Trans. Nonferrous Met. Soc. China 24(2014) 177-183
Rapid microwave irradiation fast preparation and characterization of few-layer graphenes
Xian LENG, Xiang XIONG, Jian-peng ZOU
State Key Laboratory of Powder Metallurgy, Central South University, Changsha 410083, China
Received 27 December 2012; accepted 5 June 2013
Abstract: Few-layer graphenes were fabricated from expandable graphite by rapid microwave exfoliation. Expandable graphite was irradiated in a domestic microwave in full power for 3 min, then soaked in mixed strong hydrogen nitrate and sulfuric acid with volume ratio of 1:1 for 24 h and re-irradiated, thus few-layer graphene sheets were obtained. Specimens gained from every step were selectively characterized by different techniques, such as SEM, XRD, Raman, AFM, XPS, FTIR and combustion elemental analysis. The results show that expandable graphite with loose, porous and worm-like morphology forms instantaneously in microwave irradiation with crackling sound and sparkles, which manifests physical exfoliation of graphene sheets. Few-layer graphene sheets with a dozen or more layers and average thickness of about 4.7 nm are obtained eventually after sequential treatment of microwave irradiation, mixed acid soaking and second microwave irradiation. The as-prepared few-layer graphenes still have high crystallinity and high purity with traces of oxide groups and without serious unrecoverable oxidation damage.
Key words: graphene; expandable graphite; microwave irradiation; strong acid soaking
1 Introduction
Graphene, which was mechanically cleaved from highly oriented pyrolytic graphite, not only challenges the prevalent hypothesis that free standing planar graphene dose not exist, but also excites great interest among researchers worldwide. Numerous applications are based on those superior properties of graphene [1]. With the concentration of charge carriers up to 1013 cm-2 [1], mobilities to 1.5×104 cm2/(V·s) [2] and theoretical specific surface area of 2630 m2/g, graphene-based materials could be applied in sensitive sensors, semiconductors, super-capacitors, transparent conducting thin films (as a supplant of ITO), and micro- electromechanical systems. With elastic modulus as high as 1.01 TPa, studies on graphene may accelerate the process of manufacturing ultra-thin airplanes and bulletproof vests. Due to high thermal conductivity of 5 kW/(m·K) [3], graphene-based composites may take place of silicon chips in microcomputer-processing. Graphene derivatives also show promise in biomedical applications [4,5]. Recently, microwave-assisted exfoliation has emerged as an efficient and versatile method to produce graphene sheets, which enjoys obvious advantages, such as energy transfer instead of heat transfer, quick and volumetric heating, eco-friendliness, higher safety and can increase reaction rate by orders of magnitude compared with traditional heating [6-8]. However, previous researches often deal with graphite oxide and as-prepared products containing relatively high residue of oxygen functional groups with m(C)/m(O)=2.75-14 (measured by combustion elemental analysis) [9-11], which reduces purity, conductivity and exhibition of other superior physical and chemical properties.
In the present study, few-layer graphene with high purity and quality was extracted from expandable graphite sequentially by microwave irradiation, mixed acid soaking and second microwave irradiation, which is facile and energy-saving. Combustion elemental analysis, scanning electron microscopy (SEM), X-ray diffraction (XRD), Raman spectroscopy, X-ray photoelectron spectroscopy (XPS), atomic force microscopy (AFM) and Fourier transform infrared spectroscopy (FTIR) were employed to investigate and characterize the specimens.
2 Experimental
2.1 Raw materials
Graphite powders (G5099) and expandable graphite powders (EG20, EG400) were purchased from Hebei Flame-retardant Materials Company. Acetone (AR), 98% H2SO4 and 69% HNO3 were purchased from Zhuzhou Chemical Reagents Company.
2.2 Preparation of few-layer graphene
G5099, EG20 and EG400 were heated by microwave irradiation (Galanz, G80F23MP-M8SO, 700 W) for 3 min. EG400 displayed the most dramatic volume expansion that resulted in the lightest and most fluffy powders (recorded as MEG400). In order to further exfoliate expanded graphite, 0.1 g of MEG400 was soaked in 100 mL strong mixed acid (V(H2SO4):V(HNO3)=1:1) for 24 h, then washed with deionized water till the percolated solution became neutral. Vacuum filtration was conducted and the grayish filter cake was dried at 60 °C in a blast electric oven to obtain AMEG400 powders. After mixed acid soaking, AMEG400 was treated with second microwave irradiation and the powders gained were recorded as MAMEG400. To directly measure the thickness of the prepared few-layer graphene, MEG400 series powders were dispersed in acetone (0.1 mg/mL) by ultrasonication (KS Instrument, KQ218, 100W) for 10 min and aged for 24 h in normal temperature and pressure. In order to measure the thickness of as- prepared few-layer graphene, dispensions were drop-cast on clear slides and dried in the blast electric oven.
2.3 Characterization
SEM (FEI NOVA, Nano230) was employed to observe the morphology and surface microstructure of exfoliated graphite. Phase analysis was processed by XRD (Rigaku Ltd., Japan, D/max 2550VB, Cu Kα radiation, 40 kV, 250 mA). Molecular vibration behavior was investigated by Raman spectroscopy (HORIBA Scientific, France, Labram Aramis, laser excitation source: λ=532 nm). Thickness measurement was carried out with AFM (VEECO Nano man VS) on as-cast slides. XPS analyzer (K-Alpha 1063, Britain), Thermo Avantage software and FTIR (Nicolet 6700, USA) were employed to check remnant oxide groups and identify the oxidization degree of the few-layer graphene. Combustion elemental analysis was also conducted to analyze the elemental contents with CS600 CS analyzer and TCH600 NHO analyzer (LECO, America).
3 Results and discussion
3.1 SEM images
The microstructures of graphite (G5099) and expandable graphite (EG20 and EG400) before and after microwave irradiation for 3 min are shown in Fig. 1. G5099 (Fig. 1(a)) has clear edges, lamellar structure (seemly 0.3-2.5 μm) and coarse surface with slight delamination. After microwave heating treatment, thinner layers with irregular edges are created, which still firmly adhere to the bulk (Fig. 1(b)). It seems that though graphite is a good microwave absorbent, the energy absorbed from microwave irradiation is not strong enough to break numerous chemical bond interactions that exist between graphite layers. The microstructures of expandable graphite (Figs. 1(c) and (e)) demonstrate blur thicker edges and unevenly-distributed V-shaped clefts, which may be caused by nonuniform intercalation and as-generated violent gas expulsion. SEM images of expanded graphite (Figs. 1(d) and (f)) indicate that large space among the particles, crevice-like pores on the surface and pores in particles [12] facilitate to form hierarchically porous and worm-like microstructure with volume apparently increasing several hundred times. Accordion-like morphology (Figs. 1(d) and (f)) features folded, curve and translucent layers with thickness less than 100 nm [13]. Once the force produced by rapid vaporization of intercalants in layers is larger than interlaminar van der Waals force (about 16.7 kJ/mol), exfoliation along c-axis direction will take place. Hopefully graphene will be obtained by further processing. Among as-listed materials and other graphite derivatives investigated, EG400 obviously enjoys the highest volume expansion in 3-min microwave irradiation, and is chosen to prepare few-layer graphenes.
3.2 XRD patterns
Three XRD patterns (Fig. 2) exhibit some changes in graphite crystal that (002) peaks of MEG400, AMEG400 and MAMEG400 are at 26.25°, 26.23° and 26.17°, respectively, and the corresponding inter-planar distances of d002 are 3.392, 3.395 and 3.402
. These data are a little higher than those of typical graphite (d002=3.354
) and lower than those of the halogen-treated graphite derivatives ((3.47±0.01)
) [14]. Thus, mixed acid soaking and microwave treatment only slightly increase the distance between graphene ab planes, and the specimens retain high crystallographic quality. Without the (001) XRD peak of graphite oxide (2θ≈11°), sp2 hybridization network of the investigated specimens is not irreversibly changed into sp3 defects that may need complicated steps to restore.
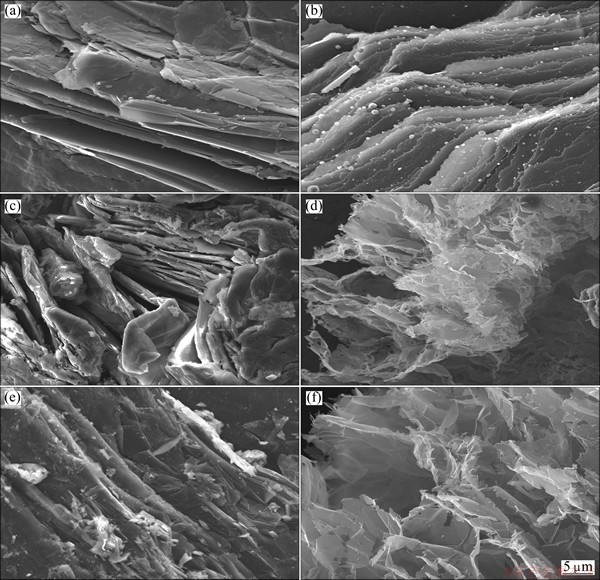
Fig. 1 SEM images of G5099 (a, b), EG20 (c, d) and EG400 (e, f) before (a, c, e) and after (b, d, f) microwave irradiation
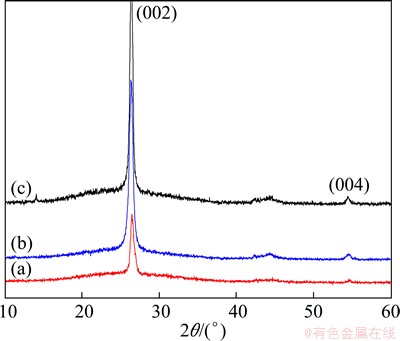
Fig. 2 XRD patterns of MEG400 (a), AMEG400 (b) and MAMEG400 (c)
3.3 Raman spectra
Raman spectroscopy is widely performed to examine carbon derivatives. Figure 3 shows changes in D (1350 cm-1), G (1582 cm-1) and 2D(2700 cm-1) bands of G5099, MEG400, AMEG400 and MAMEG400. Band G corresponds to E2g phonon in Brillouin zone center [15], while all specimens exhibit sharp band G, which means that carbon atoms mainly bond in sp2 hybridization to form a dense-hexagonal structure. AMEG400 (Fig. 3(c)) is obtained by soaking MEG400 (Fig. 3(b)) in the mixed acid, which induces a slightly broader band G and a new band D. Generally speaking, band D is associated with breath vibration mode of sp2 atoms in carbon rings, and implies the reduction in strong sp2 covalent bonds induced by defects, newly emerged edges and sp3 hybridization atoms. In this case, the rise of band D (Fig. 3(c)) may be attributed to the intercalation of sulfate molecules and oxidation reactions that happen on the edges of planar graphene layers. ID/IG ratio was used to evaluate the average size of sp2 domain and the degree of disorder. The suppression of band D in Fig. 3(d) compared with that in Fig. 3(c) suggests that delocalized π-conjugation restores after re-irradiating in microwave oven and the ratio of ID/IG (about 0.14) is lower than that of graphene sheets prepared by chemical reduction approaches [16,17], which indicates high order and low degree of structural defects as a result of efficient deoxygenation of carboxyl, epoxy and other oxygen functional groups. The position, shape and full width half maximum (FWHM) of the second-order 2D band are sensitive to the number of layers in graphene [18-20], which may be triggered by double-resonant effect, in other words, caused by changes in electronic and vibrational properties [21]. Band 2D in Fig. 3(d) is noticeably different from a typical 2D band of graphite (similar to Figs. 3(a)-(c)) which contains two separate fitted bands with intensity about 1/4 IG and 1/2 IG, and also different from the sharp and single 2D band of graphene [22], thus few-layer graphenes are obtained through these procedures.
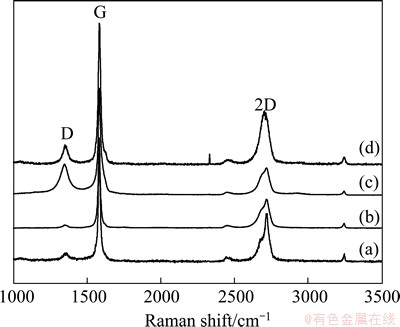
Fig. 3 Raman spectra of G5099 (a), MEG400 (b), AMEG400 (c) and MAMEG400 (d)
3.4 AFM images
Ultrasonic treatment is very popular in preparing graphene nano-materials [23-25]. Here, MEG400, AMEG400 and MAMEG400 were sonicated in acetone (0.1 mg/mL) to form processable dispersions that were drop-cast on glass slides, dried and measured by AFM. MEG400 after sonication (Fig. 4(a)) shows a layer thickness of about 34.5 nm (thinner than that shown in Fig. 1(f)), while the thickness of AMEG400 (Fig. 4(b)) decreases to 19.6 nm, which means the intercalation of sulfate ions or molecules and acoustic cavitation of sonication facilitate further exfoliation of thin graphite sheets. The thickness of MAMEG400 (Fig. 4(c)) is about 4.7 nm, which indicates that few-layer graphene is prepared. Hydrocarbons that exist in atmosphere can be absorbed between few-layer graphenes and glass slide, which may increase the thickness of graphene that is measured. Graphenes on glass substrate also exhibit more fluctuation and rougher surface compared with those on mica substrate [26]. It can be learnt from Fig. 4 that mixed acid soaking and second microwave irradiation can effectively prompt the exfoliation of graphite through sudden formation and expulsion of CO2, H2O and other decomposed products. Ultrasonication can provide localized hot-spots (extreme pressure and temperature) that facilitate the exfoliation. Powerful thrust along c-axis direction surpasses van der Waals force between graphene layers, so, the crumpled worm morphology forms and can be sonicated to few-layer graphene sheets with only a dozen or more layers.
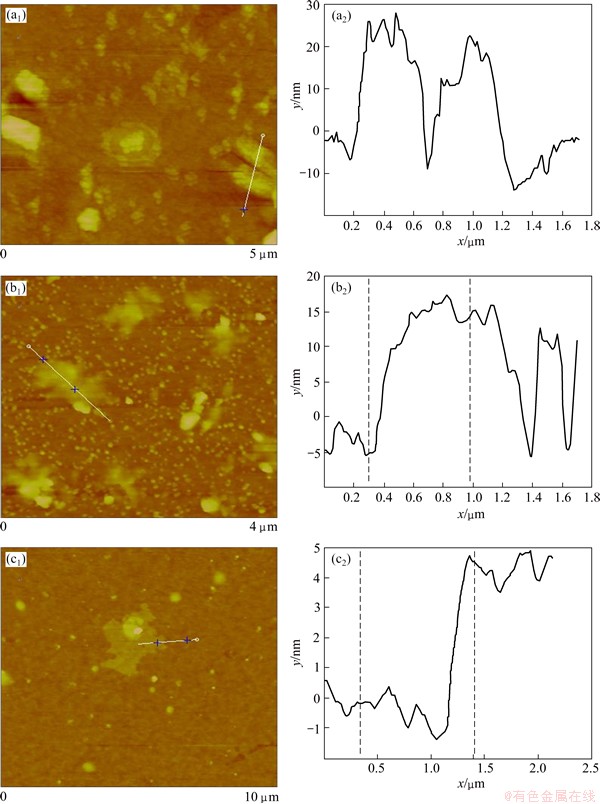
Fig. 4 AFM images of MEG400 (a1, a2), AMEG400 (b1, b2) and MAMEG400 (c1, c2)
3.5 XPS survey analysis
XPS scan was adopted to confirm remnant amount of oxygen functional groups that may impair the conductive network and electrochemically active surfaces for supercapacitor applications. The survey scan plotted in Fig. 5 shows C 1s peak at about 284.78 eV and weak O 1s peak at about 530 eV, which indicates lower oxygen content. The mole fractions of C and O shown in Fig. 5 are similar to graphene nanosheets prepared by SHANMUGHARAJ et al [27] and SRIDHAR et al [28]. The inserted picture in Fig.5 reveals Lorentz fitting of C 1s core level spectrum, which refers to sharp peak at about 284.78 eV (C—C and C=C bonds), minor peak at about 285.78 eV (C—O bond) and 286.98 eV (O—C=O bonds). It is clear that high quality few-layer graphene with low oxygen content is prepared. Noticeable π-π* satellite peak at about 291 eV affirms the restoration of π-π conjugated structure.
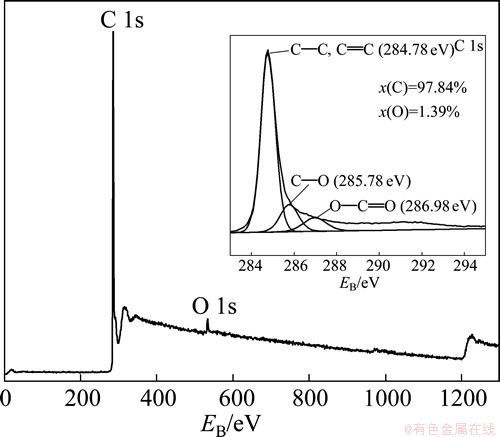
Fig. 5 XPS spectra of MAMEG400
3.6 FTIR analysis
The FTIR spectrum acquired from as-prepared MAMEG400 in Fig. 6 majorly illustrates O—H stretching vibration at 3444 cm-1, carbonyl group (
) and skeletal ring vibrations at 1637 cm-1, and C—O—C groups at 1064 cm-1 according to previous studies [29-31]. The O—H peak is relatively sharp, which contradicts the feature for —COOH and may come from absorbed water. Apparently, Fig. 6 shows weak oxygen functional groups and proves the formation of graphene with high quality, which is consistent with XPS results.
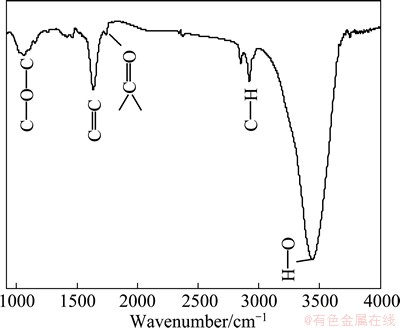
Fig. 6 FTIR spectrum of MAMEG400
3.7 Combustion elemental analysis
As seen in Table 1, the as-prepared few-layer graphene has noticeably high carbon content and only traces of oxygen content, and high purity is obtained during rapid vaporization of intercalants and byproducts in volumetric re-heating where oxide moieties are significantly driven off without using toxic reducing agents. Probably, few-layer graphene with high purity and quality presented here has a bright future in manufacturing of lithium-ion batteries and ultracapacitors.
Table 1 Combustion elemental analysis of MAMEG400 (mass fraction, %)

4 Conclusions
1) Expandable graphite can be rapidly exfoliated in domestic microwave irradiation, which produces accordion-like graphite worm with hierarchical porous morphology. Graphene can be produced by further treatment.
2) Few-layer graphenes were fabricated by first microwave irradiation for 3 min, and then strong mixed acid soaking for 24 h and second microwave irradiation for 3 min.
3) Microwave irradiation is a facile and eco-friendly approach to prepare graphene.
References
[1] NOVOSELOV K S, GEIM A K, MOROZOV S V, JIANG D, ZHANG Y, DUBONOS S V, GRIGOTIEVA I V, GIRSOV A A. Electric field effect in atomically thin carbon films [J]. Science, 2004, 306: 666-669.
[2] CHEN Jian-hao, JANG Chaun, XIAO Shu-dong, ISHIGAMI M, FUHRER M S. Intrinsic and extrinsic performance limits of graphene devices on SiO2 [J]. Nature Nanotechnology, 2008, 3(4): 206-209.
[3] BALANDIN A A, GHOSH S, BAO Wen-zhong, CALIZO I, TEWELDEBRHAN D, MIAO Feng, LAU Chun-ning. Superior thermal conductivity of single-layer graphene [J].Nano Letters, 2008, 8: 902-907.
[4] YANG Xiao-ying, ZHANG Xiao-yan, MA Yan-feng, HUANG Yi, WANG Yin-song, CHEN Yong-sheng. Superparamagnetic graphene oxide–Fe3O4 nanoparticles hybrid for controlled targeted drug carriers [J]. J Mater Chem, 2009, 19(18): 2710-2714.
[5] WANG Zhi-juan, ZHOU Xiao-zhu, ZHANG Juan, BOEY F, ZHANG Hua. Direct electrochemical reduction of single-layer graphene oxide and subsequent functionalization with glucose oxidase [J]. J Phys Chem C, 2009, 113(32): 14071-14074.
[6] MENENDEZ J A, ARENILLAS A, FIDALGO B, FERNANDEZ Y, ZUBIZARRETA L, CALVO E G, BERMUDEZ J M. Microwave heating processes involving carbon materials [J]. Fuel Processing Technology, 2010, 91: 1-8.
[7] HU Hua-ting, WANG Xian-bao, WANG Jing-chao, LIU Fang-ming, ZHANG Min, XU Chun-hui. Microwave-assisted covalent modification of graphene nanosheets with chitosan and its electrorheologiacal characteristics [J]. Applied Surface Science, 2011, 257: 2637-2642.
[8] WEI Tong, FAN Zhuang-jun, LUO Gui-lian, ZHENG Chao, XIE Da-shou. A rapid and efficient method to prepare exfoliated graphite by microwave irradiation [J]. Carbon, 2008, 47: 313-347.
[9] CHEN Wu-feng, YAN Li-feng, BANGAL P R. Preparation of graphene by the rapid and mild thermal reduction of graphene oxide induced by microwaves [J]. Carbon, 2010, 48: 1146-1152.
[10] ZHU Yan-wu, MURALI S, STOLLER M D, VELAMAKANNI A, PINER R D, RUOFF R S. Microwave assisted exfoliation and reduction of graphite oxide for ultracapacitors [J]. Carbon, 2010, 48: 2106-2122.
[11] ZHANG Li-li, ZHAO Xin, STOLLER M D, ZHU Yan-wu, JI Heng-xing, MURALI S, WU Ya-ping, PERALES S, CLEVENGER B, RUOFF R S. Highly conductive and porous acitvated reduced graphene oxide films for high-power supercapacitors [J]. Nano Letters, 2012, 12: 1806-1812.
[12] Michio I, Masahiro T, Kang Fei-yu, Zheng Yong-ping, Shen Wan-ci. Pore structure of exfoliated graphite [J]. New Carbon Material, 2003, 18(4): 241-249.
[13] Stankovich S, Piner R D, Chen Xin-qi, Wu Nian-qiang, Nguyen S T, Ruoff R S. Stable aqueous dispersions of graphitic nanoplatelets via the reduction of exfoliated graphite oxide in the presence of poly (sodium 4-styrenesulfonate) [J]. J Mater Chem, 2006, 16: 155-158.
[14] Makotchenko V G, Grayfer E D, Nazarov A S, Kim S J, Fedorov V E. The synthesis and properties of highly exfoliated graphites from fluorinated graphite intercalation compounds [J]. Carbon, 2011, 49: 3233-3241.
[15] Reina A, Jia Xiao-ting, Ho J, Nezich D, SON H B, Bulovic V, Dresselhaus M S, Kong J. Large area, few-layer graphene films on arbitrary substrates by chemical vapor deposition [J]. Nano Letters, 2009, 9: 30-35.
[16] LIU Chang-qing, HU Guo-xin, GAO Han-yang. Preparation of few-layer and single-layer graphene by exfoliation of expandable graphite in supercritical N, N-dimethylformamide [J]. Journal of Supercritical Fluids, 2012, 63: 99-104.
[17] Gao Wei, Alemany L B, CI Li-jie, Ajayan P M. New insights into the structure and reduction of graphite oxide [J]. Nature Chemistry, 2009, 1: 403-408.
[18] NI Z H, WANG H M, Kasim J, Fan H M, Yu T, Wu Y H, Feng Y P, Shen Z X. Graphene thickness determination using reflection and contrast spectroscopy [J]. Nano Letters, 2007, 7: 2758-2763.
[19] Ferrari A C, Robertson J. Interpretation of Raman spectra of disordered and amorphous carbon [J]. Phys Rev B, 2000, 61(20): 14095-14107.
[20] Ni Zhen-hua, Yu Ting, Lu Yun-hao, Wang Ying-ying, Feng Yuan-ping, Shen Ze-xiang. Uniaxial strain on graphene: Raman spectroscopy study and band-gap opening [J]. ACS Nano, 2008, 2(11): 2301-2305.
[21] Thomsen C, Reich S. Double resonant raman scattering in graphite [J]. Phys Rev Lett, 2000, 85(24): 5214-5217.
[22] Ferrari A C, Meyer J C, Scardaci V, Casiraghi C, Lazzeri M, Mauri F, Piscanec S, Jiang D, Novoselov K S, Roth S, Geim A K. Raman spectrum of graphene and graphene layers [J]. Phys Rev Lett, 2006, 97(18): 187401-4.
[23] Bourlinos A B, Georgakilas V, Zboril R, Steriotis T A, Stubos A K. Liquid-phase exfoliation of graphite towards solubilized graphenes [J]. Small, 2009, 5(16): 1841-1845.
[24] Dhakate S R, Chauhan N, Sharma S, Tawale J, Singh S, Sahare P D, Mathur R B. An approach to produce single and double layer graphene from re-exfoliation of expanded graphite [J]. Carbon, 2011, 49: 1946-1954.
[25] Janowska I, Chizari K, Ersen O, Zafeiratos S, Soubane D, Dosta V D, Speisser V, Boeglin C, HoullE M, BEgin D, Plee D, Ledoux M J, Pham-Huu C. Microwave synthesis of large few-layer graphene sheets in aqueous solution of ammonia [J]. Nano Res, 2010, 3: 126-137.
[26] Lui C H, Liu L, Mak K F, Flynn G W, Heinz T F. Ultraflat graphene [J]. Nature, 2009, 462: 339-341.
[27] Shanmugharaj A M, Choi W S, Lee C W, Ryu S H. Electrochemical performances of graphene nanosheets prepared through microwave radiation [J]. Journal of Power Sources, 2011, 196: 10249-10253.
[28] Sridhar V, Jeon J H, Oh I K. Synthesis of graphene nano-sheets using eco-friendly chemicals and microwave radiation [J]. Carbon, 2010, 48: 2953-2957.
[29] Nethravathi C, Rajamathi M. Chemically modified graphene sheets produced by the solvothermal reduction of colloidal dispersions of graphene oxide [J]. Carbon, 2008, 46: 1994-1998.
[30] LI Xiao-lin, ZHANG Guang-yu, BAI Xue-dong, SUN Xiao-ming, WANG Xin-ran, WANG En-ge, DAI Hong-jie. Highly conducting graphene sheets and Langmuir Blodgett films [J]. Nature Nanotechnology, 2008, 3: 538-542.
[31] SU Ching-yuan, LU Ang-yu, XU Yan-ping, CHEN Fu-rong, KHLOBYSTOV A N, LI Lain-jong. High-quality thin graphene films from fast electrochemical exfoliation [J]. ACS Nano, 2011, 5(3): 2332-2339.
少层石墨烯的微波辐照法快速制备及表征
冷 娴,熊 翔,邹俭鹏
中南大学 粉末冶金国家重点实验室,长沙 410083
摘 要:以可膨胀石墨为原料制备少层石墨烯,通过初次微波辐照3 min、混酸(浓硫酸与浓硝酸的体积比为1:1)浸泡处理24 h及二次微波辐照剥离3 min的工艺流程,得到了少层石墨烯。用扫描电镜(SEM)、X射线衍射(XRD)、拉曼光谱(Raman)、原子力显微镜(AFM)、X射线光电子能谱(XPS)、红外光谱(FTIR)和燃烧法元素分析对实验产物进行表征。结果表明:经过初次微波辐照处理,伴随闪光及爆裂声,可膨胀石墨层片结构被剥离,迅速形成疏松、多孔的蠕虫形貌。通过混酸浸泡、二次微波辐照和超声分散等后续处理,可快速制得厚度约为4.7 nm的约十几层的石墨烯,且石墨烯未被严重氧化,纯度高,结晶度高。
关键词:石墨烯;可膨胀石墨;微波辐照;强酸浸泡
(Edited by Wei-ping CHEN)
Foundation item: Project (51274248) supported by the National Natural Science Foundation of China
Corresponding author: Jian-peng ZOU; Tel: +86-731-88830376; E-mail: zoujp@csu.edu.cn
DOI: 10.1016/S1003-6326(14)63045-4