J. Cent. South Univ. (2016) 23: 3079-3084
DOI: 10.1007/s11771-016-3372-x

Effects of MgO content and CaO/Al2O3 ratio on surface tension of calcium aluminate refining slag
XU Ji-fang(许继芳)1, ZHANG Jie-yu(张捷宇)2, CHEN Dong(陈栋)1,
SHENG Min-qi(盛敏奇)1, WENG Wen-ping(翁文凭)1
1. Shagang School of Iron and Steel, Soochow University, Suzhou 215021, China;
2. State Key Laboratory of Advanced Special Steel, Shanghai University, Shanghai 200072, China
Central South University Press and Springer-Verlag Berlin Heidelberg 2016
Abstract: Surface tension of calcium aluminate refining slag was measured by the Slide method at 1823 K. Based on different levels of the MgO content and the mass ratio of CaO to Al2O3, the effects of MgO content and the mass ratio of CaO to Al2O3 on surface tension were investigated. The results indicate that surface tension decreased with increasing MgO content (from 0 to 4.86%), followed by an increase with further increasing MgO content up to 11.33%. The trend that surface tension changed with the mass ratio of CaO to Al2O3 was the same as the trend that surface tension changed with the MgO content. The surface tension was varied from 0.617 N/m to 0.710 N/m, for the mass ratio of CaO to Al2O3 varying between 0.60 and 1.28. An attempt was made to estimate surface tension of CaO-Al2O3-MgO slag and its sub-system, and the application showed that the model worked well.
1 Introduction
It is of importance to investigate surface tension of slag not only to the theory research for molten melt, which is directly amenable to the structure correlations of molten melt, but also to the application for processes. Many metallurgy phenomena are closely related to surface tension of slag. It may directly affect the behavior of bubbles or drops in gas-liquid reaction systems of materials and metallurgy processing, therefore, influencing the rates of the relevant processes. CaO-Al2O3 system is of considerable importance in metallurgical processes, such as LF (Ladle furnace) and ESR (Electroslag remelting), ceramic materials and geological phenomena. The binary phase diagram shows that the eutectic composition (about 50% CaO and 50% Al2O3, near to 12CaO·7Al2O3 composition) has the lowest temperature on the liquidus surface [1]. However, the MgO component is introduced into the slag inevitably because metallurgical flux and refractory contain a small amount of MgO. Therefore, as a matter of fact, the calcium aluminate refining slag containing magnesia is usually used during metallurgical process. Many researchers reported optimization of CaO-Al2O3- MgO system [2-5]. The temperature of calcium aluminate slag was lower than 1823 K when the addition of MgO into 12CaO·7Al2O3 was less than 10%.
As we know, surface tension of liquid CaO-Al2O3 slag has been investigated experimentally, and few experimental studies have been reported on CaO-Al2O3- MgO system, most of which investigated the effect of MgO content on surface tension with the mass ratio of CaO to Al2O3 about 1.0 and 1.2 [6]. However, the currently available surface tension data are often felt too few to fulfill the requirements either in conventional or in advanced metallurgy processing. Furthermore, the available measured surface tension data of a system under the same or similar experimental conditions are often scattered. Many works are needed for further research.
The main interest of this work is on the 12CaO·7Al2O3 refining slag with addition of MgO. In our previous work, the density and viscosity of selected CaO-Al2O3-MgO system were investigated, and effects of the MgO content and the mass ratio of CaO to Al2O3 were studied. The results showed that both of the MgO content and the mass ratio of CaO to Al2O3 had a significant influence on density and viscosity of slag [7-8]. The aim of the present work is to investigate effects of the MgO content and the mass ratio of CaO to Al2O3 on surface tension of slag. On the basis of the present experimental data and the data from literature, an attempt was made to estimate surface tension of CaO-Al2O3-MgO slag and its sub-system by the so-called excess surface tension model.
2 Experimental
These samples were prepared from CP grade purity CaO (≥99.0%), Al2O3 (≥99.5%) and MgO (≥99.0%) powders, which were dried at 373 K for 24 h. The slag in the experiments was prepared in the laboratory and the method of preparation has been reported elsewhere [9]. As shown in Table 1, the desired samples were divided into two groups. One was that the compositions of samples were on the line of the mass ratio of CaO to Al2O3 equal to 1.0, in which the MgO content was varied from 0% to 12%. The other group is that the compositions of slag samples were on the line of MgO content equal to 5.5%, in which the mass ratio of CaO to Al2O3 was varied from 0.60 to 1.20. Table 1 also gives the analyzed chemical compositions of the homogeneous samples, which were melted in air induction furnace and then crushed into fine powders.
The surface tension was measured using the High-temperature physical function determinator (RTW-08, China) by the Slide method, which involves measuring the maximum excess force exerted on a cylinder during its withdrawal from immersion in the melt [10]. Schematic of experimental apparatus is shown in Fig. 1. The measurement has a maximum continuous temperature of 1873 K, which was measured by a Pt-30Rh/Pt-6Rh thermocouple touching the crucible bottom from outside. The metal pin for surface tension measurement was made of molybdenum, and the size of pin had to be carefully designed so that was is applicable in the required measurement range. As shown in Fig. 2, the dimension of the pin at high temperature was calculated from those measured at room temperature and coefficient of thermal expansion of molybdenum. Knowledge of the maximum force and the dimensions of the cylinder allow the surface tension of the melt to be determined as follows:
(1)
where Fmax is the maximum excess force required for detachment of the pin from the surface of the melt; R is the average of the inner and outer radii of the pin; r is half the wall thickness of the pin; V is the volume of liquid suspended above the free surface of the melt; and f (R3/V, R/r) is a correction factor to account for the noncylindrical shape of the suspended liquid, which was measured by pure water several times until the measured values are stable at room temperature and coefficient of thermal expansion of molybdenum.
For each sample, the graphite crucible, filled with 120 g fine slag power, was placed in the furnace, and the furnace was programmed to heat up to 1823 K with heating rate of 10 K/min. The furnace was kept at the temperature at least for 30 min before slag was melted completely. Then, the surface tension was measured until the measured values were stable, with the error of ±1×10-3 g. After measurement was complete, the furnace was cooled down.
In surface tension measurements, several sources of error may occur. As mentioned above, a deviation from the volume of pin should cause an error in surface tension; the determination of the temperature with an accuracy of ±5 K should introduce an error in surface tension; the accuracy of ±1×10-3 g in the weighting balance should also cause an additional error in surface tension. The total error in the determination of the surface tension was at least ±2.5%.
Table 1 Nominal and analyzed chemical compositions of slag samples
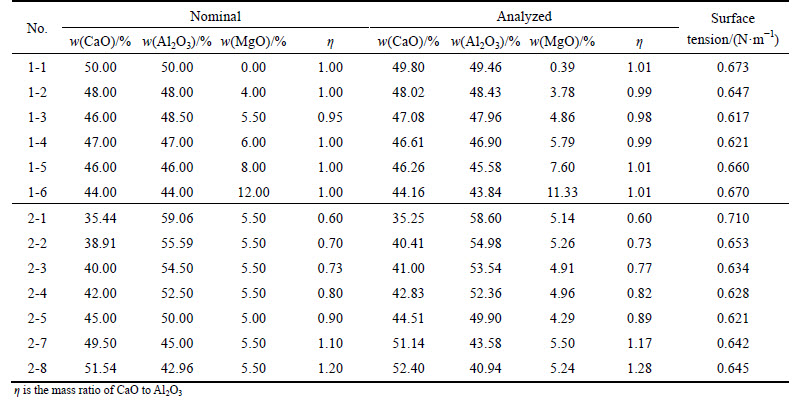
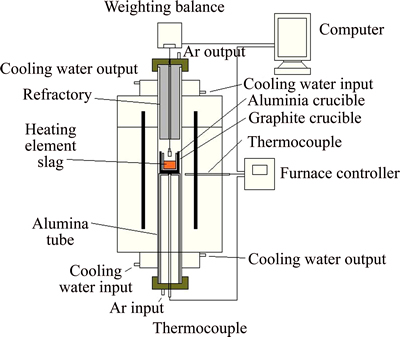
Fig. 1 Schematic of experimental apparatus
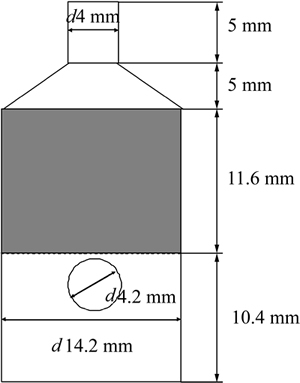
Fig. 2 Dimension of pin (Unit: mm)
3 Results and discussion
3.1 Effect of MgO content on surface tension of selected slag
Figure 3 presents relationship between the surface tension and the MgO content at 1823 K. It is shown that the MgO content had obvious influence on surface tension of selected slag. At the same mass ratio of CaO to Al2O3, surface tension decreased with the increasing MgO content at first, and then increased as the MgO content continued to increase. The results showed that there was a minimum surface tension value approximately 0.617 N/m when the MgO content was 4.86%. The comparison of the experimental results with the data in Ref. [6] is also shown in Fig. 3. At the composition range of the present study, no previously measured surface tension values were found in literature. However, surface tension of CaO-Al2O3 slag has been studied at 1773, 1873 and 1973 K [6]. Comparison of corresponding slags reveals that the value of the present study is close to the results from literature.
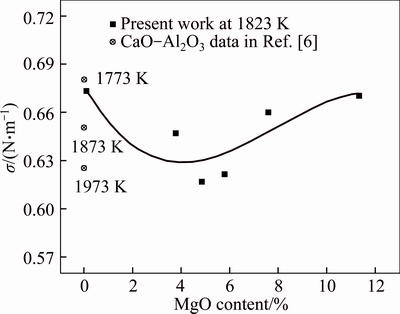
Fig. 3 Relationship between surface tension and MgO content
In general, on the basis of the additive method widely used for alloys and slags, surface tension of slag depends upon surface tension of oxide [11]. The pure component surface tensions of CaO, MgO, and Al2O3 are 0.621, 0.611 and 0.701 N/m at 1823 K, respectively [12]. Since the pure component surface tension of MgO and CaO are much lower than the pure component surface tension of Al2O3, the surface tension of slag will decrease with the small amount of increasing MgO content. On the other hand, surface tension is also closely related to the total number of ions and moment among the ions on the surface of the unit, and the variation of surface tension mainly depends on the force between O2- in the surface and nearby cations [13-14]. In molten slag, the gradual addition of cations results in the progressive breaking of these oxygen bonds with the formation of non-bridging oxygen (NBO). The tendency to form more extreme anionic units can be ranked in terms of the parameter in the hierarchy Mg2+>Ca2+. With the further addition of MgO, instead of CaO and Al2O3 in 12CaO·7Al2O3, should reduce the degree of polymerization, and the amount of free O2- in molten slag, which plays a more significant role in changing surface tension than the cations and the complex anions, will increase, which should lead to raise surface tension of slag [10]. Consequently, surface tension of slag is enhanced with the further increasing of the MgO content.
3.2 Effect of mass ratio of CaO to Al2O3 on surface tension of selected slag
The relationship between surface tension and the mass ratio of CaO to Al2O3 at 1823 K is shown in Fig. 4. The result indicated that the mass ratio of CaO to Al2O3 also had obvious influence on surface tension of selected slags. The trend that surface tension changed with the mass ratio of CaO to Al2O3 was the same as the trend that surface tension changed with the MgO content. Surface tension was varied from 0.617 N/m to 0.710 N/m, for the mass ratio of CaO to Al2O3 varying between 0.60 and 1.28. The data of corresponding slags of CaO-Al2O3 system and CaO-MgO-Al2O3 system in Ref. [6] are also shown in Fig. 4. Comparison of corresponding slags in CaO-Al2O3 binary shows that the value of the present study is close to the most of the literature data. The trend of surface tension varying with the mass ratio of CaO to Al2O3 in the present work is the same as that of the literature at elevated temperature, which also confirmed the repeatability of the present experiment. Comparison of corresponding slags in CaO-MgO-Al2O3 system indicates that the values of the present study are higher than the experimental ones in literature. The difference could be involved in slag composition and experimental procedure.
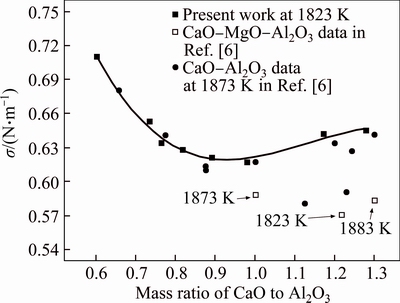
Fig. 4 Relationship between surface tension and mass ratio of CaO to Al2O3
Since the pure component surface tension of Al2O3 is larger than that of the other pure components mentioned above, surface tension will also decrease with increasing the mass ratio of CaO to Al2O3. Slags mainly are composed of the cations and complex anions and the amount of ions and the attraction force between ions directly affect the slag surface tension [15-16]. With increasing the mass ratio of CaO to Al2O3, the network-breaking cations (e.g. Ca2+, Mg2+) present in slag increase, and the complex polymers of aluminum complicated structures as AlO45- tetrahedral break down into smaller units, reducing the degree of polymerization; the amount of free O2- of molten slag gets more and more with raising the mass ratio of CaO to Al2O3, which makes surface tension increase. Furthermore, the molar masses of CaO, MgO and Al2O3 are 56.08 g/mol, 40.31 g/mol and 101.96 g/mol, respectively. Since the molar mass of Al2O3 is larger than that of other metal oxide components, the total number of ions in molten slag will also increase with increasing the mass ratio of CaO to Al2O3. The lower degree of polymerization and the higher number of ions in molten slag will enhance the attraction forces between ions, and surface tension of slag will increase.
4 Estimated surface tension of CaO-MgO- Al2O3 slag
Due to the inherent problems and difficulties associated with measurements at high temperature, it is necessary to have access to reliable model for estimating surface tension of slag. There are many kinds of models [12, 17-22] for estimating surface tension of slag, most of which are proposed based on the structure of atoms and molecules with physical picture of the practical solution, or the model combines both theoretical considerations and practical thermodynamic. Based on the regular solution approximation rules of excess molar quantities for binary system melt, the expanded approximation rules was proposed to estimate molar volume for multi-component slag [23]. In the present work, this idea was used to estimate surface tension for CaO-Al2O3-MgO slag. The surface tension of slags should be expressed as follows:
(2)
and
(3)
where xi and σi respectively indicate the mole fraction and surface tension for component i; σI and σE represent the contributions of the ideal and non-ideal behaviors of the solution, respectively; σE is defined as the excess surface tension. We do it to obtain the relation between the excess surface tension and composition. It is well known that the regular solution approximation rule is most widely used. The excess surface tension of slags can be expressed as
(4)
where aij represents the parameters for components i and j, which could be obtained by optimizing experimental data in certain composition and temperature range; aijk represents the parameters for components i, j and k; xi, xj and xk indicate the mole fractions of component i, j and k, respectively.
The average error of all calculated values can be assessed by Eq. (5)-(6). △n is the percentage difference of the calculated and measured values; △ value is calculated by taking the summation of all absolute values of △n and dividing by the number of all data.
(5)
(6)
The surface tension of the pure oxides used in the present model is listed in Table 2, recommended by MILLS and KEENE [17]. The necessary data have been collected using the present experimental data and the data from Ref. [6] in CaO-MgO-Al2O3 system and CaO-Al2O3 sub-system, and the present model was used to estimate surface tension of slag. The optimized parameters are shown in Table 3. As shown in Fig. 5, the estimated values using the present model are compared with experimental data verify the model. The result indicated that the estimated values agree with the experimental data, and for all calculated values, the average error △ is 4.33%. It can be seen that the model prediction can reach a reasonable accuracy as an appropriate amount of experimental data is available.
Table 2 Surface tension of pure components [17]
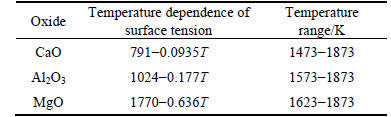
Table 3 Values of model parameters for selected slags
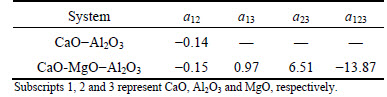
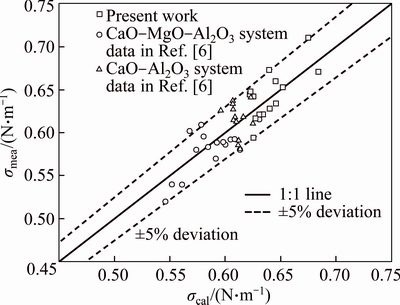
Fig. 5 Comparison between experimental data and predicated
5 Conclusions
Surface tension of calcium aluminate refining slag was measured by the Slide method at 1823 K. Different slag compositions were chosen based on different levels of the MgO content and the mass ratio of CaO to Al2O3, and effects of the MgO content and the mass ratio of CaO/Al2O3 were investigated. The main features are concluded as follows:
1) Both of the MgO content and the mass ratio of CaO to Al2O3 had influence on surface tension of calcium aluminate refining slag. The surface tension decreased with increasing MgO content, followed by an increase with further increasing MgO content.
2) The trend that surface tension changed with the mass ratio of CaO to Al2O3 was the same as the trend that surface tension changed with the MgO content. Surface tension was varied from 0.617 N/m to 0.710 N/m, for the mass ratio of CaO to Al2O3 varying between 0.60 and 1.28.
3) On the basis of the regular solution approximation rules, an attempt was made to estimate surface tension of CaO-Al2O3-MgO slag and its sub- system. The result indicated that the present model could fit the experimental data well.
References
[1] HALLSTEDT B. Assessment of the CaO-Al2O3 system [J]. J Am Ceram Soc, 1990, 73(1): 15-23.
[2] HALLSTEDT B. Thermodynamic assessment of the CaO-MgO- Al2O3 system [J]. J Am Ceram Soc, 1995, 78(1): 193-198.
[3] de AZA A H, PENA P, de AZA S. Ternary system Al2O3-CaO- MgO: Ι, primary phase field of crystallization of spinel in the subsystem MgAl2O4-CaAl4O7-CaO-MgO [J]. J Am Ceram Soc, 1999, 82(8): 2193-2203.
[4] de AZA A H, IGLESIAS J E, PENA P, de AZA S. Ternary system Al2O3-CaO-MgO: Part ΙΙ, phase relationships in the subsystem Al2O3-MgAl2O4-CaAl4O7 [J]. J Am Ceram Soc, 2000, 83(4): 919-927.
[5] JUNG I H, DEGTEROV S A, PELTON A D. Thermodynamic evaluation and optimization of the MgO-Al2O3, CaO-MgO-Al2O3 and MgO-Al2O3-SiO2 systems [J]. Journal of Phase Equilibria and Diffusion, 2004, 25(4): 329-345.
[6] Verein Deutscher Eisenhuttenleute. Slag atlas [M]. 2nd ed. Düsseldorf: Verlag Stahleisen, 1995.
[7] XU Ji-fang, WAN Kang, ZHANG Jie-yu, CHEN Yao, SHENG Min-qi. Measuring and modelling of density for selected CaO-Al2O3-MgO slags [J]. Journal of the Southern African Institute of Mining and Metallurgy, 2015, 115(8): 775-780.
[8] XU Ji-fang, ZHANG Jie-yu, JIE Chang, RUAN Fei, CHOU Kuo-chih. Experimental measurements and modeling of viscosity in the CaO-Al2O3-MgO slag system [J]. Ironmaking and Steelmaking, 2011, 38(5): 329-337.
[9] XU Ji-fang, ZHANG Jie-yu, JIE Chang, TANG Lei, CHOU Kuo-chih. Measuring and modeling of density for selected CaO-MgO-Al2O3-SiO2 slag with low silica [J]. J Iron Steel Res Int, 2012, 19(7): 26-32.
[10] YANG Li-li, WANG Hong-ming, ZHU Xiang, LI Gui-rong. Effect of boron mud and CaF2 on surface tension and density of CaO-SiO2-B2O3 ternary slag systems [J]. J Iron Steel Res Int, 2014, 21(8): 745-748.
[11] MAO Yu-wen. Metallurgical melt [M]. Beijing: Metallurgical Industry Press, 1994. (in Chinese)
[12] HANAO M, TANAKA T, KAWAMOTO M, TAKATANI K. Evaluation of surface tension of molten slag in multi-component systems [J]. ISIJ Int, 2007, 47(7): 935-939.
[13] MILLS K C. The influence of structure on the physico-chemical properties of slags [J]. ISIJ Int, 1993, 33(1): 148-155.
[14] DONG Yan-wu, JIANG Zhou-hua, CAO Yu-long, ZHANG Hong-kui, SHEN Hai-jun. Effect of MgO and SiO2 on surface tension of fluoride containing slag [J]. Journal of Central South University, 2014, 21(11): 4104-4108
[15] HAEA S, OGINO K. The density and the surface tensions of fluoride melts [J]. ISIJ Int, 1989, 29(6): 477-485.
[16] CUI Chuan-meng, XU Xiu-guang, ZHANG Xian-peng, WANG Kui-han, HANG Wei-nu. Effect of composition of B2O3-MgO-SiO2- Al2O3-CaO slag system on physical properties of melt [J]. Acta Metallurgica Sinica, 1996, 32(6): 637-641. (in Chinese)
[17] MILLS K C, KEENE B J. Physical properties of BOS slags [J]. International Materials Reviews, 1987, 32(1/2): 1-120.
[18] QIAO Zhi-ya, YAN Li-jun, CAO, Zhan-min, XIE Yu-nan. Surface tension prediction of high-temperature melts [J]. Journal of Alloys and Compounds, 2001, 325(1/2): 180-189.
[19] CHENG Guo-guang, LIAO Neng-bin. Calculation model for surface tension of slag melt [J]. J Iron Steel Res Int, 1999, 6(2): 17-20.
[20] AUNE R, HAYASHI M, NAKAJIMA K, SEETHARAMAN S. Thermophysical properties of silicate slags [J]. JOM, 2002, 54(11): 62-69.
[21] ZHANG Jia-yun, SHU Qi-feng, WEI Shou-kun. Prediction of surface tension in molten metallic and ionic systems [J]. High Temp Mat Pr-ISR, 2003, 22(5/6): 395-400.
[22] CHOU Kuo-chih, ZHANG Guo-hua. Calculation of physicochemical properties with limited discrete data in multicomponent systems [J]. Metallurgical and Materials Transactions B, 2009, 40(2): 223-232.
[23] NAKAJIMA K. Estimation of molar volume for multicomponent silicate melts [J]. Tetsu-to-Hagane, 1994, 80(8): 593-598.
(Edited by YANG Hua)
Foundation item: Project(51204115) supported by the National Natural Science Foundation of China; Project(BK20130308) supported by the Basic Research Program of Jiangsu Province, China; Project(2014M561710) supported by China Postdoctoral Science Foundation
Received date: 2015-08-27; Accepted date: 2016-05-23
Corresponding author: XU Ji-fang, Associate Professor, PhD; Tel: +86-512-67164815; E mail: xujifangsuda@163.com