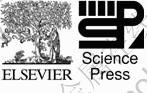
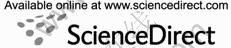
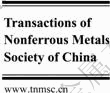
Trans. Nonferrous Met. Soc. China 22(2012) 2146-2152
Magnetostriction and structural characterization of Fe-Ga bulk alloy prepared by copper mold casting
HU Yong1, 2, DING Yu-tian1, 2, ZHANG Yan-long1, WANG Guo-bin1, ZHOU Zhi-guang1
1. State Key Laboratory of Gansu Advanced Nonferrous Metal Materials,Lanzhou University of Technology, Lanzhou 730050, China;
2. Wenzhou Pump & valve Engineering Research Institute of Lanzhou University of Technology,Wenzhou 325105, China
Received 26 September 2011; accepted 25 April 2012
Abstract: Rapidly solidified Fe100-xGax (x=16-20) alloy rods were prepared by induction melting and copper mold casting under the protection of inert gases. The optical microscopy observation shows that the large and elongated columnar grains grow along the radial direction, which is parallel to the temperature gradient direction. The <110> preferred orientation texture along the axial direction of the rod was detected by XRD. With the increase of Ga content, the saturation magnetization (Ms) of the alloys decreases distinctly and the dynamic response in low magnetic field increases drastically, the maximum longitudinal saturation magnetostriction for as-cast Fe82Ga18 alloy rods is 92×10-6 under an applied magnetic field strength of 30 kA/m. The large magnetostriction of Fe100-xGax alloys is attributed to the rapidly solidified disordered A2 phase and the high concentration of short range order of Ga atom clusters, which are arranged in the <100> direction and finally trigger the formation of modified-DO3 structure, just as shown by the split of the (200) diffraction peak. Ordered DO3 phase is not conducive to the magnetostriction.
Key words: magnetostriction; rapid solidification; Fe-Ga alloy; oriented polycrystalline; microstructure
1 Introduction
Magnetostrictive materials such as Terfenol alloys are widely used in various sensor and actuator applications due to their reversible strains in the presence of an applied magnetic field and changes in magnetic properties on the application of stress [1]. However, they are brittle, require large fields for saturation and are expensive due to the high costs of Tb and Dy. These factors limit the applications of these materials. Recently, it has been recognized that the magnetostriction of Fe changes drastically with the addition of non-magnetic element Ga [2] which has the potential to serve as magnetostrictive material due to its high mechanical strength, good ductility, large magnetostriction at low saturation fields and low cost relative to the Terfenol alloys [3].
The magnetostriction and microstructure of Fe-Ga alloys are closely related to the composition, preparation technology of alloy and the history of heat treatment [4]. CLARK et al [4] studied the magnetic, magnetostrictive and elastic properties of Fe100-xGax (x=15-20) single crystals which exhibited very good properties. They also reported that Fe100-xGax single crystals water-quenched from temperatures of above 800 ℃ with A2 structure showed larger magnetostriction [5]. Polycrystalline Fe-Ga alloy has lower magnetostriction ((80-100)×10-6) than single crystal. However, directional solidified textured polycrystalline Fe-Ga alloys have been reported to possess a magnetostriction of (220-270)×10-6 [6,7]. In recent years, a large number of papers have reported that rapidly quenched Fe-Ga ribbons have the large and even “giant” magnetostriction values ranging from -2100×10-6 to -3000×10-6 [8-10]. Whereas systematic research found that the giant magnetostriction of melt-spun Fe-Ga ribbons results from the unavoidable bending effect, which introduces stress into the sample and leads to large signals in the strain gauge. Although rapidly quenched materials having large magnetostriction values (exceeding 400×10-6) along preferred orientation are possible [11], the lacking is the dimensions of these melt-spun ribbons, being typically 0.2-0.3 cm wide and 50 μm thick, which restricts their mechanical applications. It is essential to develop new bulk Fe-Ga alloys with preferred-orientation as well as rapid solidification structure and large magnetostriction.
The structure, especially the disorder caused by the Ga substitution, plays an important role on the high magnetostriction found in single-crystalline Fe-Ga alloys. In the present work, copper mold-cast rapid solidification metohd, a kind of nonequilibrium solidifying technology that usually results in a more disordered state with additional Ga clusters, was used to prepare preferred orientation polycrystalline Fe100-xGax (x=16-20) bulk alloys, in order to investigate the magnetostriction properties, microstructural features and to elucidate the structure-property in correlation.
2 Experimental
Precursor ingots with a nominal composition of Fe100-xGax (x=16-20) were prepared from Fe (99.95% purity) and Ga (99.99% purity) using arc-melting under an argon atmosphere. Before arc melting, a piece of Ti was melted to absorb residual oxygen and water molecules in the chamber. Despite the higher vapor pressure of Ga relative to Fe at the melt temperature, melting procedures resulted in negligible loss of Ga. A low power arc was used in the early stages of arc-melting and the melting completed using a high-power arc to obtain ingots. Each ingot was re-melted four times and homogenized in a vacuum heat treatment furnace at 1273 K for 48 h in order to achieve complete composition homogenization.
Ingots broken into pieces of small masses were sealed in quartz tubes and melted using an induction equipment under the protection of an argon atmosphere. The melted alloy was solidified by ejecting the liquid into a water-cooled copper mold (as shown in Fig. 1(a)). Consequently, the rod might cause a temperature gradient (TG) during solidification from the surface in contact with the copper mold to the region far away from this contact (center of the rod), the Fe100-xGax (x=16-20) rods of 3 mm in diameter and 80 mm long were obtained (Fig. 1(b)).
In order to study the texture and grain morphology, the samples were polished and etched using 5% nital (5% nitric acid and 95% methyl alcohol) for 3 min, then a MeF3 optical microscope was used to research the structural characteristic. The preferred orientation and microstructure of the samples were detected by X-ray diffraction (XRD) using Cu Kα radiation at room temperature. The magnetostriction was measured by using standard strain gauges along the axis of the rods at room temperature. The cylindrical specimens with 3 mm in diameter and 10 mm in length used in XRD and magnetostriction experiments were sectioned from the middle part of the rods.
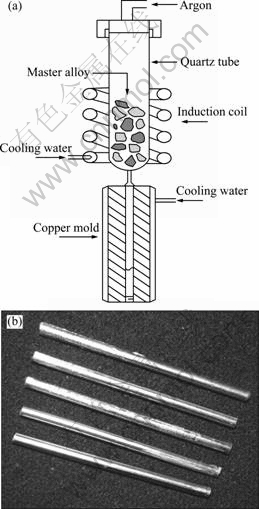
Fig. 1 Schematic diagram of production procedure (a) and photo of alloy samples (b)
3 Results and discussion
Figure 1 shows the schematic diagram of the production procedure and photo of the alloy samples. Figure 2 shows the X-ray diffraction patterns of the as-cast Fe100-xGax (x=16-20) alloys. Both samples have the (110), (200) and (211) characteristic peaks, which indicate a typical BCC structure of α-Fe phase. The substitution of Fe atoms by Ga atoms changes the peak positions corresponding to the BCC phase (A2) of pure α-Fe to smaller values of 2θ. From these results we can conclude that the addition of Ga atoms causes a dilatation in the lattice. Additionally, we did not obtain extra weak reflections in the diffraction profile; therefore, we can suggest no occurrence of the ordered B2 or DO3 phases. Due to the rapid cooling process, the disordered BCC A2 phase can be preserved at room temperature, which causes improved magnetic properties. From Fig. 2 we also can observe that the as-cast rod has a relative strong intensity in the (110) direction compared to the randomly oriented powder Fe-Ga alloys. This indicates that as-cast Fe100-xGax (x=16-20) alloy has <110> preferred-orientation of grains parallel to the rod axis.
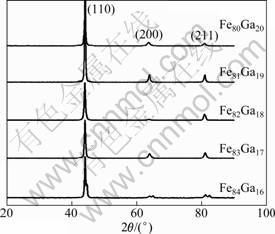
Fig. 2 XRD patterns of copper mold cast Fe100-xGax (x=16-20) alloy rods
The microstructure of the as-cast Fe100-xGax (x=16-20) alloys were investigated by the MeF3 optical microscope. Figure 3 shows the optical morphologies of the as-cast Fe100-xGax (x=16-20) alloy rods. Specimens prepared by copper mold are composed of three portions (as shown in Fig. 3). The surface of the as-cast rod is primarily chill zone of small equiaxed crystal. Columnar grains grew along the temperature gradient (TG) direction. At the center close to the axis of the rod, the texture is primarily large equiaxed crystal. Fe-Ga alloy matrix is softer. There are some holes and dark particles in the matrix during the polishing and corrosion. The emergence of the center hole is due to the fast cooling rate, which leads to the liquid metal having not enough time to feed during the solidification process. The existence of holes and crack also decreases the strength of the materials.
For the directional solidified specimen, the temperature gradient was found to be along the rod axis, which results in the preferential orientation of grains growing parallel to the axis. For the sample prepared by copper mold casting, a strong TG exists along the rod’s radial direction. Figure 3 shows that the grains grow perpendicularly to the axis.
Magnetostriction curves of the as-cast Fe100-xGax (x=16-20) alloys are shown in Fig. 4. All the specimens have similar curve shape, magnetostriction monotone increasing with the increase of magnetic field. With the increase of Ga content, the saturation magnetization (Ms) magnetic field of the alloys decreases distinctly and the dynamic response in low magnetic field increases drastically, the maximum longitudinal saturation magnetostriction of as-cast Fe82Ga18 alloy rods is 92×10-6 under an applied magnetic field strength of 30 kA/m. The magnetization decreases with the increase of Ga content. The reason is attributed to the dilution of iron magnetic moment by the addition of non-magnetic Ga element.
The interesting feature in the Fe-Ga system is the occurrence of several phase transformations indicated by the Fe-Ga phase diagram [12]. According to the work given by IKEDA et al [12] A2, B2, DO3, LI2 and B2-like phases may be obtained by different processes while the Ga content varied from 15% to 30%. A2 is a disordered phase while the other phases are ordered. The phase identification and distribution in Fe-Ga alloys by powder X-ray diffraction is problematic due to nearly identical lattice parameters of the various cubic phases. Further, the similarity in the atomic scattering factors of Fe and Ga in these structures results in super-lattice reflections of extremely low intensity. The magnetostriction coefficients strongly depend on the atomic arrangement. Phase structure, which may be obtained using various preparation methods, has shown that only B2-like (modified-DO3) phase appears to play a crucial rule on the large positive magnetostriction in the FeGa alloys [13], while the DO3 phase has a negative effect on magnetostriction.
In order to study the phase transformation and the microstructural features to elucidate the structure- property correlation in the system, different thermal treatments were applied to Fe82Ga18 alloy. The sample was heated at 1000 ℃ in a vacuum tube furnace for 1 h and annealed at 720 ℃ for 3 h. Then, the sample was cooled in two ways as follows: quenching in ice water and furnace-cooling.
Figure 5 shows the X-ray diffraction patterns of the Fe82Ga18 alloy in different states. All samples have the (110), (200) and (211) characteristic peaks, which indicate a typical BCC structure of α-Fe phase. From Fig. 5(a) we can observe that the as-cast rod has a strong relative intensity in the (110) direction. Compared to the randomly oriented powder Fe-Ga alloys, a <110> preferred-orientation indicates grains parallel to the rod axis. The diffraction patterns of the quenched and annealed alloys are similar to the as-cast rods (Figs. 5(b) and (c)) but the intensities of characteristic peaks (200) and (211) became strong. XRD pattern also shows that there is no evidence of any DO3 super-lattice reflections observed in the as-cast rods. The copper mold casting was successful in suppressing LRO DO3 as evidenced in the absence of (100) or (111) super-lattice reflection, which is not allowed for the A2 structure. However, a clear splitting of the 64°(200) reflections, which clearly indicates the presence of two-phases, can be seen from the enlarged figure in the top right of Fig. 5. Based on the work of CAO et al [14], we calculated that the low angle peak with lattice parameter of a1=0.2916 nm corresponds to DO3 phase, and the high angle peak with lattice parameter of a2=0.2903 nm corresponds to A2 phase.
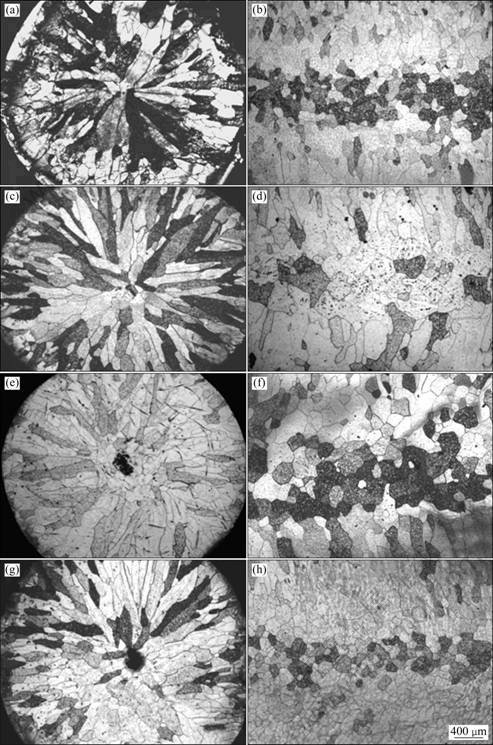
Fig. 3 Optical morphology images of sections of as-cast Fe100-xGax (x=16-19) alloy rods: (a) Fe84Ga16, transverse section; (b) Fe84Ga16, longitudinal section; (c) Fe83Ga17, transverse section; (d) Fe83Ga17, longitudinal section; (e) Fe82Ga18, transverse section; (f) Fe82Ga18, longitudinal section; (g) Fe81Ga19, transverse section; (h) Fe81Ga19, longitudinal section
Magnetostriction measurements of the Fe82Ga18 alloy under different conditions were performed using a strain gauge method along the axis of the rods at room temperature. From Fig. 6, as-cast Fe82Ga18 alloy has saturated magnetostriction of 92×10-6 under an applied magnetic field of 30 kA/m. The specimens after heat treatment have similar curve shape to the as-cast alloy. However, the magnetostriction of heat treated specimens shows a significant drop. The quenched sample has the saturated magnetostriction of 68×10-6 and the annealed sample has the saturated magnetostriction of 38×10-6 under the magnetic field strength of 20 kA/m.
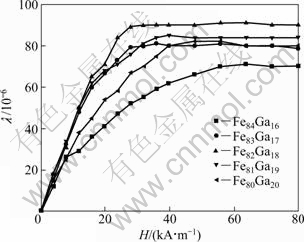
Fig. 4 Room temperature magnetostriction of Fe100-xGax alloys vs applied magnetic field
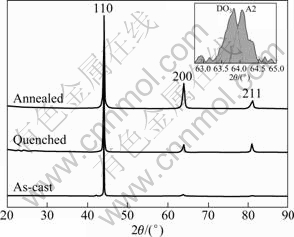
Fig. 5 XRD patterns of copper mold cast Fe82Ga18 alloy rods in different states (Insert graph is a partially enlarged XRD pattern of (200) diffraction peak of as-cast alloy)
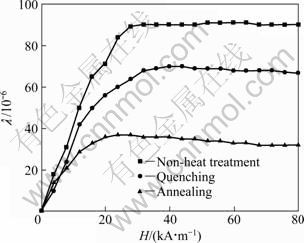
Fig. 6 Magnetostriction curves of Fe82Ga18 alloy under different heat treatment conditions
According to the phase diagram in Fig. 7, Fe-18%Ga (mole fraction) is located in the two-phase region. Assuming that this phase diagram is correct, the absence of (100) and/or (111) super lattice reflection is due to the quite small average size of any DO3 clusters; if the size of DO3 clusters is much smaller than the coherence length of the X-ray, then the diffraction pattern will only reveal features related to the A2 structure [15]. In fact, when the Fe82Ga18 alloy bar was cooled slowly from high temperature to room temperature, the alloy entered the BCC+LI2 binary area, and the meta-stable long-range-ordered DO3 phase separated out. Under our experiment condition, copper mold casting is a non-equilibrium solidification procedure. When the melt was cooled rapidly from high temperature to room temperature, the long range order of the DO3 phase was restricted. The A2 phase only present at high temperature can be reserved at room temperature and the sample maintains a disordered BCC structure. It is confirmed that the DO3 phase in the rapidly solidified bar does not bring any disadvantage to its magnetostriction performance, which is different from the standard DO3 phase in the slow-cooled bulk sample. As shown in Fig. 5, (200) diffraction peak of the quenched Fe82Ga18 alloy shows extremely weak split and diffuse reflections which deviate slightly from the exact (200) super lattice positions. However, the annealed Fe82Ga18 alloy shows much stronger and sharper reflections compared with as-cast and quenched samples, owing to the presence of LRO DO3 phase dispersed in A2 matrix. LOGROSSO et al [16] concluded that a split in the characteristic peak of an as-cast alloy could be attributed to the unsymmetrical DO3 phase of Fe3Ga in the Ga-rich region when the alloy was prepared using copper mold casting. In this unsymmetrical structure (modified-DO3 or B2-like), neighboring Ga atoms align along the [100] direction resulting in a pre-martensitic short-range strain order. A cluster centered around the next-nearest Ga pairs contains about 12 atoms, which causes a distortion of the crystal lattice in the neighboring region. LOGRASSO et al [16] proposed that the Ga-Ga pairs in such modified DO3 structure create a local stress couples to external strain and thereby soften the shear modulus. This results in an enhancement of magnetoelastic effect and an increasing magnetostriction. Additionally, rapid solidification leads to a more disordered state with supersaturated Ga atoms and much small size of modified DO3 clusters.
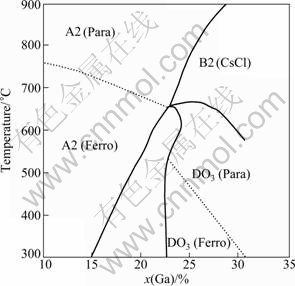
Fig. 7 Metastable Fe-Ga phase diagram redrawn from Ref. [12]
The A2 structure is of BCC with Ga atoms randomly substituting for iron atoms. DO3 is an ordered cubic structure with Ga atoms occupying specific positons (4a sites) and unit cell expanding about twice that of BCC iron [17]. Characteristic peaks of the DO3 phase and the α-Fe phase overlap in XRD diffraction profile because these two phases have similar crystal structures while Fe atoms and Ga atoms have similar scatter factors. Depending on the heat treatment, the correlation length increases sharply. The large increase in correlation length and increase in intensity of the reflection are consistent with exceeding the solubility limit of the A2 phase and the formation of long-range DO3 precipitates. The magnetostriction of Fe82Ga18 alloys after heat treatment shows a significant drop, as shown in Fig. 6. The magnetostriction property is strongly dependent on the short range order structure [18]. The samples prepared by copper mold casting have relatively high magnetostriction, which is caused by the large amount of short range order structure and the distorted unsymmetrical DO3 phase in the disordered A2 structure. The structure becomes homogenous after heat treatment, at the same time heat treatment promotes the meta-stable unsymmetrical modified DO3 structure to transform to the stable and ordered DO3 structure, which eventually leads to a decrease in magnetostriction.
4 Conclusions
1) Fe100-xGax (x=16-20) alloys prepared by copper mold casting are mainly in a disordered BCC A2 phase and have preferred <110> orientation along the axis direction. During rapid solidification, a strong temperature gradient perpendicular to the axis direction results in the formation of columnar crystals oriented toward the radial direction.
2) With the increase of Ga content, the saturation magnetization (Ms) of the alloys decreases distinctly and the dynamic response in low magnetic field increases drastically, and the maximum longitudinal saturation magnetostriction value of as-cast Fe82Ga18 alloy rods is 92×10-6 under an applied magnetic field strength of 30 kA/m.
3) The large magnetostriction of Fe100-xGax (x=16-20) alloys is attributed to the rapidly solidified disordered A2 phase and the high concentration of short range order of Ga atom clusters, which are arranged in the <100> direction and finally trigger the formation of modified DO3 structure, just as shown in the split of the (200) diffraction peak. Ordered DO3 phase is not conducive to the magnetostriction.
References
[1] SUMMERS E M, LOGRASSO T A, WUN-FOGLE M. Magnetostriction of binary and ternary Fe-Ga alloys [J]. Journal of Materials Science, 2007, 42(23): 9582-9594.
[2] CLARK E, RESTORFF J B, WUN-FOGLE M, HATHAWAY K B, LOGRASSO T A, HUANG M, SUMMERS E. Magnetostriction of ternary Fe-Ga-x (x=C,V,Cr,Mn,Co,Rh) alloys [J]. Journal of Applied Physics, 2007, 101(9): 09C507-1-09C507-3.
[3] CULLEN J R, CLARK A E, WUN-FOGLE M, RESTORFF J B, LOGRASSO T A. Magnetoelasticity of Fe-Ga and Fe-Al alloys [J]. Journal of Magnetism and Magnetic Materials, 2001, 226-230(part 1): 948-949.
[4] CLARK E, HATHAWAY K B, WUN-FOGLE M, RESTORFF J B, LOGRASSO T A, KEPPENS V M, PETCULESCU G, TAYLOR R A. Extraordinary magnetoelasticity and lattice softening in bcc Fe-Ga alloys [J]. Journal of Applied Physics, 2003, 93(10): 8621-8623.
[5] TOSHIYA T, KENJI H, TEIKO O, YASUBUMI F, TAKESHI K, CHIHIRO S. Characterization of magnetostrictive Fe-Ga-based alloys fabricated by rapid solidification [J]. Scripta Materialia, 2009, 60(10): 847-849.
[6] BASUMATARY H, PALIT M, CHELVANE J A, PANDIAN S, RAJA M M, CHANDRASEKARAN V. Structural ordering and magnetic properties of Fe100-xGax alloys [J]. Scripta Materialia, 2008, 59(8): 878-881.
[7] SRISUKHUMBOWORNCHAI N, GURUSWAMY S. Large magnetostriction in directionally solidified FeGa and FeGaAl alloys [J]. Journal of Applied Physics, 2001, 90(11): 5680-5688.
[8] ZHANG M C, JIANG H L, GAO X X, ZHU J, ZHOU S Z. Magnetostriction and microstructure of the melt-spun Fe83Ga17 alloy [J]. Journal of Applied Physics, 2006, 99(2): 023903-1-023903-3.
[9] ZHANG M C, GAO X X, JIANG H L, QIAO Y, ZHOU S Z. Effect of Ga content on the magnetostriction and microstructure of Fe-Ga ribbons [J]. Journal of Alloys and Compounds, 2007, 431(1-2): 42-44.
[10] ZHANG M C, GAO X X, JIANG H L, ZHU J, ZHOU S Z, HAO X P, WANG B Y. Atomic vacancies in Fe81Ga19 ribbons and its effect on the magnetostrictions [J]. Journal of Alloys and Compounds, 2008, 450(1-2): 28-30.
[11] GR?SSINGER R, TURTELLI R S, MEHMOOD N, HEISS S, M?LLER H, BORMIO-NUNES C. Giant magnetostriction in rapidly quenched Fe-Ga [J]. Journal of Magnetism and Magnetic Materials, 2008, 320(20): 2457-2465.
[12] IKEDA O, KAINUMA R, OHINUMA I, FUKAMICHI K, ISHIDA K. Phase equilibria and stability of ordered b.c.c phases in the Fe-rich portion of the Fe-Ga system [J]. Journal of Alloys and Compounds, 2002, 347(1-2): 198-205.
[13] XING Q, LOGRASSO T A. Phase identification of quenched Fe-25at. %Ga [J]. Scripta Materialia, 2009, 60(6): 373-376.
[14] CAO H, BAI F M, LI J F, VIEHLAND D D, LOGRASSO T A, GEHRING P M. Structural studies of decomposition in Fe-xat.% Ga alloys [J]. Journal of Alloys and Compounds, 2008, 465(1-2): 244-249.
[15] LOGRASSO T A, SUMMERS E M. Detection and quantification of DO3 chemical order in Fe-Ga alloys using high resolution X-ray diffraction [J]. Materials Science and Engineering A, 2006, 416(1-2): 240-245.
[16] LOGRASSO T A, ROSS A R, SCHLAGEL D L, CLARK A E, WUN-FOGLE M. Structural transformations in quenched Fe-Ga alloys [J]. Journal of Alloys and Compound, 2003, 350(1-2): 95-101.
[17] XING Q, DU Y, MCQUEENEY R J, LOGRASSO T A. Structural investigations of Fe-Ga alloys: Phase relations and magnetostrictive behavior [J]. Acta Materialia, 2008, 56(16): 4536-4546.
[18] LIU G D, LIU L B, LIU Z H, ZHANG M, CHEN J L, LI J Q, WU G H. Giant magnetostriction on Fe85Ga15 stacked ribbon samples [J]. Applied Physics Letters, 2004, 84(12): 2124-2126.
铜模铸造块体Fe-Ga合金的磁致伸缩性能和结构特征
胡 勇1, 2,丁雨田1, 2,张艳龙1,王国斌1,周质光1
1. 兰州理工大学 甘肃省有色金属新材料省部共建国家重点实验室,兰州 730050;
2. 兰州理工大学 温州泵阀研究院,温州 325105
摘 要:采用惰性气体保护下的感应熔炼铜模吹铸方法,制备具有非平衡快速凝固组织、晶粒沿温度梯度取向生长的Fe100-xGax(x=16~20)合金棒。XRD分析判定棒材主要为无序A2相结构且具有<110>轴向择优取向。随着Ga元素含量的增加,合金的饱和磁化强度明显降低,低场动态响应迅速增加。快速凝固获得的Fe82Ga18合金棒的纵向饱和磁致伸缩在30 kA/m磁场强度下为92×10-6。吹铸法制备的Fe100-xGax(x=16~20)合金棒材大的磁致伸缩性能源自快速凝固过程中所保留下来的高温无序A2相和合金内部所具有的大量沿<100>方向排列的短程有序富Ga原子团簇,这些富Ga团簇最终诱发畸变DO3相的生成,正如 (200)衍射峰发生劈裂所示。有序化转变生成的DO3相对该合金的磁致伸缩性能不利。
关键词:磁致伸缩;快速凝固;Fe-Ga合金;取向多晶;显微结构
(Edited by YANG Hua)
Foundation item: Project (11004091) supported by the National Natural Science Foundation of China; Project (Y4090219) supported by the Natural Science Foundation of Zhejiang Province, China; Project (0916RJZA025) supported by the Natural Science Foundation of Gansu Province, China; Project (Q201104) supported by the “Hongliu Outstanding Youth” Foundation of Lanzhou University of Technology, China
Corresponding author: HU Yong; Tel: +86-931-2757285; E-mail: luthuyong@163.com
DOI: 10.1016/S1003-6326(11)61441-6