
Nickel and nickel-phosphorous matrix composite electrocoatings
Nicolas SPYRELLIS, Evangelia A. PAVLATOU, Styliani SPANOU, Alexandros ZOIKIS-KARATHANASIS
General Chemistry Laboratory, School of Chemical Engineering, National Technical University of Athens,
9 Heroon Polytechniou Str, Zografos Campus, Athens 15780, Greece
Received 18 June 2008; accepted 10 March 2009
Abstract: Nickel and nickel-phosphorous matrix composite coatings reinforced by TiO2, SiC and WC particles were produced under direct and pulse current conditions from an additive-free Watts’ type bath. The influence of the variable electrolysis parameters (type of current, frequency of current pulses and current density) and the reinforcing particles properties (type, size and concentration in the bath) on the surface morphology and the structure of the deposits was examined. It is demonstrated that the embedding of ceramic particles modifies in various ways the nickel electrocrystallisation process. On the other hand, Ni-P amorphous matrix is not affected by the occlusion of the particles. Overall, the imposition of pulse current conditions leads to composite coatings with increased embedded percentage and more homogenous distribution of particles in the matrix than coatings produced under direct current regime.
Key words: nickel electrodeposition; pulse plating; composite coatings; nickel-phosphorus alloy; structure
1 Introduction
Electrodeposited composite coatings consist of a metal or alloy matrix containing hard particles like Al2O3, TiO2, SiC, WC or SiO2 as second phases. For several decades considerable research work has been focused on the impact of the electrodeposition parameters, such as the electrolysis conditions (composition and agitation of the electrolytic bath, the presence of additives, temperature, pH), the current conditions (values of current density and mode of current) and the reinforcement properties (type, size, shape, concentration, conductivity, surface charge) on the structure, morphology and properties of the composite coatings[1].
The major challenges for the codeposition of ceramic particles seem to be the occlusion of a sufficient number of non-agglomerated particles combined with a good dispersion of the particles in the metal matrix. In general, it has been observed that the amount of embedded ceramic particles increases with increasing concentration of suspended particles in the electrolyte[2]. Additionally, the reduction of particle size increases the agglomeration tendency of the particles, due to their enhanced surface energy, while decreases their codeposition content in the metal matrices and the mean grain size of the matrix crystallites[3]. Moreover, recent research has pointed out that the physico-chemical properties of the ceramic particles are crucial to the understanding of the codeposition mechanism of each type of particles[4].
A significant number of reports have shown that the microstructure and properties of pure Ni deposits may be effectively altered under the application of specific pulse current conditions. Pulse electrodeposition (PC) has been found to be an effective means of perturbing the adsorption-desorption phenomena occurring at the electrode/electrolyte interface and hence the electrocrystallization process[5-6].
Regarding Ni-P composite coatings, most of research concerns mainly electroless plating to obtain deposits with enhanced properties[7].
In the present work, the effects of electrolysis parameters on the structure and the morphology of Ni and Ni-P matrix composite coatings reinforced by TiO2, SiC and WC particles were examined.
2 Experimental
Metal and alloy composite coatings were produced from an additive-free modified Watts type bath with addition of the proper reinforcing agent (TiO2, SiC and WC). The electrodeposition experiments were realized on a rotating disc electrode (RDE). An overview of the electrodeposited parameters for the preparation of the coatings is presented in Table 1.
Table 1 Overview of electrodeposition parameters for preparation of Ni and Ni-P matrix composite coatings
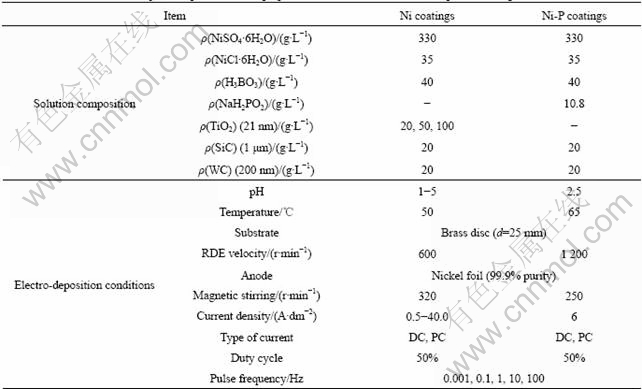
The structure of the deposits was examined using XRD. The grain size of the metal matrix composite materials was calculated using the Sherrer equation. The surface morphology of the coatings was observed by SEM and FE-SEM, while the concentration of the embedded particles was determined by using energy dispersive spectroscopy and fluorescence X-ray spectroscopy.
3 Results and discussion
3.1 Ni matrix composite coatings
The electrocrystallization of Ni is known to be highly inhibited due to the simultaneous hydrogen codeposition. Depending on plating conditions, a predominance of an inhibitor, which selectively promotes one mode of growth (Fig.1(a)), is observed and leads to a deposit exhibiting definite structural properties. A structural investigation of Ni deposits led AMBLARD et al to distinguish a free mode of growth, which is characterized by [100] oriented deposits, from the inhibited modes [110], [210] and [211]. The latter is stabilized by Ni(OH)2, while the two formers are due to atomic and molecular forms of adsorbed hydrogen, respectively[8].
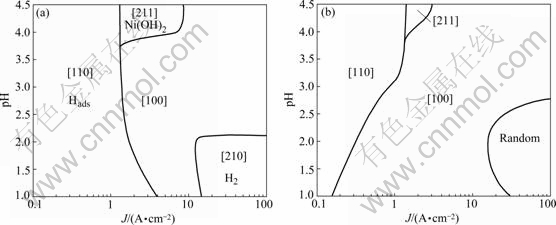
Fig.1 Stability diagrams of crystal preferred orientations as function of pH and applied current density: (a) Pure Ni; (b) Ni-TiO2 composites (TiO2 concentration in bath: 20 g/L)
The embedding of TiO2 nano-particles in the Ni matrix provokes changes in structure of the pure nickel coatings (Fig.1(b)). In detail, the region of [100] oriented deposits is expanded with increasing amounts of titania in the bath, accompanied by a confinement of the [211] and [110] domains. Additionally, a transformation of [210] preferred orientation to random oriented deposits is observed in the presence of TiO2 nano-particles. Overall, all the textural modifications could be attributed to the adsorption of inhibiting species on the surface of TiO2 nanoparticles, while these specific adsorption phenomena could result in a diminution of the activity of the inhibitors, affecting the nickel grains growth and thus imposing the free [100] texture.
As the particle concentration in the bath increases from 0 to 100 g/L, the content of the TiO2 nano-particles in the composite coatings is increased, reaching the maximum incorporation percentage of 9% (volume fraction) achieved at low current densities and low pH values. Moreover, as the TiO2 nano-particles in the deposit increases, the mean grain size of the nickel matrix decreases, resulting in the production of nanostructured nickel matrix in the range of ~20 nm. TiO2 nano-particles in the composite coatings are dispersed uniformly in the matrix, with no serious agglomeration (Fig.2).
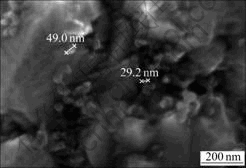
Fig.2 FE-SEM surface micrograph of Ni-nano TiO2 composite electrodeposit under 100 g/L loading in electrolyte prepared under pH 3.5 and current density 0.5 A/dm2
In the case of micron-SiC and nano-SiC composite coatings[6, 9], the embedding of the particles in the Ni matrix modifies the soft-mode [100] texture to a mixed preferred orientation of Ni crystallites through [100] and [211] axis. Several researchers[10] propose that codeposition of SiC particles perturbs the crystal growth of the Ni matrix by inducing an increase in the number of the nucleation sites and, consequently, a reduction in the crystallite size occurs. This effect cannot be explained only by the simple presence of SiC particles, if they are considered physico-chemically inert particles. In fact, the particles are intervened by changing the catholyte composition by adsorption of H+[11], resulting in local alkalization of the cathode/electrolyte interface and, therefore, leading to a [211] mode of crystal growth. Also, this inhibition in synergy with the incorporation effect of SiC particles provokes an increasing number of crystalline dislocations. The overall microstructure change results in the production of Ni/SiC composite deposits with significantly increased hardness in comparison with pure Ni deposits prepared under the similar experimental conditions[9].
The embedding of WC submicron-particles in the metal matrix under DC conditions leads to a porous composite, with almost randomly oriented Ni crystallites, while in the case of the pure Ni deposition, prepared at the same experimental conditions, a non-porous deposit with a high quality [100] preferred orientation is revealed. Although the Ni crystallites of the composites are almost randomly oriented, a careful analysis of the intensities of the diffraction lines shows that there is a slight reinforcement of the [210] orientation. It should be noticed that the [210] texture has not been observed at pH higher than 3 before. Hence, the reinforcement of the [210] texture could be correlated with the enhanced production of molecular hydrogen during the electro- codeposition process, due to the presence of WC particles in the metal matrix. On the contrary, the application of PC conditions and high rotation velocities of the cathode result in the production of compact deposits with high concentration of embedded WC particles with uniform distribution. Taking into consideration of a surface complexation model and the experimental data, a mechanism of nickel electro- crystallization in the presence of WC particles has been proposed[12].
3.2 Ni-P matrix composite coatings
Electrodeposition from an electrolytic Watts’ type bath containing 0.1 mol/L NaH2PO2 leads to the production of Ni-P alloy coatings that are characterized by an amorphous structure (Fig.3). The amorphous structure seems to be preserved also in the case of codeposition of WC or SiC particles, despite the fact that the phosphorous (P) content of the alloy matrix in composite coatings varies. Specifically, for pure NiP coatings it is 12%-14% (mass fraction), while for NiP- WC or NiP-SiC composite coatings it ranges in 13%-15% and 4%-12% (mass fraction), respectively.
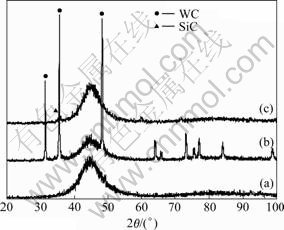
Fig.3 XRD patterns of as-plated NiP (a), NiP-WC (b) and NiP-SiC (c) coatings produced under PC plating (Duty cycle 50%, f=10 Hz)
After thermal treatment at 400 ℃, the NiP matrix is crystallized in the steady phases of Ni and Ni3P (Fig.4). The main diffraction peaks correspond to Ni [111] crystallographic orientation, when P content is lower than 7% (mass fraction), and Ni3P phase for higher P content. Furthermore, for NiP-WC coatings, in which NiP matrix presents the highest observed P content, Ni2P phase is revealed in XRD patterns. It should be mentioned that this phase is referred for the first time for heat treated NiP coatings produced by electrodeposition.
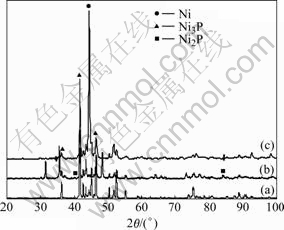
Fig.4 XRD patterns of heat treated NiP (a), NiP-WC (b) and NiP-SiC (c) coatings at 400 ℃ produced under PC plating (Duty cycle 50%, f=10 Hz)
The imposition of PC plating leads to composite coatings with higher percentage of incorporated particles than in the case of DC regime. So, in the case of NiP-WC deposits more than 30% (mass fraction) of codeposited WC particles are achieved, while in the case of NiP-SiC the percentage of embedded SiC particles is 5%-8%. In both cases the percentages of codeposited reinforcing particles obtained from PC plating are more than twice higher than for those achieved by DC plating. Regarding the morphology, the application of PC conditions results in composite coatings with homogeneous distribution of the embedded particles on the surface as well as, across the depth of the deposit (Fig.5).
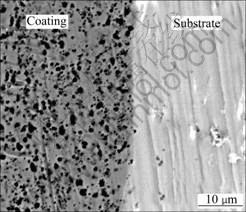
Fig.5 SEM image of cross section of NiP-SiC coating produced under PC plating (Duty cycle 50%, f=1 Hz)
4 Conclusions
It has been proved that the structure and the morphology of the nickel matrix composites depend on the physicochemical properties, the size and the concentration in the bath as well, in the deposit of the codepositing particles. In particular, the [100] crystal orientation of pure Ni is modified by adsorption of H+ on the surface of SiC particles, thus leading to a mixed [100] and [211] mode of crystal growth. The presence of WC particles in the catholyte area results in randomly oriented deposits with enhanced [210] texture due to massive molecular hydrogen production. In the case of TiO2 particles, the [100] is the predominant texture associated with the adsorption of the different inhibitors on the surface of the particles. On the other hand, Ni-P matrix composites present an amorphous structure that is crystallized in Ni, Ni3P and Ni2P phases after heat treatment. Overall, PC application leads to higher embedding percentages and more homogeneous distribution of ceramic particles in the Ni and Ni-P matrices compared with deposits prepared under DC conditions.
References
[1] LOW C T J, WILLS R G A, WALSH F C. Electrodeposition of composite coatings containing nanoparticles in a metal deposit [J]. Surface & Coatings Technology, 2006, 201: 371-383.
[2] GARCIA I, FRANSAER J, CELIS J P. Electrodeposition and sliding wear resistance of nickel composite coatings containing micron and submicron SiC particles [J]. Surface & Coatings Technology, 2001, 148: 171-178.
[3] LAMPKE Τ, WIELAGE B, DIETRICH D, LEOPOLD A. Details of crystalline growth in co-deposited electroplated nickel films with hard (nano)particles [J]. Applied Surface Science, 2006, 253: 2399-2408.
[4] VIDRICH G, CASTAGNET J F, FERKEL H. Dispersion behavior of Al2O3 and SiO2 nanoparticles in nickel sulfamate plating baths of different compositions [J]. Journal of the Electrochemical Society, 2005, 152(5) C294-C297.
[5] KOLLIA C, SPYRELLIS N, AMBLARD J, FROMENT M, MAURIN G. Nickel plating by pulse electrolysis: Textural and microstructural modifications due to adsorption/desorption phenomena [J]. Journal of Applied Electrochemistry, 1990, 20: 1025-1032.
[6] GYFTOU P, PAVLATOU E A., SPYRELLIS N. Effect of pulse electrodeposition parameters on the properties of Ni/nano-SiC composites [J]. Applied Surface Science, 2008, 254: 5910-5916.
[7] APACHITEI I, TICHELAAR F D, DUSZCZYK J, KATGERMAN L. The effect of heat treatment on the structure and the abrasive wear resistance of autocatalytic NiP and NiP-SiC coatings [J]. Surface & Coating Technology, 2006, 200: 263-278.
[8] AMBLARD J, EPELBOIN I, FROMENT M, MAURIN G. Inhibition and electrocrystallization [J]. Journal of Applied Electrochemistry, 1979, 9: 233-242.
[9] PAVLATOU E A, STROUMBOULI M, GYFTOU P, SPYRELLIS N. Hardening effect induced by incorporation of SiC particles in nickel electrodeposits, [J]. Journal of Applied Electrochemistry, 2006, 36: 385-394.
[10] SOCHA R P, NOWAK P, LAAJALEHTO K, VAYRYNEN J. Particle-electrode surface interaction during nickel electrodeposition from suspensions containing SiC and SiO2 particles [J]. Coll Surf A, 2004, 235: 45-55.
[11] LIN C S, HUANG K C. Codeposition and microstructure of nickel-SiC composite coating electrodeposited from sulphamate bath [J]. Journal of Applied Electrochemistry, 2004, 34: 1013-1019.
[12] STROUMBOULI M, GYFTOU P, PAVLATOU E A, SPYRELLIS N. Codeposition of ultrafine WC particles in Ni matrix composite electrocoatings [J]. Surface & Coatings Technology, 2005, 195: 325- 332.
Corresponding author: Evangelia A. PAVLATOU; Tel: +30210-7723110; E-mail: pavlatou@chemeng.ntua.gr
DOI: 10.1016/S1003-6326(08)60353-2
(Edited by YANG Bing)