscosity of welan gum solution more pronounced.
Hydroxypropyl methyl cellulose (HPMC), which is a non-ionic cellulose derivative, contains both hydrophobic and hydrophilic structural units. Its gelation properties can be modified by temperature, salts and surfactants [20–25]. Additionally, the effect of cations on gelation temperature is less significant than that of anions [24, 26].
In HPMC solution, water molecules form hydrogen bonding with the hydroxyl group of the HPMC (intermolecular chains hydrogen bonding) and structure water cages surrounding hydrophobic clusters of the HPMC chains. Salt ions tend to attract and interact with water molecules strongly because of its strong hydration abilities. Thus, the intermolecular hydrogen bonding of HPMC is weakened and easily disrupted. At the same time, water cages are more easily broken. With increasing NaCl concentration, the salt ions attract more hydration water molecules around them [20, 24]. In addition, macromolecules may adsorb salt ions, and the electrostatic repulsion between charged groups of macromolecules makes macromolecules to adopt a more compact conformation. As a consequence, salt decreases the viscosity of HPMC solution more pronounced than that of Welan gum solution.
There is a chain contraction due to the intense intramolecular hydrophobic association junctions [27]. The binding of surfactant molecules to HPMC may modify the stiffness and this may, in turn, induce a coil-to-globule transition. When PNS is added into HPMC solution, a significant increase in the viscosity is observed. It is speculated that micelle formation of PNS takes place along the HPMC chains and progressively converts the non-ionic polymer into a polyelectrolyte. Repulsion among the negative charges of the polymer– surfactant complex causes the polymer to swell leading to a consequent increase in the shear viscosity. However, the addition of PCA does not affect rheological behavior of HPMC solution much, probably because of its low charging density.
3.2 Rheological behaviour of cement paste
3.2.1 Effect of HPMC content
Effect of HPMC content on rheological behavior of cement paste (Vs is 0.47) containing different SPs is given in Fig. 4. Non-newton fluid is often described by Hereshel-Bulkley (H-B) model,
(1)
where τ is shear stress, Pa; τ0, HB is yield shear stress, Pa; K is consistency factor, Pa·sn;
is shear rate, s–1; and n is flow index. H-B model is used to fit the rheological curve, and fitted parameters are given in Table 5.
It can be observed from Fig. 4(a) that the addition of HPMC increases the viscosity of cement paste with saturated PCA. As the addition level of HPMC increases, the viscosity of paste gradually increases. Table 5 illustrates that yield stress of paste increases gradually with the increase in addition level of HPMC, and values of n are greater than 1, i.e. cement paste is shear thickening. It seems that the addition of HPMC may aggravate the trend of shear thickening. It can be seen from Fig. 4(b) that the addition of HPMC increases the viscosity of cement paste with saturated PNS, especially at low shear rate. The addition level of HPMC (0.04%–0.08%) does not affect rheological curve much. Table 4 indicates that yield stress of paste increases significantly with the increase in addition level of HPMC. Furthermore, the addition of HPMC changes paste from shear thickening to shear thinning.
3.2.2 Effect of Welan gum content
Effect of Welan gum content on rheological behavior of cement paste (Vs is 0.47) containing different SPs is given in Fig. 5. H-B model is used to fit the rheological curve, and fitted parameters are given in Table 6.
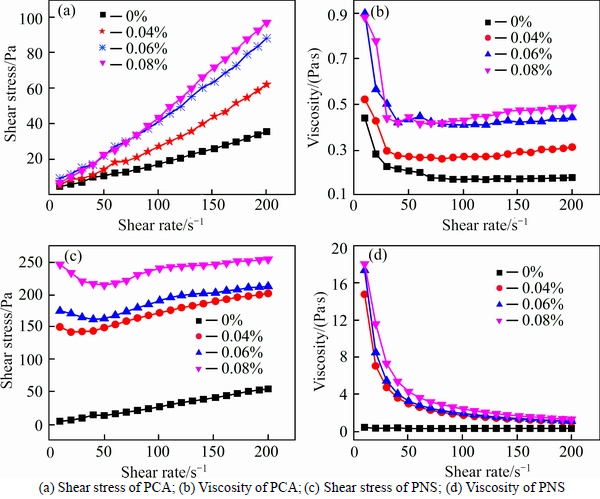
Fig. 4 Effect of HPMC on rheological behavior of cement paste containing different SPs:
Table 5 Parameters of H-B model for cement paste containing HPMC and different SPs
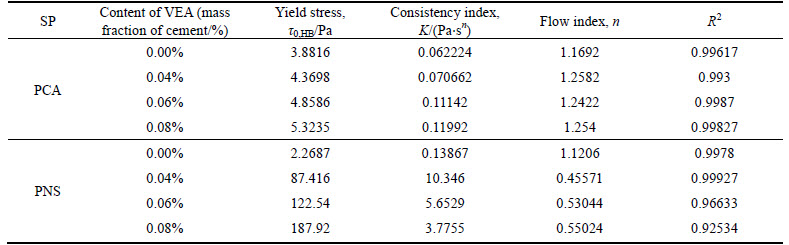
It can be observed from Fig. 5(a) that the addition of welan gum increases the viscosity of cement paste with saturated PCA. As the addition level of welan gum increases, the viscosity of paste gradually increases. The shapes of rheological curves are very similar. Table 6 illustrates that yield stress of paste increases gradually with the increase in addition level of welan gum, and values of n are greater than 1, i.e. cement paste is shear thickening. Obviously, the addition of welan gum may aggravate the trend of shear thickening, i.e. the higher addition level of welan gum, the more pronounced shear thickening.
It can be seen from Fig. 5(b) and Table 6 that rheological behavior of cement paste with saturated PCA and welan gum presents similar trend to that of cement paste with PNS and welan gum.
3.2.3 Discussion
SPs affect the rheological behavior of cement paste with HPMC differently. It is agreed with the test results of HPMC aqueous solutions. Cement pastes containing HPMC and PNS present a much higher yield stress than that of cement with HPMC and PCA. This is attributed to the formation of a chemical gel resulting from the incompatibility between HPMC and PNS. Whereas Welan gum does not exhibit an incompatibility with PNS [8, 28]. In addition, a pronounced shear thinning behavior is observed. This can be explained by the following reasons: at low shear rate, the stretching macromolecules intertwin to form aggregates, resulting in high viscosity, while high shear rate destroys aggregates and dispersing molecules arranged along the flow direction, resulting in low apparent viscosity.
No significant difference in rheological behavior of cement pastes containing Welan gum and SPs is observed. This is also agreed with the test results of welan gum aqueous solutions. The discrepancy is that shear thinning behavior is observed on Welan gum solution, whereas, shear thickening behavior happens to cement paste with Welan gum and SPs. This implicitly indicates that cement particles are dispersed well, and no aggregations of macromolecules are formed. But the mechanisms behind this phenomenon need in-depth study.
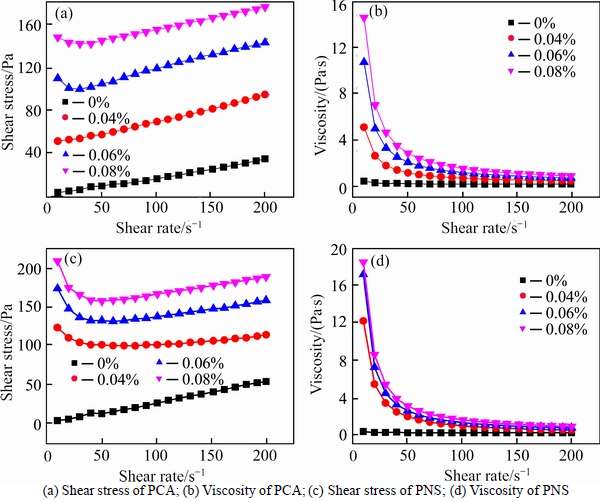
Fig. 5 Effect of welan gum on rheological behavior of cement paste containing different SPs:
Table 6 Parameters of H-B model for cement paste containing Welan gum and different SPs
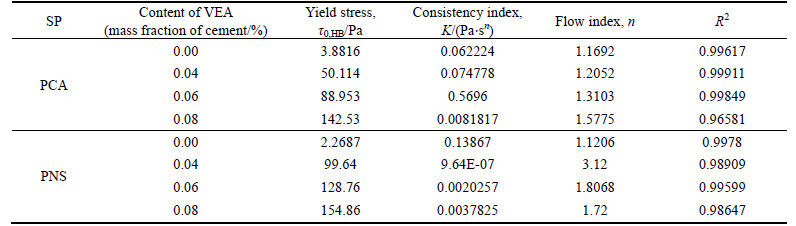
4 Conclusions
Different superplasticzers and viscosity enhancing agents are used to tune the rheological behavior of cement-based materials, the following conclusions can be made.
1) Strong interaction between PNS and HPMC is observed in this work.
2) Rheological behavior of macromolecules solution may be different from that of suspension with macromolecules.
3) Special attentions should be paid to the effect of ionic concentrations of pore solution, and the interaction between polymers and surfactants when using various macromolecules in different cementing materials.
References
[1] SCHUTTER G D, BARTOS P J M. Self-compacting concrete [M]. Scotland UK: Whittle publishing, 2008.
[2] SAKATA N, YANAI S, YOKOZEKI K, MARUYAMA K. Study on new viscosity agent for combination use type of self-compacting concrete [J]. Journal of Advanced Concrete Technology, 2003, 1(1): 37–41.
[3] SARIC-CORIC M, KHAYAT K H, TAGNIT-HAMOU A. Performance characteristics of cement grouts made with various combinations of high-range water reducer and cellulose-based viscosity modifier [J]. Cement and Concrete Research, 2003, 33(12): 1999–2008.
[4] KHAYAT K H, YAHIA A. Effect of welan gum-high-range water reducing combination on rheology of cement grout [J]. ACI Materials Journal, 1997, 94(5): 365–372.
[5] PENG Jian-wei, DENG De-hua, LIU Zan-qun, YUAN Qiang, YE Tao. Rheological models for fresh cement asphalt paste [J]. Construction and Building Materials, 2014, 71: 254–262.
[6] RAMACHANDRAN V S. Concrete admixtures handbook: Properties, science and technology [M]. 2nd ed. USA: Noyes Publications, 1997.
[7] KAWAI T. Non-dispersible underwater concrete using polymers. Marine Concrete [M]. UK: International Congress on Polymers in Concrete. Brighton, 1988.
[8] KHAYAT K H, SARIC-CORIC M. Effect of welan gum– Superplasticizer combination on properties of cement grouts [J]. ACI Special Publication, 2000, 195: 249–268.
[9] KHAYAT K H, SARIC-CORIC M. Effect of welan gum– Superplasticizer combination on properties of cement grouts [J]. ACI, 2000, 195(S): 249–268.
[10] KAWAI T, OKADA T. Effect of superplasticizer and viscosity- increasing admixture on properties of lightweight aggregate concrete [J]. ACI, 1989, 119(S): 583–604.
[11] HOT J, HELA BESSAIES-BEY, CORALIE BRUMAUD, DUC M, CASTELLA C, ROUSSEL N. Adsorbing polymers and viscosity of cement pastes [J]. Cement and Concrete Research, 2014, 63: 12–19.
[12] BAUMANN R, ROUSSEL N. Consequences of competitive adsorption between polymers on the rheological behaviour of cement pastes [J]. Cement & Concrete Composites. 2014, 54(5): 17–20.
[13] SCHMIDT F, F S.
, SCHMIDT F,
F S. A method of calculation of the chemical composition of the concrete pore solution [J]. Cement and Concrete Research, 1993, 23(5): 1159–1168.
[14] DIAMOND. S. Effects of two danish fly ashes on alkali contents of pore solutions of cement-flyash pastes [J]. Cement and Concrete Research, 1981, 11(3): 383–394.
[15] KAUR V, BERA M B, PANESAR P S, KUMAR H, KENNEDY J F. Welan gum: Microbial production, characterization, and applications [J]. International Journal of Biological Macromolecules. 2014, 65: 454–461.
[16] TAKO M, TERUYA T, TAMAKI Y, KONISHI T. Molecular origin for rheological characteristics of native gellan gum [J]. Colloid and Polymer Science, 2009, 287: 1445–1454.
[17] XU Long, XU Gui-ying, LIU Teng, CHEN Yi-jian, GONG Hou-jian. The comparison of rheological properties of aqueous welan gum and xanthan gum solutions [J]. Carbohydrate Polymers, 2013, 92(1): 516–522.
[18] CHAGAS B S, DILON L P, MACHADO J R, HAAG R B, LUCAS E F. Evaluation of hydrophobically associated polyacrylamide- containing aqueous fluids and their potential use in petroleum recovery [J]. Journal of Applied Polymer Science, 2004, 91(6): 3686–3692.
[19] OH M H, SO J H, YANG S M. Rheological evidence for the silica-mediated gelation of xanthan gum [J]. Journal of Colloid and Interface Science, 1999, 216(2): 320–328.
[20] LIU S Q, JOSHI S C, LAM Y C. Effects of salts in the hofmeister series and solvent isotopes on the gelation mechanisms for hydroxypropylmethylcellulose hydrogels [J]. Journal of Applied Polymer Science, 2008, 109(1): 363–372.
[21] XU Y, LI L, ZHENG P, HU X. Controllable gelation of methylcellulose by a salt mixture [J]. Langmuir, 2004, 20(15): 6134–6138.
[22] PYGALL SR, GRIFFITHS PC, WOLF B, TIMMINS P, MELIA C D. Solution interactions of diclofenac sodium and meclofenamic acid sodium with hydroxypropyl methylcellulose (HPMC) [J]. International Journal of Pharmaceutics, 2011, 405(1,2): 55–62.
[23] SILVA S M C, ANTUNES F E, SOUSA J J S, VALENTE A J M, PAIS A A C C. New insights on the interaction between hydroxypropylmethyl cellulose and sodium dodecyl sulfate [J]. Carbohydrate Polymers, 2011, 86(1): 35–44.
[24] ALMEIDA N, RAKESH L, ZHAO J. Monovalent and divalent salt effects on thermogelation of aqueous hypromellose solutions [J]. Food Hydrocolloids, 2004, 36: 323–331.
[25] KHAN I A, ANJUMA K, KOYAB P A, KABIR-UD-DIN. Effect of inorganic salts on the clouding behavior of hydroxypropyl methyl cellulose in presence of amphiphilic drugs [J]. Colloids and Surfaces B: Biointerfaces, 2013, 103: 496–501.
[26] ALEXANDRIDIS P, HOLZWARTH J F. Differential scanning calorimetry investigation of the effect of salts on aqueous solution properties of an amphiphilic block copolymer (poloxamer) [J]. Langmuir, 1997, 13: 6074–6082.
[27] HOFF E,
B, LINDMAN B. Polymer-surfactant interactions in dilute mixtures of a nonionic cellulose derivative and an anionic surfactant [J]. Langmuir, 2001, 17(1): 28–34.
[28] KAWAI T, OKADA T. Effect of superplasticizer and viscosity- increasing admixture on properties of lightweight aggregate concrete [J]. ACI, 1989, 119(S): 583–604.
(Edited by FANG Jing-hua)
Cite this article as: YUAN Qiang, LIU Wen-tao, WANG Chao, DENG De-hua, LIU Zan-qun, LONG Guang-cheng. Coupled effect of viscosity enhancing admixtures and superplasticizers on rheological behavior of cement paste [J]. Journal of Central South University, 2017, 24(9): 2172–2179. DOI:https://doi.org/10.1007/s11771-017-3626-2.
Foundation item: Project(2017YFB0310101) supported by National High Technology Research and Development Program of China; Project(51778269) supported by the National Natural Science Foundation of China
Received date: 2015-09-16; Accepted date: 2016-05-11
Corresponding author: YUAN Qiang, Associate Professor, PhD; Tel: +86–13548955591; E-mail: yuanqiang@csu.edu.cn