
Electrochemical behaviors of Mg2+ and B3+ deposition in fluoride molten salts
SHI Zhong-ning, LI Min, LI Lan-lan, GAO Bing-liang, HU Xian-wei, WANG Zhao-wen
School of Materials and Metallurgy, Northeastern University, Shenyang 110004, China
Received 31 August 2010; accepted 19 January 2011
Abstract: By using cyclic and linear sweep voltammetry, the electrochemical deposition behaviors of Mg2+ and B3+ in fluorides molten salts of KF-MgF2 and KF-KBF4 at 880 °C were investigated, respectively. The results show that the electrochemical reduction of Mg2+ is a one-step reaction as Mg2++2e-→Mg in KF-1%MgF2 molten salt, and the electrochemical reduction of B3+ is also a one-step reaction as B3++3e-→B in KF-KBF4 (1%, 2% KBF4) molten salts. Both the cathodic reduction reactions of Mg2+ and B3+ are controlled by diffusion process. The diffusion coefficients of Mg2+ in KF-MgF2 molten salts and B3+ in KF-KBF4 molten salts are 6.8×10-7 cm2/s and 7.85×10-7 cm2/s, respectively. Moreover, the electrochemical synthesis of MgB2 by co-deposition of Mg and B was carried out in the KF-MgF2-KBF4 (molar ratio of 6:1:2) molten salt at 750 °C. The X-ray diffraction analysis indicates that MgB2 can be deposited on graphite cathode in the KF-MgF2-KBF4 molten salt at 750 °C.
Key words: magnesium diboride; electro-deposition; fluoride molten salts; diffusion coefficient
1 Introduction
MgB2, which is a kind of superconductive material with simple structure [1], has attracted much attention in the fundamental physics and engineering application. There are many methods such as pulse laser deposition, chemical vapor deposition (CVD), DC magnetron sputtering, to prepare high quality alkali metal boride [2] and alkaline-earth metal boride [3]. By electro-deposition, magnesium and boron will co-deposit on the cathode, and then react spontaneously with each other by the reaction:
Mg+2B=MgB2 (1)
Recently, YANG et al [4], HIROSHI et al [5] and KENJI and HIDEKI [6] successfully fabricated MgB2 by electro-deposition in chloride-based molten salts. However, the evolving of Cl2 from anode will reduce the concentration of chlorine ion in the electrolyte which consists of B2O3 or MgB2O4 as solute, and make the MgB2 not uniform and costly. If using the fluoride electrolyte with B2O3, MgB2O4 or MgO as solute for MgB2 electrochemical deposition, the anode product is O2 (with inert anode) or CO2 (with carbon anode). Thus the concentration of fluorine ion will keep stable during electro-deposition, which will make the electro- deposition process more easily be controlled.
Although several articles have reported the electrochemical behavior of Mg2+ in ionic liquid [7] and B3+ in the fluoride molten salts [8-9], the electrochemical behaviors of MgB2 in KF-MgF2-KBF4 molten salt systems are not involved. Therefore, the aim of this study is to investigate the reduction mechanism of Mg2+ and B3+ in KF-MgF2 and KF-KBF4 molten salts.
2 Experimental
Electrochemical research was conducted in a three-electrode system with tungsten wire (d0.48 mm, ≥99.95%) as work electrode, platinum wire (d2.4 mm, 99.99%) as reference electrode and graphite rod as counter electrode. A high pure graphite crucible with holding capacity of 70 g electrolyte, which has a specification of 45mm in diameter and 80 mm in depth with a sidewall thinness of 5 mm, was used. The whole apparatus was protected in argon gas. The used chemicals of KF, MgF2 and KBF4 are anhydrous and analytically pure reagents, respectively. Cyclic voltammetry and linear sweep voltammograms were performed with an AUTO Lab 30 potentiostat instrument.
3 Results and discussion
3.1 Electrochemical behavior of Mg2+ in KF-MgF2
Cyclic voltammograms were carried out on a tungsten wire working electrode in a KF-1%MgF2 molten salt system at 880 °C. The cyclic voltammograms under different scanning rates of 0.4, 0.6 and 0.8 V/s are shown in Fig. 1. It is clear that magnesium deposition occurs from -1.7 V (Point A) to -1.9 V (Point B), while potassium deposition occurs from -2.0 V (vs Pt, Point C) to -2.5 V (Point D) versus the quasi-reference electrode. During the reverse sweep, the oxidation peaks of magnesium and potassium run at -2.15 V (Point E) and -1.6 V (Point F), respectively.
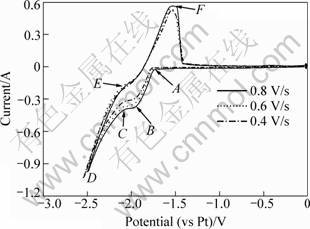
Fig. 1 Cyclic voltammograms in KF-1%MgF2 molten salt system at 880 °C
It is confirmed that the reduction peak at Point B (-1.9 V, vs Pt) is associated with the electrochemical reduction of Mg2+. As seen in Fig. 1, the change in the scanning rate range of 0.4-0.8 V/s does not make the reduction potential shift in the voltammograms. According to Refs. [10-11], the number of exchanged electrons can be deduced from the cyclic voltammograms. For the magnesium reduction peak, the plot of potential φ vs ln[I/(IPC-I)] (IPC is the reduction peak current, and I is the current) is linear, as seen in Fig. 2. From the slope (RT/nF) of linear equation in Fig. 2, the exchanged electron number of Mg2+ reduction is approximately equal to 2 (n≈2). So, it can be concluded that two electrons are exchanged in this reduction. That is to say, the electrochemical reduction of Mg2+ proceeds as follows: Mg2++2e-→Mg. It is a single step for the reaction of Mg2+ reduction.
Furthermore, the relationship between the reduction peak current (IPC) and the square root of the scanning rate (v1/2) is plotted in Fig. 3. From Fig. 3, it is concluded that the reduction peak current (IPC) is linearly related with
the square root of the scanning rate (v1/2). It satisfies the Randles-Sevcik equation completely, which indicates that magnesium electrochemical reduction is a diffusion control process [11]. The diffusion coefficient D can be deduced by the Randles-Sevcik equation as follows [10]:
IPC=-0.4463nFSc(nFD/RT)1/2v1/2 (2)
where S is the electrode area; c is the solute concentration; D is the diffusion coefficient; F is the Farady constant; n is the number of exchanged electrons; v is the scanning rate; T is the thermodynamic temperature.
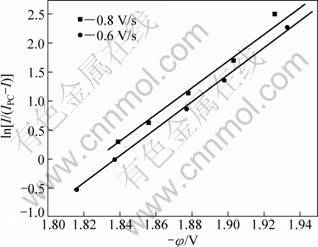
Fig. 2 ln[I/(IPC-I)] as function of potential (φ) in KF-1%MgF2 system
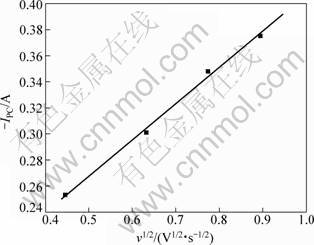
Fig. 3 Plot of peak current (IPC) vs square root of scanning rate (v1/2) in KF-1%MgF2 system at 880 °C
From Fig. 3, we can get the value of slope, which is equal to the value of IPC/v1/2:
IPC/v1/2=-31 mA?s1/2/V1/2 (3)
Taking all parameters (n=2, S=0.226 cm2, c=4.29×10-4 mol/cm3, F=96 487 C/mol, R=8.314 J/(K·mol), T=1 153 K) into Eq. (2), and combining Eqs. (2) and (3), we can obtain the diffusion coefficient of magnesium cation D(Mg2+) under the experiments condition. The calculated D(Mg2+) is 6.8×10-7 cm2/s. This value is less than (7.2-9.9)×10-6 cm2/s in MgCl2-CaCl2-NaCl chloride system [12] and 5.3×10-6 cm2/s in chloride-fluoride MgCl2-MgF2 system [13]. This difference is caused by the different operating temperatures and different molten salt systems.
3.2 Electrode behavior of B3+ in KF-KBF4 molten salts
Figure 4 shows the linear sweep voltammograms of B3+ on tungsten electrode in KF-1%KBF4 and KF- 2%KBF4 molten salts at 880 °C. Only one reduction peak (Point B) in the voltammograms is found, which indicates that the reduction of B3+ occurs in a one-step reaction. It is clear that boron deposition occurs from near -0.8 V (vs Pt, Point A) to -1.25 V (vs Pt, Point B). The reduction peak of boron in the cathode is at -1.25 V (vs Pt, Point B).
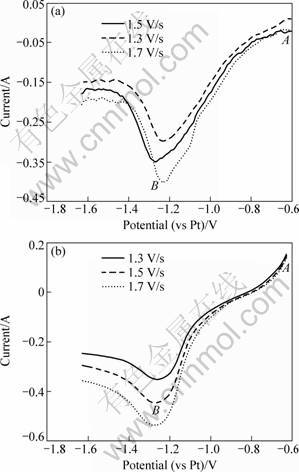
Fig. 4 Liner sweep voltammograms at 880 °C with different scanning rates: (a) KF-1% KBF4; (b) KF-2% KBF4
From Fig. 4, it is observed that the change in the scanning rate does not make the reduction potential shift in the voltammograms. The number of exchanged electrons of boron reduction can be deduced with the same method of Mg2+. The relationship between the potential (φ) and ln[I/(IPC-I)] are plotted in Fig. 5. By calculating the slope (RT/nF) of these linear equations, the average number of exchanged electrons for B3+ reduction reaction (existing as BF4- complex anion actually [14]) is approximately equal to 3. Therefore, it can be concluded that three electrons are exchanged in this reduction. That is to say, the electrochemical reduction of B3+ proceeds as follows: B3++3e-→B.
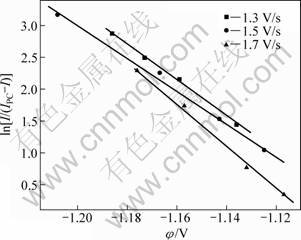
Fig. 5 ln[I/(IPC-I)] as function of potential (φ) in KF-KBF4 system
Moreover, the relationship between the reduction peak current (IPC) and the square root of the scanning rate(v1/2) is also plotted, as shown in Fig. 6.
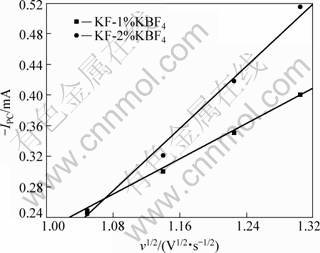
Fig. 6 Plot of peak current (IPc) vs square root of scanning rate (v1/2) in KF-KBF4 system at 880 °C
From Fig. 6, we can conclude that the B3+ reduction peak current (IPC) is also linearly related with the square root of scanning rate (v1/2) like Mg2+. So, the electrochemical reduction process is also controlled by the diffusion of boron cation in the solution. The value of IPC/v1/2 is as follows.
1) In KF-1%KBF4 molten salt,
IPC/v1/2=-61.03 mA?s1/2/V1/2 (4)
2) In KF-2%KBF4 molten salt,
IPC/v1/2=-118.40 mA?s1/2/V1/2 (5)
Taking all the parameters into Eq. (2), the diffusion coefficient of boron cation at 880 °C can be calculated as follows:
1) In KF-1%KBF4 molten salt, D(B3+)=7.9×10-7 cm2/s;
2) In KF-2%KBF4 molten salt: D(B3+)=7.8×10-7 cm2/s.
These diffusion coefficient values are more than 4.5×10-10 cm2/s on steel cathode at 800 °C and 1.13×10-10 cm2/s on molybdenum cathode at 830 °C in KF-LiF-KBF4 molten fluorides [15-16]. The difference is caused by the different substrates, temperatures and molten salt systems.
The concentration of B3+ does not much affect the diffusion coefficient of B3+. Therefore, the corresponding diffusion coefficient of B3+ can be treated using an average value as 7.85×10-7 cm2/s.
3.3 Electro-deposition of MgB2 in KF-MgF2-KBF4 system
In the above experiments, the operating temperatures were 880 °C in both KF-1%MgF2 and KF-1%KBF4 molten salts system. However, in an eutectic MgF2-KF-KBF4 system, the electro-deposition could be carried out near 750 °C, which is lower than the melting point of MgB2 (800 °C). According to the Gibbs free energy of Eq. (1), the value of ?GΘ1023K is significantly negative, so MgB2 coating could be deposited at a suitable deposition potential.
An electro-deposition of MgB2 experiment was conducted in a close system of argon environment at 750 °C. The electrolyte consisted of MgF2, KF and KBF4 with a molar ratio of 1:6:2. The graphite crucible (d75 mm×80 mm, sidewall thickness of 7.5 mm) acted as an anode, and the cathode was high cylinder pure graphite (d10 mm×50 mm). The current density was 0.35 A/cm2. At the beginning of the electro-deposition process, pre- electrolysis was performed for 10 min.
During the course of electro-deposition, the cell voltage fluctuated in a range of 3.6-3.9 V, as seen in Fig. 7. After experiment, the cathode surface was analyzed with XRD, and MgB2 was found, as seen in Fig. 8.
According to the ionic structure of KF-MgF2-KBF4 molten slat, the electrode reactions are given as follows:
Anodic reaction,
8F--8e-=4F2 (6)
Cathodic reaction,
Mg2++2B3++8e-=MgB2 (7)
The overall reaction is
MgF2+2KBF4=2KF+4F2+MgB2 (8)
Using graphitic anode, the anodic gas of fluorine will react with carbon at 750 °C:
2F2+C=CF4 (9)
3F2+2C=C2F6 (10)
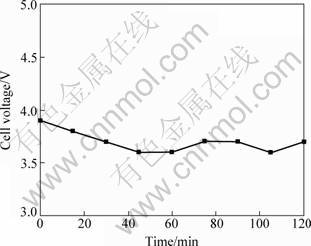
Fig. 7 Relationship between cell voltage and time during MgB2 electrochemical deposition
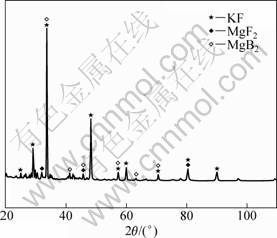
Fig. 8 XRD pattern of electro deposited product from KF-MgF2-KBF4 system
Therefore, the following expression could describe this process:
MgF2+KBF4+C→F2+CF4 (or C2F6)+KF+MgB2 (11)
When using oxides MgO and B2O3, CO2 (with carbon anode) or oxygen (with inert anode) could be evolved, which is similar to the Hall-Heroult aluminium reduction process [15]. This will provide an environmental friendly, low cost and high efficiency process for MgB2 synthesis.
4 Conclusions
1) Both electrochemical reduction processes of Mg2+ in the KF-MgF2 and B3+ in the KF-KBF4 molten salts are governed by the diffusion process. The diffusion coefficient of Mg2+ in KF-1%MgF2 is 6.8×10-7 cm2/s, while the diffusion coefficient of B3+ in KF-KBF4 is 7.85×10-7 cm2/s at 880 °C.
2) The deposition processes of Mg2+ in the KF-MgF2 molten salt and B3+ in the KF-KBF4 molten salt are one-step reaction process at 880 °C.
3) MgB2 could be fabricated by electro-deposition on graphite electrode in the KF-MgF2-KBF4 molten salt at 750 °C. The overall reaction may be: MgF2+KBF4+ C→F2+CF4(or C2F6)+KF+MgB2.
References
[1] NAGAMATSU J. Super conductivity at 39 K in magnesium diboride [J]. Nature, 2001, 410: 63-66.
[2] LIU Zhi-jian, YIN Jian, MENG Zhen-qiang, CHONG Jin, ZHOU De-bi. Electrochemical behavior and morphology of LiB compound anode materials[J]. Transactions of Nonferrous Metals Society of China, 2006,16(1): 127-131.
[3] SHIMAKAGE H, SAITO A, KAWAKAMI A, WANG Z. Fabrication condition dependence of as-grown MgB2 thin films by co-evaporation method [J]. Physica C, 2004, 408-410: 891-893.
[4] YANG H Z, SUN X G, HUANG W Q, LI M L, YU X M, ZHANG B S, QI Y, ZHAO Q. Properties of MgB2 films fabricated on copper cathodes by electrochemical technique [J]. Acta Metallurgica Sinica, 2008, 21(5): 351-354.
[5] HIROSHI S, TAKASHI K, YUDAI F, HIROMI O, TADASHI K, KAZUSHI H. Synthesis of MgB2 film by electrochemical process [J]. Physica C, 2008, 468: 1097-1099.
[6] KENJI Y, HIDEKI A. Electrochemical synthesis of superconductive MgB2 from molten salts[J]. Physica C, 2003, 388: 113-114.
[7] AMIR N, VESTFRID Y, CHUSID O, GOFER Y, AURBACH D. Progress in nonaqueous magnesium electrochemistry[J]. J Power Sources, 2007, 174: 1234-1240.
[8] LI Jun, LI Bing. Electrochemical reduction and electrocrystallization process of B(III) in the LiF-NaF-KF-KBF4 molten salt[J]. Rare Metals, 2007, 26(1): 74-78.
[9] SHI Qing-rong, DUAN Shu-zhen, WU Shi-min. Electrochemical reduction of B(III) in molten fluorides [J]. Journal of University of Science and Technology Beijing, 1994, 16(6): 599-603. (in Chinese)
[10] BARD A J, FAULKNER R L. Electrochemical methods fundamentals and applications [M]. SHAO Y S, ZHU K Y, DONG X D, ZHANG B L, transl. Beijing: Chemical Industry Press, 2008: 159. (in Chinese)
[11] MASSOT L, CHAMELOT P, BOUYER F, TAXIL P. Electrodeposition of carbon films from molten alkaline fluoride media [J]. Electrochimica Acta, 2002, 47: 1949-1957.
[12] CASTRILLEJO Y, MARTINEZ A M, PARDO R, HAARBERGB G M. Electrochemical behaviour of magnesium ions in the equimolar CaCl2-NaCl mixture at 550 °C[J]. Electrochimica Acta, 1997, 42(12): 1869-1876.
[13] BORRESEN B, HAARBER G M, TUNOLD R. Electrodeposition of magnesium from halide melts-charge transfer and diffusion kinetics [J]. Electrochimica Acta, 1997, 42(10): 1613-1622.
[14] GRJOTHEIM K, KROHN C, MALINOVSKY M, MATIASOVSKY K, THONSTAD J. Aluminium electrolysis—Fundamentals of the Hall-Héroult process [M]. 2nd ed. Düsseldorf: Aluminium-Verlag, 1982: 339.
[15] BONOMI A, HABERSAAT R, BIENVENU G. Electrochemical boriding of nitriding steel in molten salts [J]. Surface Technology, 1978, 6: 313-319.
[16] BONOMI A, GIESS H, GENTAZ C. Electrochemical boriding of molybdenum in molten salts [J]. Electrodeposition Surface Treatment, 1972, 73: 419-427.
氟化物熔盐体系中Mg2+和B3+的电化学沉积行为
石忠宁,李 敏,李兰兰,高炳亮,胡宪伟,王兆文
东北大学 材料与冶金学院,沈阳 110004
摘 要:采用循环伏安和线性扫描伏安技术,研究在880 °C下Mg2+和B3+分别在KF-MgF2和KF-KBF4氟化物熔盐体系中的电化学沉积行为。结果表明:Mg2+在KF-1%MgF2熔盐体系中的还原是一步两电子反应,Mg2++2e-→Mg;B3+在KF-KBF4熔盐体系中的还原是一步三电子反应,B3++3e-→B。Mg2+和B3+的还原过程受扩散控制,Mg2+在KF-MgF2 体系中的扩散系数为6.8×10-7 cm2/s,B3+在KF-KBF4熔盐体系中的扩散系数为7.85×10-7 cm2/s。在温度为750 °C、摩尔比为6:1:2的KF-MgF2-KBF4熔盐体系中进行电沉积制备MgB2。X射线衍射分析结果表明,在石墨阴极的表面得到了MgB2。
关键词:二硼化镁;电沉积;氟化物熔盐;扩散系数
(Edited by YANG Hua)
Foundation item: Project (50804010) supported by the National Natural Science Foundation of China; Project (2007CB210305) supported by the National Basic Research Program of China
Corresponding author: SHI Zhong-ning; Tel: +86-24-83686464; E-mail: znshi@163.com
DOI: 10.1016/S1003-6326(11)60910-2