Trans. Nonferrous Met. Soc. China 25(2015) 4001-4007
Ag-Ni alloy nanoparticles for electrocatalytic reduction of benzyl chloride
Hai-hui ZHOU1,2, Yan-ling LI1,2, Jia-qi HUANG1,2, Chen-xu FANG1,2, Dan SHAN1,2, Ya-fei KUANG1,2
1. State Key Laboratory for Chemo/Biosensing and Chemometrics, Hunan University, Changsha 410082, China;
2. College of Chemistry and Chemical Engineering, Hunan University, Changsha 410082, China
Received 9 December 2014; accepted 15 July 2015
Abstract: Ag-based nanocatalysts exhibit good catalytic activity for the electrochemical reduction of organic halides. Ag-Ni alloy nanoparticles (NPs) were facilely prepared by chemical reduction, and the as-prepared nanocatalysts were characterized by X-ray diffraction, ultraviolet-visible spectroscopy, transmission electron microscopy and energy-dispersive X-ray spectroscopy. The electrocatalytic activity of Ag-Ni NPs for benzyl chloride reduction was studied in organic medium using cyclic voltammetry, chronoamperometry and electrochemical impedance spectroscopy. The results show that the addition of Ni element can obviously decrease the size of Ag-Ni NPs, shift the reduction peak potential (φp) of benzyl chloride positively, and increase the catalytic activity of Ag-Ni NPs. However, when the Ni content reaches a certain value, the catalytic activity of Ag-Ni NPs decreases. Meanwhile, the synergistic catalytic effect of Ag-Ni NPs was also discussed.
Key words: Ag-Ni nanoparticles; benzyl chloride; synergistic catalytic effect; electroreduction
1 Introduction
Organic halides, a kind of widely used and versatile compounds in different industrial fields, unfortunately are very toxic and anti-degradable due to the existence of halogenated basis [1]. In order to reduce the harm of organic halides to the environment and human, artificial methods are usually adopted to accelerate the degradation of organic halides. Generally, the degradation of organic halides focuses on a reducing reaction called dehalogenation including catalytic hydrogenation, biological technique and electrochemical reduction [2-4]. Among these methods, the electro- chemical reduction stands out due to its high efficiency of dehalogenation and low cost [5]. However, on the other hand, the dehalogenation requires a relatively negative potential, particularly in the case of PhCH2Cl.
Electrode materials play an important role in electrochemical dehalogenation. In the past few decades, Ag has been found to show extraordinary electrocatalytic property for the reduction of organic halides [6]. It was found that Ag-based nanoparticles (NPs) exhibit better electrocatalytic activity than Ag in the subsequent deepening research. The synergistic catalytic effect between Ag and some noble metals [7-10], such as Au, Pd and Ru, can promote the electrochemical reduction process of organic halides. Especially, Ag-Pd nanocatalysts show excellent electrocatalytic activity in the one-electron reductive cleavage of C—X bond by an electrocatalytic synergy during two steps that Pd atom firstly inserts into C—X bond with a possible participation of Ag atom(s) as the donors, followed by a “transmetalation” process that corresponds to a redox reaction [8]. Ni is an important transition metal, and various methods, such as laser-liquid-solid interaction technique, room-temperature radiolysis and chemical synthesis, have been reported to synthesize Ag-Ni NPs [11,12]. Ag-Ni alloy has shown excellent performance in its applications like sensors and catalysis [13,14], due to its excellent synergistic effect between Ni and Ag. YANG et al [15] concluded that Ag-Ni exhibited much higher activity and selectivity for acetylene hydrogenation than Ag. YI et al [14] reported that Ag-Ni showed excellent catalytic activity in hydrazine oxidation. Some studies have also reported the synergistic effect of Ag and Ni on CO oxidation and methanol electrooxidation [16,17]. Nevertheless, to the best of our knowledge, there is no report on the application of Ag-Ni alloy NPs as the catalyst for the electrochemical reduction of organic halides.
In this study, Ag-Ni alloy NPs were synthesized by the co-reduction of AgNO3 and Ni(NO3)2·6H2O in aqueous solution with NaBH4. The catalytic performance of the Ag-Ni NPs was evaluated by the electrochemical reduction of 0.01 mol/L PhCH2Cl in CH3CN +0.05 mol/L (C2H5)4NBF4 solution.
2 Experimental
2.1 Preparation of nanoparticles
All reagents with analytical grade were used without further purification. Deionized water was used throughout this study. The Ag-Ni alloy NPs were prepared as follows: 5.41 mL 0.05 mol/L AgNO3, 1.99 mL 0.05 mol/L Ni(NO3)2·6H2O and 10 mL 5 mg/mL polyvinyl pyrrolidone (PVP) aqueous solutions were added to 70 mL deionized water under continuous magnetic stirring. Subsequently, excess freshly prepared NaBH4 was added into the above suspension dropwise under stirring condition. After reacting at room temperature for 12 h, the product was obtained by centrifugation, washing and drying. The resultant catalyst was noted as 5Ag1Ni (m(Ag):m(Ni)=5:1) according to the mass ratio of Ag to Ni. For comparison, Ag, Ni catalysts and Ag-Ni nanoalloys with other mass ratios were synthesized by the similar procedure.
2.2 Characterization of nanoparticles
The morphology of nanoparticles was investigated by transmission electron microscopy (TEM, JEOL- 3010). The diffraction patterns were recorded using selected-area electron diffraction (SAED). The composition of the samples was characterized by energy- dispersive X-ray spectroscopy (EDS, JEOL-3010) and ultraviolet-visible spectrometer (UV-Vis, TU-1901). The crystalline structure of the as-prepared Ag-Ni NPs was characterized by X-ray diffraction (XRD, Rigaku D/Max Ultima II diffractometer).
2.3 Electrochemical measurement
Cyclic voltammetry (CV) and chronoamperometry (CA) were carried out in a standard three-electrode electrochemical cell using a CHI 760C electrochemical workstation (Shanghai Chenhua Instrument Factory, China) at room temperature. Pt wire and saturated calomel electrode (SCE) were used as the counter and reference electrodes, respectively. The working electrode was fabricated as follows. Firstly, glassy carbon (GC, S=0.071 cm2) electrode was polished with 0.3 μm alumina powder. Then, 1 mg as-prepared nanocatalyst was dispersed ultrasonically in 1 mL H2O, and 10 μL well-dispersed suspension was dropped onto the GC electrode and dried at room temperature, and 5 μL Nafion (0.5%, mass fraction) was placed on the surface of the catalyst modified GC electrode and dried naturally again. Finally, the electrolyte of 0.01 mol/L PhCH2Cl and 0.05 mol/L (C2H5)4NBF4 in CH3CN solution was deaerated by N2 (99.99%) before used.
Electrochemical impedance spectroscopy (EIS) was utilized to study the performance of cathode in a three-electrode electrochemical cell configuration mentioned above. EIS measurements were carried out on a Zennium electrochemical workstation (CIMPS-2, Zahner) at the reduction potential of -1.5 V. The frequency range was from 10 mHz to 100 kHz with AC voltage amplitude of 10 mV. The EIS data were deconvolved with the Thale equivalent circuit software (Zahner). The electrolyte of 0.01 mol/L PhCH2Cl + 0.05 mol/L (C2H5)4NBF4 in CH3CN solution was deaerated by N2 (99.99%) before used.
3 Results and discussion
3.1 Material characterization
Figure 1(a) shows the XRD patterns of Ag and Ag-Ni NPs with different mass ratios. These patterns clearly show that all Ag-Ni NPs exhibit the face-centered cubic (FCC) structure peaks (111), (200), (220) and (311), which resemble the silver FCC structure [11]. The crystallite sizes of 2Ag1Ni, 5Ag1Ni and 10Ag1Ni calculated using Scherrer formula (d=Kλ/(B cos θ)) from the most prominent peak (111) are 12, 15 and 17 nm, respectively, showing that the size increases with decreasing the Ni content. Ni atoms with smaller radius (0.124 nm) compared with that of Ag atoms (0.144 nm) being present in silver lattice is accounted for this. The presence of single FCC structure peak and the decrease of crystallite size with increasing the Ni content are evidences that Ag-Ni alloy NPs are formed by completely dissolving nickel atoms in silver lattice. Figure 1(b) shows the UV-Vis absorption spectra of Ag, Ni and Ag-Ni alloy NPs with different mass ratios. The absorption bands of 2Ag1Ni, 5Ag1Ni and 10Ag1Ni centring at 397, 403 and 410 nm, respectively, are attributed to the presence of Ag atoms in the alloy samples. These values are similar to that of Ag which is at 414 nm, and nickel does not show any prominent peak. The peak intensity of Ag-Ni NPs decreases with increasing the Ni content. Meanwhile, the peak position of Ag-Ni NPs indicates a blue shift with the inclusion of Ni in the materials. Namely, the presence of Ni dampens the Ag plasmon band and causes a blue shift of the peak position, which indicates that the alloying of Ag-Ni occurs.
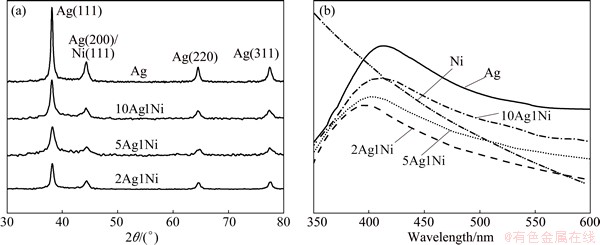
Fig. 1 XRD patterns (a) and UV-Vis spectra (b) of Ni, Ag and Ag-Ni NPs
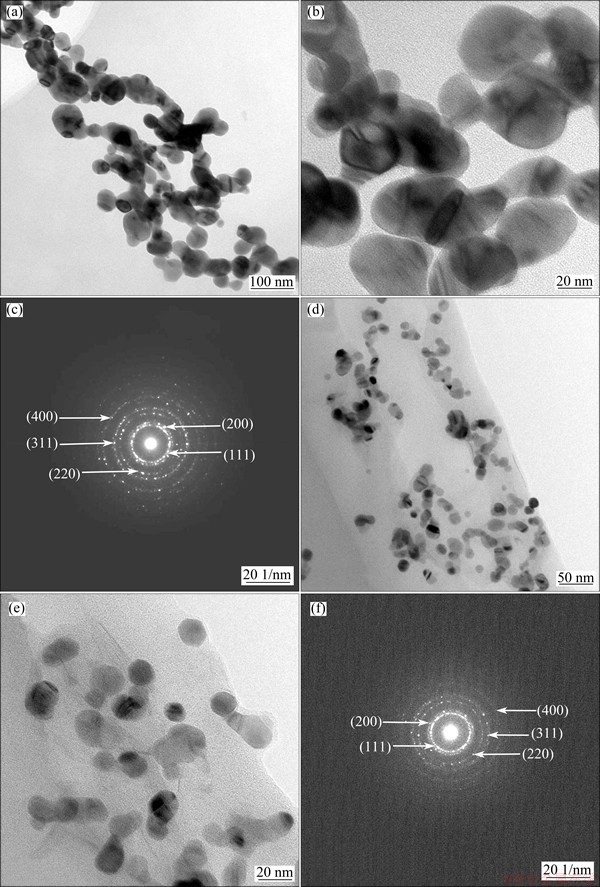
Fig. 2 TEM (a, b, d, e) and SAED (c, f) images of Ag (a-c) and 5Ag1Ni(d-f)
Figures 2(a)-(c) and (d)-(f) show the typical TEM and SAED images of Ag and 5Ag1Ni, respectively. As shown in Figs. 2(b) and (e), the 5Ag1Ni alloy particles are nanostructures with an average particle size of around 15 nm which certainly agrees with the calculation result of XRD, while the size of Ag particles is three to four times that of the 5Ag1Ni particles. According to the EDS analysis, the 5Ag1Ni NPs contain 82.89% Ag and 17.11% Ni (mass fraction), giving the mass ratio of 5:1 of Ag to Ni. The SAED patterns of Ag and 5Ag1Ni which exhibit a polycrystalline structure are given in Figs. 2(c) and (f), respectively. The (111) lattice spacings of Ag and 5Ag1Ni are 0.234 and 0.227 nm, and the (200) spacings are 0.202 and 0.195 nm, respectively. Compared with Ag, the decrease of lattice spacing of 5Ag1Ni shows that there is atomic level alloying of Ag and Ni metal atoms. The results indicate that the 5Ag1Ni alloy NPs were successfully synthesized by co-reduction.
3.2 Electrocatalytic behaviors of catalysts for benzyl chloride reduction
The electrocatalytic activity of the as-prepared Ag-Ni NPs was tested for the reduction of PhCH2Cl in CH3CN+(C2H5)4NBF4 solution. Figure 3(a) shows the CVs of PhCH2Cl on bare GC electrode, 5Ag1Ni, pure Ag and Ni electrodes at 50 mV/s. The Ni electrode shows no reduction peak which is so far considered as a non-catalytic material for PhCH2Cl. Generally, the peak potential is one of the benchmarks of electrocatalytic performance [18]. The peak potential (φp) of PhCH2Cl on GC (-2.25 V) is more negative than those on Ag (-1.75 V) and 5Ag1Ni (-1.55 V). The voltammetric data of Ag-Ni NPs with different mass ratios are presented in Table 1. The potential separation on Ag-Ni NPs, expressed as Δφp (φp(Ag-Ni)-φp(Ag)), with the sequence of 5Ag1Ni (0.20 V) > 10Ag1Ni (0.14 V) > 2Ag1Ni (0.10 V) > 1Ag2Ni (-0.05 V), is much different due to the difference in the Ni content. Compared with the datum of Ag NPs, the φp of Ag-Ni NPs (except 1Ag2Ni) shifts positively and the peak current density (Jp) increases. The above results indicate that the electrochemical reduction activity of benzyl chloride on Ag-based NPs/GC electrode is improved obviously owing to the addition of Ni element to the Ag-based materials, although Ni does not have the electrochemical catalytic activity. This may be because the combination of Ag and Ni has a synergistic catalytic function to the reduction process [7]. Namely, with a suitable mass ratio of Ag to Ni, the Ag-Ni alloy NPs show much better catalytic activity for the electroreduction of PhCH2Cl than the Ag NPs.
Figure 3(b) shows the CAs of Ag and 5Ag1Ni NPs for the electroreduction of PhCH2Cl at -1.5 V. The rapid current decay at the initial period shows the adsorption between the electrode and PhCH2Cl [19]. Obviously, 5Ag1Ni NPs exhibit much higher initial current density (Ji) and terminal current density (Jt) than Ag. The Jt of 5Ag1Ni (-2.43 mA/cm2) drops to about 47% of its Ji (-5.12 mA/cm2), while the Jt of Ag (-1.03 mA/cm2) drops to about 41% of its Ji (-2.49 mA/cm2). Moreover, the current density of 5Ag1N is 2.4 times that of Ag after 1800 s. Therefore, the CAs result indicates that 5Ag1Ni has much higher catalytic activity and better long-term performance for the electrochemical reduction of PhCH2Cl than Ag.
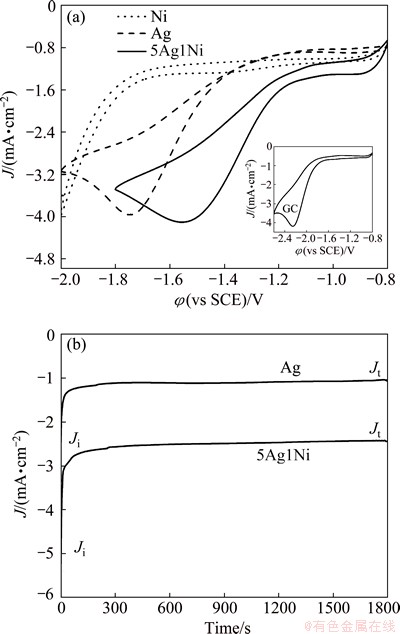
Fig. 3 CVs at 50 mV/s (a) and CAs of Ag and 5Ag1Ni NPs at -1.5 V (b) in CH3CN with PhCH2Cl + (C2H5)4NBF4 solution (Insert in (a) is CV of PhCH2Cl on bare GC at 50 mV/s)
Table 1 Voltammetric data of different cathode materials for reduction of 0.01 mol/L PhCH2Cl in CH3CN + (C2H5)4NBF4 at 50 mV/s
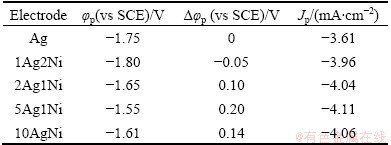
Figure 4 shows the Nyquist plots of the Ag and 5Ag1Ni electrodes at -1.5 V. Both the Ag/GC and 5Ag1Ni/GC electrodes show a similar semicircle domain. It is observed that the electron-transfer resistance (Rct) of Ag is bigger than that of 5Ag1Ni, indicating a relatively fast electron-transfer process of 5Ag1Ni/GC electrode for the reduction of PhCH2Cl compared with that of Ag/GC. The result shows that Ag-Ni NPs show much better catalytic activity for the electroreduction of PhCH2Cl than Ag, which is agreeable with the CAs result.
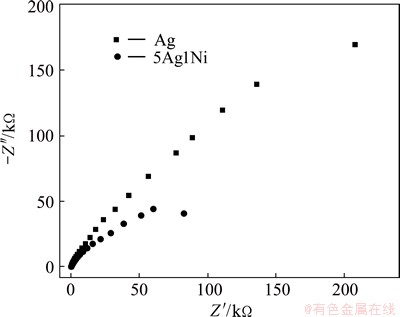
Fig. 4 EIS of Ag/GC and 5Ag1Ni/GC electrodes in PhCH2Cl + (C2H5)4NBF4 + CH3CN solution at -1.5 V
As shown in Figs. 5(a) and (b), the kinetic behaviors of PhCH2Cl on Ag and 5Ag1Ni catalysts were measured by CV at various scan rates (v). It is obvious that with the increase of v, the Jp increases and φp shifts negatively. Besides, the dependence of Jp on v1/2 shown in Figs. 5(c) and (d), presents a linear relationship. It is clear that Ag and 5Ag1Ni show similar catalytic behaviors. Since neither of the two curves pass through (0, 0) in coordinates, it can be concluded that the reaction rate-limiting step of the electrochemical reduction of benzyl chloride on Ag/GC and 5Ag1Ni/GC electrodes is neither the diffusion process nor the electrochemical reaction [20], but may be a more complex interfacial catalytic process.
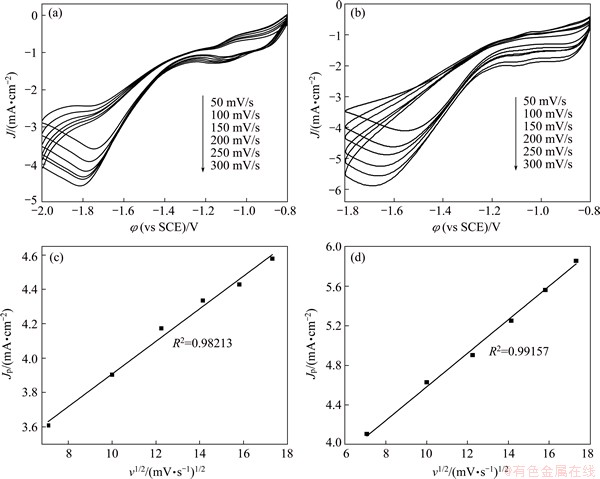
Fig. 5 CVs of Ag (a) and 5Ag1Ni (b) at different scan rates in CH3CN with PhCH2Cl + (C2H5)4NBF4, dependence of Jp on v1/2 of Ag (c) and 5Ag1Ni (d)
Based on the above results and the opinion of POIZOT and SIMONET [8], the reaction model of electrochemical reduction process of PhCH2Cl on Ag/GC and 5Ag1Ni/GC electrodes is proposed as follows: PhCH2+ migrating from bulk solution to the electrode/solution interface is not reduced directly, but undergoes an adsorption process on the electrode surface to form an intermediate of Ag-PhCH2+ which can be reduced. So, the formation of the intermediate may affect the electrochemical reaction rate of PhCH2+. As Ni is 3d84s2, having unoccupied d-orbitals, PhCH2Cl served as a charge donor can easily react with Ni to form a chemical adsorbed chain species such as CH2Ph-Ni-Cl. Then, a fast transmetalation step between CH2Ph-Ni-Cl and Ag0* is followed to form [CH2Ph-Ag+, Cl-]. Subsequently, the electron transfer occurs and a free radical CH2Ph· which is capable of dimerization and/or disproportionation forms (Eqs. (1)-(5)). Besides, the rate of forming [CH2Ph-Ag+, Cl-] on the Ag/GC electrode is slower than that on the 5Ag1Ni/GC electrode. The above catalytic hypothesis corresponds with the electro- chemical result that Ag-Ni NPs show much better catalytic activity for the electroreduction of PhCH2Cl than Ag, and this may be based on a synergistic effect between Ag and Ni [21].
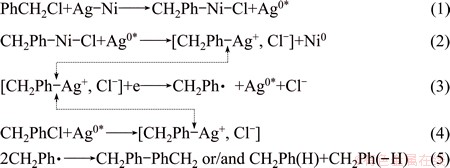
4 Conclusions
1) Ag-Ni alloy NPs with different mass ratios of Ag to Ni were prepared via a simple chemical method.
2) Compared with Ag, Ag-Ni NPs exhibit much better catalytic activity for the benzyl chloride reduction due to the synergistic effect between Ag and Ni. When the mass ratio of Ag to Ni reaches 5:1, the catalytic activity of Ag-Ni NPs is optimal.
3) Ag and 5Ag1Ni show similar catalytic behaviors, and with the increase of scan rates (v), the Jp increases and φp shifts negatively. Besides, the dependence of Jp on v1/2 presents a linear relationship.
References
[1] ALONSO F, BELETSKAYA I P, YUS M. Metal-mediated reductive hydrodehalogenation of organic halides [J]. Chem Rev, 2002, 102(11): 4009-4092.
[2] JANIAK T, OKAL J. Effectiveness and stability of commercial Pd/C catalysts in the hydrodechlorination of meta-substituted chlorobenzenes [J]. Applied Catalysis B: Environmental, 2009, 92(3-4): 384-392.
[3] QUAN Xiang-chun, SHI Han-chang, ZHANG Yong-ming, WANG Jian-long, QIAN Yi. Biodegradation of 2,4-dichlorophenol and phenol in an airlift inner-loop bioreactor immobilized with Achromobacter sp [J]. Separation and Purification Technology, 2004, 34(1-3): 97-103.
[4] YANG Bo, YU Gang, SHUAI Dan-meng. Electrocatalytic hydrodechlorination of 4-chlorobiphenyl in aqueous solution using palladized nickel foam cathode [J]. Chemosphere, 2007, 67(7): 1361-1367.
[5] SUN Zhi-rong, GE Hui, HU Xiang, PENG Yong-zhen. Preparation of foam-nickel composite electrode and its application to 2,4-dichlorophenol dechlorination in aqueous solution [J]. Separation and Purification Technology, 2010, 72(2): 133-139.
[6] ISSE A A, BERZI G, FALCIOLA L, ROSSI M, MUSSINI P R, GENNARO A. Electrocatalysis and electron transfer mechanisms in the reduction of organic halides at Ag [J]. Journal of Applied Electrochemistry, 2009, 39: 2217-2225.
[7] AN C, KUANG Y, FU C, ZENG F, WANG W, ZHOU H. Study on Ag-Pd bimetallic nanoparticles for electrocatalytic reduction of benzyl chloride[J]. Electrochemistry Communications, 2011, 13(12): 1413-1416.
[8] POIZOT P, SIMONET J. Silver–palladium cathode: Selective one-electron scission of alkyl halides: Homo-coupling and cross- coupling subsequent reactions [J]. Electrochimica Acta, 2010, 56(1): 15-36.
[9] SHAN D, ZHOU H, ZHANG C, XIE C, CHEN Z, YE T, KUANG Y. Synthesis of Ag-Ru nanostructures for electroreduction of benzyl chloride[J]. ECS Electrochemistry Letters, 2014, 3(8): H20-H23.
[10] ZHANG G, KUANG Y, LIU J, CUI Y, CHEN J, ZHOU H., Fabrication of Ag/Au bimetallic nanoparticles by UPD-redox replacement: Application in the electrochemical reduction of benzyl chloride[J]. Electrochemistry Communications, 2010, 12(9): 1233-1236.
[11] SANTHI K, THIRUMAL E, KARTHICK S N, KIM H J, NIDHIN M, NARAYANAN V, STEPHEN A. Synthesis, structure stability and magnetic properties of nanocrystalline Ag-Ni alloy [J]. Journal of Nanoparticle Research, 2012, 14(5): 868-872.
[12] ZHANG Z, NENOFF T M, LEUNG K, FERREIRA S R, HUANG J Y, BERRY D T, PROVENCIO P P, ROLAND S. Room-temperature synthesis of Ag-Ni and Pd-Ni alloy nanoparticles [J]. J Phys Chem C, 2010, 114(34): 14309-14318.
[13] CHIRIAC H, URSE M, RUSU F, HISON C, NEAGU M. Ni-Ag thin films as strain-sensitive materials for piezoresisitive sensors [J]. Sensors and Autuators, 1999, 76(1-3): 376-380.
[14] YI Q, LI L, YU W, LIU X, ZHOU Z, NIE H. Novel nanoporous binary Ag-Ni electrocatalysts for hydrazine oxidation [J]. Rare Metals, 2010, 29(1): 26-31.
[15] YANG B, BURCH R, HARDACRE C, HEADDOCK G, HU P. Origin of the increase of activity and selectivity of nickel doped by Au, Ag, and Cu for acetylene hydrogenation[J]. ACS Catalysis, 2012, 2(6): 1027-1032.
[16] MU R, FU Q, XU H, ZHANG H, HUANG Y, JIANG Z, ZHANG S, TAN D, BAO X. Synergetic effect of surface and subsurface Ni species at Pt-Ni bimetallic catalysts for CO oxidation[J]. Journal of the American Chemical Society, 2011, 133(6): 1978-1986.
[17] PARK K W, CHOI J H, KWON B K, LEE S A, SUNG Y E, HA H Y, HONG S A, KIM H , WIECKOWSKI A. Chemical and electronic effects of Ni in Pt/Ni and Pt/Ru/Ni alloy nanoparticles in methanol electrooxidation. [J]. J Phys Chem B, 2002, 106(8): 1869-1877.
[18] MAIYALAGAN T. Synthesis, characterization and electrocatalytic activity of silver nanorods towards the reduction of benzyl chloride [J]. Applied Catalysis A: General, 2008, 340(2): 191-195.
[19] WU M L, CHEN D H, HUANG T C. Preparation of Pd/Pt bimetallic nanoparticles in water/AOT/isooctane microemulsion [J]. Journal of Colloid and Interface Science, 2001, 243(1): 102-108.
[20]
J M. Molecular catalysis of electrochemical reactions. Mechanistic aspects [J]. Chem Rev, 2008, 108(7): 2348-2378.
[21] SHI J. On the synergetic catalytic effect in heterogeneous nanocomposite catalysts [J]. Chem Rev, 2013, 113(3): 2139-2181.
Ag-Ni合金纳米粒子对苄氯还原的电催化
周海晖1,2,李艳玲1,2,黄家琦1,2,方晨旭1,2,单 丹1,2,旷亚非1,2
1. 湖南大学 化学/生物传感与计量学国家重点实验室,长沙 410082;
2. 湖南大学 化学化工学院,长沙 410082
摘 要:对于有机卤代物的电化学还原,银基纳米催化剂显示出优异的催化活性。采用简单的化学还原法制备Ag-Ni纳米颗粒(NPs),并采用X射线衍射、紫外-可见光谱、透射电镜以及能量散射谱等方法对制备的纳米催化剂进行表征。采用循环伏安法、计时电流法以及电化学阻抗谱在有机介质中研究Ag-Ni纳米颗粒对苄氯还原的电催化活性。结果表明:Ni元素的加入可明显减小Ag-Ni纳米颗粒的尺寸,使苄氯的还原峰电位φp正移且增加Ag-Ni纳米颗粒的催化活性。然而,当Ni的含量大于一定值后,Ag-Ni纳米颗粒的催化活性反而降低。同时,对Ag-Ni纳米颗粒的协同催化效应进行探讨。
关键词:Ag-Ni合金纳米颗粒;苄氯;协同催化效应;电化学还原
(Edited by Mu-lan QIN)
Foundation item: Projects (21271069, 51238002, J1210040, J1103312) supported by the National Natural Science Foundation of China; Project (2013GK3015) supported by the Science and Technology Project of Hunan Province, China
Corresponding author: Hai-hui ZHOU; Tel: +86-731-88821603; Fax: +86-731-88713642; E-mail: haihuizh@163.com
DOI: 10.1016/S1003-6326(15)64049-3